Abstract
Purpose
Seizures are often followed by a period of transient neurological dysfunction and postictal alterations in cerebral blood flow may underlie these symptoms. Recent animal studies have shown reduced local cerebral blood flow at the seizure onset zone (SOZ) lasting approximately 1 h following seizures. Using arterial spin labelling (ASL) MRI, we observed postictal hypoperfusion at the SOZ in 75% of patients. The clinical implementation of ASL as a tool to identify the SOZ is hampered by the limited availability of MRI on short notice. Computed tomography perfusion (CTP) also measures blood flow and may circumvent the logistical limitations of MRI. Thus, we aimed to measure the extent of postictal hypoperfusion using CTP.
Methods
Fourteen adult patients with refractory focal epilepsy admitted for presurgical evaluation were prospectively recruited and underwent CTP scanning within 80 min of a habitual seizure. Patients also underwent a baseline scan after they were seizure-free for > 24 h. The acquired scans were qualitatively assessed by two reviewers by visual inspection and quantitatively assessed through a subtraction pipeline to identify areas of significant postictal hypoperfusion.
Results
Postictal blood flow reductions of > 15 ml/100 g−1/min−1 were seen in 12/13 patients using the quantitative method of analysis. In 10/12 patients, the location of the hypoperfusion was partially or fully concordant with the presumed SOZ. In all patients, additional areas of scattered hypoperfusion were seen in areas corresponding to seizure spread.
Conclusion
CTP can reliably measure postictal hypoperfusion which is maximal at the presumed SOZ.
Similar content being viewed by others
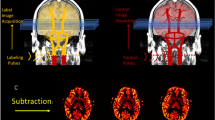
Explore related subjects
Discover the latest articles, news and stories from top researchers in related subjects.Avoid common mistakes on your manuscript.
Introduction
Epilepsy is one of the most common and serious neurological conditions. Approximately 30% of patients with epilepsy do not have their seizures controlled using medications [1]. In these cases, one of the most effective treatment options, in appropriately selected patients, is removal of the seizure onset zone (SOZ). The SOZ is conventionally localized by electroencephalography (EEG) recordings of seizures and subtraction of single photo emission computed tomography (SPECT) measures of blood flow during the ictal and interictal phase. These results are then integrated with other investigations including structural MRI, positron emission tomography, and neuropsychology, among other investigations [2]. However, EEG has limited localization accuracy and ictal SPECT is labor-intensive and costly. The overall success rate of resective epilepsy surgery is around 60% according to systematic reviews and controlled studies [3].
Vascular changes have been increasingly investigated for their potential to localize the SOZ. Transient postictal vascular changes localizing to the SOZ have been observed in animal [4] and human studies [5]. Through invasive implantation of CBF sensors in humans, progressive hypoperfusion of the epileptic focus correlated with increased epileptogenicity, further supporting the link between ischemia and epileptic seizures [5]. A recent animal study systematically demonstrated hypoperfusion and severe hypoxia (oxygen partial pressure < 10 mmHg) in the hippocampus lasting approximately 1 h after hippocampal kindled seizures in rodents [6].
Arterial spin labeling (ASL) MRI has also been used to non-invasively detect interictal and postictal alterations in cerebral blood flow (CBF) in humans [7,8,9,10,11]. CBF changes were found in regions concordant with the presumed SOZ based on EEG, MRI, SPECT, and positron emission tomography (PET). Similarly, computed tomography perfusion (CTP) studies have shown postictal focal hypoperfusion and interictal hyperperfusion in patients in status epilepticus lateralizing to the SOZ [12,13,14]. Although the aforementioned studies largely revealed interictal hypoperfusion in regions corresponding to the presumed SOZ, the results were variable, including contralateral focal and hemispheric hypoperfusion, hyperperfusion, or no significant perfusion changes [9, 11,12,13,14].
Recently, we measured postictal CBF in 21 patients with drug-resistant focal epilepsy using ASL within 90 min of an electrographically confirmed seizure [15]. Localized postictal CBF reductions greater than 30% of baseline CBF values were seen in 71% of patients, localizing to the presumed SOZ in 80% of cases.
