Abstract
Background
The location and proximity to the spinal cord in spinal osteoid osteoma can increase the likelihood of an incomplete resection. Intraoperative bone scintigraphy (IOBS) can be used to verify location and complete surgical resection.
Objective
To review our experience using IOBS for resection of intraspinal osteoid osteoma.
Methods
IRB approved, retrospective review of IOBS-guided resection over 10 years. Patients underwent injection of 200 uCi/kg (1–20 mCi) 99mTc-MDP 3–4 h prior surgery. Portable single-headed gamma camera equipped with a pinhole collimator (3- or 4-mm aperture) was used. Images were obtained pre-operatively, at the start of the procedure, and intraoperatively. Operative notes were reviewed. Evaluation of recurrence and clinical follow-up was performed.
Results
Twenty IOBS-guided resections were performed in 18 patients (median age 13.5 years, 6–22 years, 12 males). Size ranged 5–16 mm, with 38.9% (7/18) cervical, 22.2% (4/18) thoracic, 22.2% (4/18) lumbar, and 16.7% (3/18) sacral. In all cases, IOBS was able to localize the lesion. After suspected total excision, IOBS altered the surgical plan in 75% of cases (15/20), showing residual activity prompting further resection. Median length of follow-up was 6 months (range 1–156 months) with 90% (18/20) showing complete resection without recurrence. Two patients had osteoid osteoma recurrence at 7 and 10 months following the original resection, requiring re-intervention.
Conclusions
IOBS is a useful tool for real-time localization and assessment of spinal osteoid osteoma resection. In all cases, IOBS was able to localize the lesion and changed surgical planning in 75% of cases. Ninety percent of patients achieved complete resection and remain recurrence free.
Graphical abstract

Similar content being viewed by others
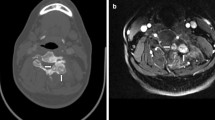
Explore related subjects
Discover the latest articles, news and stories from top researchers in related subjects.Avoid common mistakes on your manuscript.
Introduction
Osteoid osteoma is an osseous tumor accounting for up to 40% of spinal neoplasms and most commonly affects young patients aged 5 to 24 years, with a male predominance [1]. Despite its benign nature, spinal osteoid osteoma often causes intractable pain, spinal deformity (scoliosis), and debilitation that requires surgical resection or ablation. These neoplasms most often involve the cortex of the lower extremities, however, between 10 and 20% of osteoid osteomas occur in the spine, usually involving the posterior elements of the lumbar spine [1,2,3,4]. Bone scintigraphy is very sensitive for osteoid osteoma and can easily confirm the diagnosis, typically showing the “double-density sign” of marked central uptake corresponding to the nidus of osseous matrix rich in active osteoblasts and osteoclasts, as well as surrounding relatively decreased radiotracer uptake corresponding to the lesion’s sclerotic rim [1, 5]. Planar scintigraphy imaging with or without the addition of single-photon emission computerized tomography (SPECT/CT) can be helpful in lesion characterization, with the typical pattern of intense radiotracer uptake effectively confirming the diagnosis of osteoid osteoma. Three-phase bone scintigraphy is rarely necessary, while planar imaging using a pinhole collimator provides additional high-resolution images which are ideal for lesion localization and for guiding management [6,7,8].
Although it has been reported that conservative management with non-steroidal anti-inflammatory drugs (NSAIDs) treatment can result in the spontaneous resolution of symptoms over a period of 2 to 15 years, cases with intractable pain and progressive spinal deformity require procedural interventions [3]. Available surgical treatments include open surgical resection of the lesion or percutaneous minimally invasive procedures such as radiofrequency ablation (RFA) [9, 10]. In spinal osteoid osteoma, the location and proximity of the lesion to the spinal cord and exiting nerve roots can increase the likelihood of an incomplete surgical resection, resulting in multiple interventions to ensure complete resection. Institutionally, we use intraoperative bone scintigraphy (IOBS) to verify location and confirm complete surgical resection of spinal osteoid osteoma.