While these ASL data support the possibility of using postictal ASL CBF measurements as a potentially useful tool to localize the SOZ, clinical implementation of ASL is hampered by difficulty in obtaining MR scans within 1 h of seizure termination. CTP imaging has the necessary spatial resolution to detect postictal hypoperfusion and is readily accessible on short notice in most hospitals. Therefore, the aim of the present study was to use CTP to measure the extent of the postictal hypoperfusion in patients with refractory focal epilepsy.
Methods
Participants and study protocol
The study was approved by the Conjoint Health Research Ethics Board of the University of Calgary and all patients provided informed consent. Fourteen consecutive adults with drug-resistant focal epilepsy admitted to the Seizure Monitoring Unit at the Foothills Medical Center for continuous scalp video-electroencephalography (VEEG) monitoring between September 2016 and December 2017 were prospectively enrolled in the study. Exclusion criteria for the study were multiple seizure onset zones and contraindications to CT (e.g., pregnancy, allergy to IV contrast material) or MR imaging (e.g., claustrophobia, ferromagnetic intracranial devices).
When a habitual seizure was observed, a physician immediately reviewed the VEEG monitoring data. Once a seizure was confirmed electrographically, patients underwent CTP imaging within 80 min of seizure termination. After collecting the postictal scan and while still in hospital, patients underwent a baseline interictal CTP scan following a seizure-free period of > 24 h. EEG electrodes were removed for both scans.
Clinical data collection
All patients underwent continuous scalp VEEG using 10–20 electrode placement to capture habitual seizures. Demographic data including age, sex, duration of epilepsy, seizure frequency, and seizure types were recorded. As part of presurgical investigations, all patients underwent a standard structural MRI protocol at our center that includes axial 3D MP-RAGE or FSPGR, axial FSE T2, axial FLAIR, coronal FSE T2 through temporal lobes, coronal FLAIR through temporal lobes, and axial SWI. Ictal and interictal SPECT and PET investigations were obtained as clinically indicated.
Definition of the seizure onset zone
The SOZ was determined by clinical history, interictal and scalp VEEG, structural MRI, PET (if performed), interictal and ictal SPECT (if performed), and expert consensus from our weekly multidisciplinary epilepsy surgery rounds. We referred to this area as the “presumed SOZ,” as the true epileptogenic zone can only be identified postoperatively and not all cases underwent surgery.
CT perfusion data collection and functional maps
CTP images were collected with 120 mm coverage, with 5 × 5-mm-thick slices acquired at 80 kVp and 125 mA, and 1 s rotation time (GE Healthcare Revolution, Waukesha, WI, USA). After the scan, a retroactive reconstitution for slice thickness of 1.25 mm at 1.25-mm intervals was performed, allowing the collection of both 5- and 1.25-mm-thick slices. The CTP protocol consisted of 45 ml of an iodinated CT contrast agent (Omnipaque, GE Healthcare) that was power injected at 4 ml/s followed by a 40-ml saline chase. Scanning began after a delay of 5 s from contrast injection, sampling every 2 s for 24 passes, then every 15 s for 8 passes, with a total scan time of 184 s. Foam padding was placed around patients’ heads to minimize motion during scanning. Each study was analyzed using commercially available delay-insensitive deconvolution software (CT Perfusion 4D, GE Healthcare).
For each study, the arterial input function was manually selected from the internal carotid artery using a 2 × 2 voxel (in-slice) region-of-interest (ROI). Maps of absolute CBF (ml/100 g−1/min−1) were calculated by deconvolving the arterial input function from brain time density curves using a delay-insensitive algorithm (CT Perfusion 4D, GE Healthcare). In-plane patient motion was corrected using automated software. In cases of extreme motion, time points were manually removed as needed.