The aim of this study is to review our experience using IOBS for resection of intraspinal osteoid osteoma during a 10-year period.
Materials and methods
Patient selection
This study was approved by the institutional review board (IRB) of our hospital and was performed in compliance with the Health Insurance Portability and Accountability Act (HIPAA). The requirement for informed consent was waived. A retrospective review of spinal osteoid osteoma surgical resections with IOBS guidance over the course of 10 years was performed (January 2010 through February 2021). Inclusion criteria included pathology proven osteoid osteoma or osteoblastoma, surgical resection guided by IOBS, age younger than 22 years, and available images and operative report. A total of 18 patients from the neurosurgery clinic met the inclusion criteria, two of which required repeat surgical resections due to osteoid osteoma recurrence, for a total of 20 IOBS-guided resections.
Pre-operative and intraoperative bone scintigraphy: patient preparation and imaging technique
Pre-operative baseline bone scintigraphy
All patients underwent baseline bone scan to ensure adequate uptake in the lesion. Each patient was injected intravenously with 99mTechnetium-methylenediphosphonate (99mTc-MDP) (200 uCi/kg (7.4 MBq/kg), minimum 1 mCi (37 MBq), maximum 20 mCi (740 MBq)). For bone scintigraphy performed prior to the day of surgery, the injection was approximately 3–4 h prior to imaging. For pre-operative baseline bone scans performed on the day of surgery, tracer was injected 2 h prior to imaging. Prior to the procedure, patients were instructed to void so that the full bladder does not obscure possible findings within the pelvis. In the nuclear medicine radiology suite, static planar images, with or without SPECT/CT, were obtained to confirm location of the lesion and to assess the intensity of radiotracer uptake.
Baseline bone scan prior to day of surgery (for patients with no prior imaging or indeterminate findings):
-
Whole-body sweep.
-
Static planar imaging, with SPECT or SPECT/CT, as indicated for lesion localization.
-
Pinhole imaging as indicated to confirm localization.
Baseline bone scan day of surgery (for patients with lesions localized by CT or MRI):
-
Static planar image over area of interest to confirm tracer uptake in the lesion.
The static planar images were acquired in a 256 × 256 matrix using a standard dual-headed gamma camera (Symbia, Siemens Healthineers, Malvern, US) equipped with a low-energy ultra-high-resolution collimator. Static images were acquired for 10 min or 300,000 counts. Pre-operative pinhole collimator (2-mm aperture) images were not routinely obtained, unless more precise pre-operative localization and lesion confirmation was needed. Pinhole images, if needed, were acquired for 10 min or 100,000 counts. Whole-body imaging was rarely needed, with most lesions having had prior cross-sectional imaging localization. SPECT or SPECT/CT (Symbia Intevo-Bold, Siemens Healthineers, Malvern, US) imaging, if obtained, utilized a low-energy ultra-high-resolution collimator with images acquired in a 128 × 128 matrix using a full 360 degrees rotation, with 120 projections obtained at 15 s per stop. For SPECT/CT, low-dose attenuation correction CT images were acquired at 100 kVp, reference mAs 20–35, and are used for anatomic localization.
Intraoperative bone scintigraphy
Once the patient was prepped on the operating room table, a portable bone scintigraphy imaging system (Ergo, Digirad, Poway, CA, USA) was brought to the operating room (Fig. 1). A board-certified nuclear medicine physician/radiologist and a nuclear medicine technologist were physically present in the operating room to review the images in real time and to assist in guiding the surgical resection. The operating room team (neurosurgeons and nursing staff) had access to training modules provided by our radiation safety program regarding exposure and best practices during radiation-related procedures. No additional safety precautions were required.
Intraoperative images were acquired for 3 min/acquisition using a pinhole collimator equipped with a 3–4-mm aperture. The patient to aperture distance was typically 2–3 inches.
Prior to incision, the lesion was localized with the Digirad camera (Ergo, Digirad, Poway, CA, USA). The laterality of the lesion was reviewed and compared to pre-operative imaging and verified with the neurosurgeon performing the procedure. To facilitate localization, a small amount (~ 50 μCi) of 99mTc-pertechnetate in the tip of a syringe was used as a marker. This marker is essential for confirming laterality and accurate location of the lesion due to the small field of view pinhole collimator. A small drop of 99mTc-pertechnetate in the tip of a syringe cap is practical and easily prepared, although commercially available gamma probes can also be used.
Once the lesion location had been verified and the incision made, at least 3 intraoperative pinhole collimator images were acquired at 3 min per acquisition. Note the importance of using similar acquisition times to allow for comparison between pre-resection and post-resection images:
-
1)
Pre-incision image (optional): with the patient positioned on the operating table, prior to prepping and draping, a confirmatory image was obtained to affirm lesion detection and to aid in localizing the planned resection site. Acquisition was based on the surgeon’s preference.
-
2)
Post-incision pre-resection image: using the marker to confirm the lesion site, a pre-resection image was obtained following standard skin and soft tissue incision to guide the surgical approach and to establish a baseline reference image for comparison with subsequent post-excision images. Using sterile technique, the collimator/aperture was brought to within 2–3 inches of the operative site.
-
3)
Post-resection image: standard intraoperative microscope guided resection of the osteoid osteoma soft inner core using curettage and drill as necessary was performed with the aim of removing as little bone as needed. The curettage specimens were collected for post-resection specimen imaging. The post-resection bone scan was performed to evaluate for complete resection of the lesion, and was characterized by a photopenic focus at the previous site of uptake associated with the osteoid osteoma lesion. Additional excisions were performed if there was any residual focal tracer uptake, with subsequent post-resection images being obtained until complete resection was confirmed by bone scan.
-
4)
Specimen images: imaging of the resected specimen tissue was performed to confirm radiotracer uptake of the resected specimen (Fig. 2).
Serial intraoperative bone scintigraphy pinhole posterior projection images in an 11-year-old girl with osteoid osteoma in the left C6 facet (arrows). a Pre-operative posterior projection bone scan performed in the nuclear medicine radiology suite and (b) intraoperative pre-resection posterior projection image demonstrates intense focal uptake at the left C6 facet. Four post-resection posterior projection images were performed (c–f). In this case, 4 serial resections were required with complete resection demonstrated by photopenia at the previous site of uptake (circle in post-resection image f). A confirmatory image demonstrates uptake in the resected surgical specimens (g)
Data collection
The electronic medical records were evaluated to obtain relevant clinical data and demographic information including onset and nature of symptoms, treatments, and interventions that were attempted prior to surgical resection, and identification of radiologic studies that were performed for the evaluation of pain as well as their success at identifying the lesion. The intraoperative notes were reviewed to determine the usefulness of IOBS guidance in each case, by identifying need for continued resection despite initial perceived complete resection, as noted by the surgeon in the operative note. The presence or absence of residual activity was recorded. The time to resolution of osteoid osteoma symptoms of pain, the amount of postoperative follow-up time, and presence or absence of recurrence were recorded. Data collection was performed by a second-year radiology resident (AAK) and revised by a musculoskeletal pediatric radiologist with 1 year of post-fellowship experience (MAB) and a nuclear medicine radiologist with 21 years of post-fellowship experience (SDV).
Statistical analysis
All analyses were performed using SPSS statistical software (version 21.0.0; IBM, Armonk, NY, USA). Descriptive statistics were used to summarize the clinical and demographic information, imaging findings, operative findings, and follow-up. Categorical variables were displayed as percentages and counts. Continuous variables were presented as median and ranges. P-values of less than 0.05 were considered statistically significant.
Results
Twenty IOBS-guided spinal osteoid osteoma resections were performed in 18 patients with a median age of 13.5 years (range 6–22 years). Twelve patients (66.7%) were males; 6 patients (33.3%) were female. Two patients returned for repeat osteoid osteoma resection 7 and 10 months after the initial resection due to recurrence. Median time from clinical presentation to initial surgery was 3.0 months (range: 0 to 14 months). All patients presented with persistent pain, 9 (50.0%) with pain at night, 5 (27.8%) with painful scoliosis, and 1 (5.6%) with associated limited range of motion. Before surgical resection, all patients were treated with NSAIDs, 7 (38.9%) with associated physical therapy, 5 (27.8%) with rest, and 1 (5.6%) with bracing due to scoliosis. In all cases, conservative management did not result in resolution of symptoms. One patient had a prior unsuccessful resection at another institution, where IOBS was not used, without resolution of pain. All patients underwent CT evaluation before surgery, with the median time from CT to surgery being 3.0 months (range 0 to 14 months). On CT, the median size of the osteoid osteoma was 8.0 mm (range 5–16 mm). Of the 18 patients, 17 also underwent magnetic resonance imaging (MRI) before surgical resection with a median time from MRI to surgery of 4 months (range: 0–14 months). The most common osteoid osteoma location was the cervical spine in 7 patients (38.9%), followed by the lumbar spine in 4 patients (22.2%), thoracic spine in 4 patients (22.2%), and sacrum in 3 patients (16.7%). Table 1 summarizes the patient demographics, symptoms, and findings at presentation and follow-up.
IOBS was able to localize the lesions in all the cases, such that no further CT, MRI, or x-ray localization was required. Based on the 20 IOBS-guided intraoperative reports, use of intraoperative scintigraphy altered the surgical approach in 15 cases (75%). In each of these cases, although complete surgical excision was expected based on intraoperative microscopy, the post-resection bone scan showed residual activity at the operative site, prompting further resection to completion (Fig. 2). The median number of required resections was 2 (range: 1–9). The median length of the surgical procedure from skin incision to skin closure was 138 min (range: 42–211 min). None of the cases experienced surgical complications. Of the 20 surgical interventions, only 2 cases (10%) required spinal fusion. There were two cases in which mild residual scintigraphic uptake was noted at the end of surgery and was thought to be reactive in nature, related to uptake in surrounding bone. One of those two cases experienced lesion recurrence. All the specimen images demonstrated expected radiotracer uptake that confirmed the presence of nidus tissue.
Median length of follow-up was 6 months (range 1–156 months). Of the 20 IOBS-guided, 18 (90%) had complete resection without recurrence. In one unusual case of osteoid osteoma recurrence (case #7 in Table 1), a 15-year-old male had recurrence of his pain 7 months after initial surgical resection of an osteoid osteoma in the L4 right facet. RFA was performed; however, symptoms persisted. CT showed 2 separate foci of recurrent osteoid osteoma within the right facet near the previous site of surgical resection. Intraoperative pre-resection pinhole images revealed 2 adjacent foci of uptake that correlated with CT and these were resected. However, after what was initially assumed to be a complete surgical resection, an additional site of focal uptake was identified and resection of this third distinct lesion was performed (Fig. 3). This third lesion was occult on the initial pre-surgical CT as well as initial intraoperative bone scans due to its location relative to the two initial target lesions. After this definitive surgical intervention, the patient’s symptoms resolved and he has been pain free for 1 year.
Serial intraoperative bone scintigraphy pinhole images of a 15-year-old boy who had recurrence of pain 7 months after initial surgical resection of an osteoid osteoma in the L4 right facet. a Intraoperative pre-resection pinhole posterior projection images revealed 2 adjacent foci of uptake (arrow and dashed arrow) that correlated with CT. b First post-resection posterior projection image was performed after the resection of one of the lesions with expected residual uptake of the second lesion (dashed arrow). c Second post-resection posterior projection image after surgical resection of both separate foci seen pre-resection showed an additional site of focal uptake (arrowhead), and resection of the third separate lesion was performed. d Third post-resection posterior projection image shows photopenia at the previous site of uptake. The third separate lesion was occult on initial pre-surgical CT as well as initial intraoperative bone scans. e–g Specimen images and intraoperative resection images (h, i) confirmed the multifocal characteristic of the recurrence
Discussion
Intraoperative bone scintigraphy is a useful tool for real-time assessment and guidance of spinal osteoid osteoma resection. This method helps both to localize the lesion and possible satellite lesions, as well as to confirm resection of the osteoid osteoma nidus while attempting to remove the least amount of bone. Our study showed that in 75% of cases, the surgical team had suspected complete resection; however, IOBS demonstrated residual foci of uptake, prompting additional resections. The recurrence rate was only 10%, with both recurrence cases requiring additional IOBS-guided resections. A notable benefit, especially in the pediatric population, is that after administration of a single dose of 99mTc-MDP, multiple exams can be performed throughout the operative procedure without an increase in radiation dose to the patients, in contrast to other methods of intraoperative guidance, such as fluoroscopy or CT. We also present an atypical case of osteoid osteoma with satellite lesions after attempted surgical resection and RFA, where IOBS was vital in the complete resection of the multicentric lesions. Multicentric osteoid osteoma, with more than one nidus, is rare and has been observed in less than 1% of all OO lesions [11].
In general, open surgical resection of spinal osteoid osteoma is performed with fluoroscopic, CT, or cone-CT guidance. Kadhim et al. evaluated the use of intraoperative C-arm (2D navigation in 10 patients) and O-arm (3D navigation in 7 patients) for surgical resection of spinal osteoid osteomas/osteoblastomas [12]. The C-arm failed to identify the osteoid osteoma lesion in 1 case (10%), requiring transport to the CT scanner. In this cohort, there was a 13% rate of recurrence, which is slightly higher than our cohort. In addition, 10 of the 17 (59%) patients reported by Kadhim et al. had extensive spinal resections requiring spinal stabilization with posterior spinal tension band or posterior spinal fusion, as compared to our cohort where only 2 surgical resections (10%) required spinal stabilization procedures [12]. It is unclear why the rates of spinal stabilization procedures differ between these 2 studies; however, it is noted that different surgical guidance was done and the surgical resections were performed by different surgeon teams (neurosurgery in our cases and orthopedic surgery in the prior study) [12].
Radionuclide surgical guidance has been previously described in spinal osteoid osteoma, using IOBS guidance [6] or gamma probe guidance [13]; as well as extra-spinal osteoid osteoma, using gamma probe and mini gamma camera [14, 15]. Taylor et al. described the feasibility of surgical localization of osteoid osteoma with intraoperative scintigraphy in spinal and extra-spinal locations [16]. Compared to the current study, the equipment and technique differ as follows: Taylor et al. used a Siemens LEM gamma scintillation camera (NaI crystal-based scintillation detector) with a 2.5-mm pinhole collimator, compared to a portable gamma camera (Digirad ERGO) (solid-state electronics with a digital detector) and larger pinhole collimator aperture (3–4 mm). Although a direct comparison was not performed, this update is thought to provide increased sensitivity, improved image resolution and shorter image acquisition times. Additionally, in this study, consistent acquisition time (3 min per acquisition) was reinforced in order to accurately compare images pre- and post-resection, while minimizing our acquisition times in response to time pressures in the operating room, compared to the prior study that used 4–5 min per acquisition. This report extends the initial experience with IOBS-guided surgical resections of spinal osteoid osteoma lesions, in which Blaskiewicz et al. demonstrated the feasibility and sensitivity of IOBS in detecting the nidus and providing intraoperative guidance for a complete resection [6]. The recurrence rate (7%) was similar to our cohort without any reported surgical complications. In addition to extending the initial experience with IOBS for spinal osteoid osteoma, it should also be noted that the patients currently being referred to our tertiary center for surgical resection have become increasingly complex, both in terms of lesion size and location. Based on early success with this technique, patients who previously might not have been considered amenable for this type of image-guided minimally invasive approach and who have failed therapy elsewhere have come as a result of this technique being available.
Compared to osteoid osteomas located in the diaphysis of the long bones, there are several important factors that must be considered in choosing the correct treatment and planning surgical resection of spinal osteoid osteoma. These include minimizing the need for spinal stabilization procedures, limiting direct contact with neural elements such as spinal cord and nerve roots, which are often in close proximity to the resection site, and the assurance of a complete resection. In addition, the bone scan is critically important in finding the lesion intraoperatively, which can be quite difficult in the spine based solely on visual inspection. Therefore, the surgical goal is to resect the least amount of bone while performing a complete resection of the pea-shaped nidus [17]. The sclerotic rim that surrounds the pea-shaped soft tissue inner core nidus is not a histological part of the osteoid osteoma lesion and therefore does not require to be resected [6]. In general, there is no need to perform an en bloc surgical margin of resection, compared to malignant or benign aggressive spinal tumors [3, 6]. Because the central intense 99mTc-MDP uptake of osteoid osteoma lesions localizes to the soft tissue nidus [1, 5], IOBS is one of the only tools that provides direct intraoperative confirmation as to the presence of residual central core nidus tissue, thereby confirming complete surgical resection with the least amount of bone resected. Imaging of the specimens is performed together with the surgical site images to improve the confidence of complete resection of the nidus. These images are just another way of making certain that the resected specimen contained the nidus. However, if time does not allow, the specimen images could be omitted as in our study we did not see any case where the specimen images showed contradictory information compared to the surgical site images.
Percutaneous minimally invasive procedures, such as RFA are becoming increasingly more common in management of spinal osteoid osteoma [9, 10]. Most recently, additional non-surgical treatment techniques, such as cryoablation, have been used to treat non-spinal osteoid osteoma [18]; however, only a small number of successful spinal osteoid osteoma cases treated with cryotherapy has been reported [18, 19]. Despite the advantage of a minimally invasive approach in RFA, there is still debate whether surgical resection remains the preferred treatment approach in spinal osteoid osteoma given potential risk of thermal damage to neurological structures in RFA. Multiple studies have reported a lower local recurrence rate in surgical resection, compared to RFA, ranging from 1.7 to 7% after surgical resection [3, 20, 21] and 6.5% to 20% after RFA [3, 22,23,24]. However, in a recent meta-analysis that included 749 patients and compared RFA versus surgical resection for the treatment of spinal osteoid osteoma, Sangiorgio et al. demonstrated that both techniques had similar rate of success (86% in RFA and 89% in surgical resection), rate of recurrences (7% in RFA and 6% in surgical resection), and low rate of complications (4% in RFA and 8% in surgical resection) [9]. Compared to RFA, the main disadvantage of surgical resection relates to the potential need for spinal stabilization and the increased risk of perioperative complications [25]. In our study cohort, only 2 surgical resections (10%) required spinal stabilization procedures and no postoperative complications were identified. With the use of IOBS, the pre-operative surgical marking site is accurate, and the surgical incision is small, allowing an approach that is as minimally invasive as possible. In our pediatric practice, based on the complex nature of patients referred to our center and the success rate of the neurosurgical approach described here, we have not yet explored minimally invasive techniques for the initial treatment of spinal osteoid osteoma, although this will be an important adjunct to our practice in the future.
One potential disadvantage of this technique is the subjective, non-quantitative evaluation of the residual uptake present on the post-resection intraoperative image; for instance, in one of the patients in whom a recurrence occurred, the mild residual scintigraphic uptake that was demonstrated at the end of the initial resection was thought to be reactive in surrounding bone, rather than residual unresected tumor. Reactive sclerosis can contribute to uptake around the margins of the lesion, but in practice, the most intense uptake localizes to the nidus. There is no established quantitative threshold to distinguish reactive uptake from uptake in the nidus. Attenuating soft tissue and bone might limit the direct comparison between the lesion imaged in situ and the resected specimen, particularly when the specimen is composed of fragments resulting from serial curettage rather than en bloc resection. Our approach has been mainly qualitative, although by acquiring images for the same amount of time (3 min per acquisition) rather than based on counts, we rely on comparison of the photopenic resection bed (Figs. 2 and 3), to background skeletal uptake to determine the completeness of resection.
In our institution, the nuclear medicine physician is physically present in the operating room; however, if the nuclear medicine physician cannot be present, images can be sent directly from the portable camera to a processing workstation for review, although this may delay real-time decision-making in the operating room. While remote interpretation of intraoperative imaging is technically feasible, we have found the direct interaction between the surgeon and nuclear medicine physician to be invaluable, particularly in challenging cases where the distinction of reactive bone and residual nidus is difficult to assess. Although the use of IOBS for spinal osteoid osteoma resection necessarily adds time to the procedure and requires availability of additional nuclear medicine staff in the operating room, which may limit the widespread implementation of this approach, we have found this team approach to be helpful in terms of patient safety, providing additional reassurances regarding laterality, localization of the lesion, and increased confidence in determining the complete resection of the lesion.
The study is limited by the intrinsic nature of a retrospective study. Because the assessment of the intraoperative events and decision-making relied on a retrospective analysis of the operative reports, this study may underestimate the impact of intraoperative scintigraphy if the benefits were not specifically mentioned in the reports. Despite these limitations, a systematic use of this method during osteoid osteoma resections can likely improve surgical outcomes and should be considered. The heterogeneous cohort, with different tumor locations and sizes, limits the ability to perform inferential statistics.
Conclusion
In conclusion, IOBS is a useful tool for real-time localization and assessment of osteoid osteoma resection. Our study showed that in all cases it was possible to localize the lesion, and in 75% of cases, the surgical team suspected complete resection based on surgical findings, while intraoperative scintigraphy demonstrated residual neoplasm, prompting further resection. The majority (90%) of patients achieved complete resection and remain recurrence free. In cases where surgical resection of spinal osteoid osteoma is planned, IOBS should be considered.
Data availability
The datasets generated during and/or analysed during the current study are available from the corresponding author on reasonable request.
References
Iyer RS, Chapman T, Chew FS (2012) Pediatric bone imaging: diagnostic imaging of osteoid osteoma. AJR Am J Roentgenol 198:1039–1052
Mallepally AR, Mahajan R, Pacha S et al (2020) Spinal osteoid osteoma: surgical resection and review of literature. Surg Neurol Int 11:308
Pipola V, Tedesco G, Spinnato P et al (2021) Surgery versus radiofrequency ablation in the management of spinal osteoid osteomas: a spine oncology referral center comparison analysis of 138 cases. World Neurosurg 145:e298–e304
Parmeggiani A, Martella C, Ceccarelli L et al (2021) Osteoid osteoma: which is the best mininvasive treatment option? Eur J Orthop Surg Traumatol 31:1611–1624
Helms CA, Hattner RS, Vogler JB 3rd (1984) Osteoid osteoma: radionuclide diagnosis. Radiology 151:779–784
Blaskiewicz DJ, Sure DR, Hedequist DJ et al (2009) Osteoid osteomas: intraoperative bone scan-assisted resection. Clinical article. J Neurosurg Pediatr 4:237–244
Connolly LP, Treves ST, Connolly SA et al (1998) Pediatric skeletal scintigraphy: applications of pinhole magnification. Radiographics 18:341–351
Vigorita VJ, Ghelman B (1983) Localization of osteoid osteomas—use of radionuclide scanning and autoimaging in identifying the Nidus. Am J Clin Pathol 79:223–225
Sangiorgio A, Oldrini LM, Candrian C et al (2023) Radiofrequency ablation is as safe and effective as surgical excision for spinal osteoid osteoma: a systematic review and meta-analysis. Eur Spine J 32:210–220
Sagoo NS, Haider AS, Chen AL et al (2022) Radiofrequency ablation for spinal osteoid osteoma: a systematic review of safety and treatment outcomes. Surg Oncol 41:101747
Ciftdemir M, Tuncel SA, Usta U (2015) Atypical osteoid osteomas. Eur J Orthop Surg Traumatol 25:17–27
Kadhim M, Binitie O, O’Toole P et al (2017) Surgical resection of osteoid osteoma and osteoblastoma of the spine. J Pediatr Orthop B 26:362–369
Hempfing A, Hoffend J, Bitsch RG, Bernd L (2007) The indication for gamma probe-guided surgery of spinal osteoid osteomas. Eur Spine J 16:1668–1672
Infante JR, Lorente R, Rayo JI et al (2015) The use of radioguided surgery in the resection of osteoid osteoma. Rev Esp Med Nucl Imagen Mol 34:225–229
Etchebehere M, Etchebehere ECSC, Reganin LA et al (2004) Intraoperative localization of an osteoid-osteoma using a gamma probe. Int Orthop 28:379–383
Taylor GA, Shea N, O’Brien T et al (1986) Osteoid osteoma: localization by intraoperative magnification scintigraphy. Pediatr Radiol 16:313–316
Aydinli U, Ozturk C, Ersozlu S, Filiz G (2003) Results of surgical treatment of osteoid osteoma of the spine. Acta Orthop Belg 69:350–354
Lindquester WS, Crowley J, Hawkins CM (2020) Percutaneous thermal ablation for treatment of osteoid osteoma: a systematic review and analysis. Skeletal Radiol 49:1403–1411
Whitmore MJ, Hawkins CM, Prologo JD et al (2016) Cryoablation of osteoid osteoma in the pediatric and adolescent population. J Vasc Interv Radiol 27:232–7; quiz 238
Quraishi NA, Boriani S, Sabou S et al (2017) A multicenter cohort study of spinal osteoid osteomas: results of surgical treatment and analysis of local recurrence. Spine J 17:401–408
Gasbarrini A, Cappuccio M, Bandiera S et al (2011) Osteoid osteoma of the mobile spine: surgical outcomes in 81 patients. Spine 36:2089–2093
Yu X, Wang B, Yang S et al (2019) Percutaneous radiofrequency ablation versus open surgical resection for spinal osteoid osteoma. Spine J 19:509–515
Vanderschueren GM, Obermann WR, Dijkstra SPD et al (2009) Radiofrequency ablation of spinal osteoid osteoma: clinical outcome. Spine 34:901–904
Albisinni U, Facchini G, Spinnato P et al (2017) Spinal osteoid osteoma: efficacy and safety of radiofrequency ablation. Skeletal Radiol 46:1087–1094
Burn SC, Ansorge O, Zeller R, Drake JM (2009) Management of osteoblastoma and osteoid osteoma of the spine in childhood. J Neurosurg Pediatr 4:434–438
Author information
Authors and Affiliations
Contributions
M. A. B. and S. D. V. conceived, supervised data collection, and supported the study. A. A. K collated the data, performed the statistical analysis, and drafted the initial manuscript and multimedia. V. C. K, N. S. K., and L. A. D. provided technical support and interpreted the images. K. P. F. and M. R. P. performed the surgeries and provided clinical support. All authors reviewed and approved the final manuscript.
Corresponding author
Ethics declarations
Ethics approval
This study was approved by the institutional review board (IRB) at our hospital and was performed in compliance with the Health Insurance Portability and Accountability Act (HIPAA).
Conflicts of interest
None
Additional information
Publisher's Note
Springer Nature remains neutral with regard to jurisdictional claims in published maps and institutional affiliations.
Rights and permissions
Springer Nature or its licensor (e.g. a society or other partner) holds exclusive rights to this article under a publishing agreement with the author(s) or other rightsholder(s); author self-archiving of the accepted manuscript version of this article is solely governed by the terms of such publishing agreement and applicable law.
About this article
Cite this article
Bedoya, M.A., Krokhmal, A.A., Kourmouzi, V.C. et al. Use of intraoperative bone scintigraphy for resection of spinal osteoid osteoma. Pediatr Radiol 53, 2424–2433 (2023). https://doi.org/10.1007/s00247-023-05773-9
Received:
Revised:
Accepted:
Published:
Issue Date:
DOI: https://doi.org/10.1007/s00247-023-05773-9