Visual reviewers
CTP data were reviewed using two different approaches. First, CT perfusion maps were reviewed qualitatively. Second, a more objective, quantitative subtraction analysis was performed (described later) which was used in our previously published study [15]. For the qualitative review, images were anonymized and displayed using OsiriX medical image viewer (https://www.osirix-viewer.com). Two reviewers (T Lee, C d’Esterre) with extensive experience in viewing CTP quantitative maps were recruited to visually assess CTP absolute CBF maps for perfusion abnormalities. Reviewers were informed that all patients had suspected focal epilepsy but were otherwise blind to other clinical information.
Visual evaluation of hypoperfusion detected by CTP
In the first approach, reviewers were presented with a series of 27 CTP CBF maps (14 postictal, 13 baseline) from 14 patients alongside an anatomical CTP scan (CT average map generated by averaging all the images of the same brain slice acquired in a CT perfusion study) for additional anatomical delineation and differentiation between areas of hypoperfusion and white matter in the CBF maps. Reviewers were blinded to whether a scan was postictal or baseline and assessed each case independently in a randomized order. Areas of hypoperfusion were visually assessed for perfusion symmetry.
In the second approach, reviewers were unblinded to the order of the maps and viewed the baseline and postictal maps side-by-side for each patient for 13 image sets. In addition to intra-scan perfusion symmetry, symmetry between the postictal and baseline perfusion patterns was also compared. Scales were manually adjusted to visually match intensities between maps, or both maps were assessed on the same intensity scale to visualize any global perfusion differences.
In both approaches, reviewers identified areas of hypoperfusion based on hemisphere (left, right, no localization) and lobe (frontal, temporal, parietal, occipital, no localization) and provided a confidence score (1 [least confident] to 5 [most confident]) for each assessment.
Both approaches were completed twice for each reviewer and in independent individual sessions; the first round assessed images of 5 mm thickness, and the second round assessed images of 1.25 mm thickness. Each round was done approximately 2–3 months apart. CT image thicknesses of 1.25 and 5 mm were assessed to determine which image thickness to use for subsequent quantitative subtraction analysis.
Statistical analysis of visual evaluation data
The location of the presumed SOZ based on the clinical consensus of the Calgary Comprehensive Epilepsy Program comprised of ten epileptologists was used as a gold standard to compare the reviewers’ assessments. Positive predictive value (PPV) and sensitivity for each reviewer in each review condition (i.e., postictal, postictal versus baseline) and slice thickness were calculated. Negative predictive value and specificity were not calculated due to the limitation that the clinical consensus opinion provided an unclear SOZ location for only 4/14 patients (patients 8, 10, 13, and 14).
Cohen’s kappa (κ) was calculated to determine interrater reliability as it normalizes the observed agreement between reviewers due to chance and produces a coefficient between 0 (indicating chance agreement) and 1 (indicating perfect interrater agreement). For hemispheric assessments (left, right, or no localization), a kappa coefficient was calculated to evaluate interrater agreement between the baseline, postictal, and baseline versus postictal image sets. Assessments were evaluated as either complete agreement or complete disagreement.
If reviewers agreed on the hemispheric evaluation, then an additional kappa coefficient was calculated to evaluate agreement on lobar location. As summarized in Table 1, a weighted kappa allowed for additional assignments of different weightings of disagreement and was used when assessing interrater agreement in lobar assessments (frontal, temporal, parietal, occipital, and no localization).
Complete disagreements were assigned a weighting of 1 and was applied in cases of frontal versus occipital and for no localization versus any localization. Partial disagreements were assigned a weighting of 0.5 in all other cases between lobar assessments (Table 1).
The interpretation of the kappa value was based on the guidelines from McHugh [16], as summarized in Table 2.
CTP subtraction analysis for SOZ localization
For the subtraction analysis, 1.25-mm-thick slices were used except for patients 4 and 6 in whom 5-mm-thick slices were used because projection data were lost. Postictal CT average maps were registered to each patient’s baseline CT average maps using an affine transformation (12 degrees of freedom) from FSL FLIRT toolbox (http://www.fmrib.ox.ac.uk/fsl/). Because the anatomical CT images and the CBF CT images share a collective space, the same transformations may be applied to the CBF data, thereby allowing the CBF images to inherit the same accuracy as the anatomical registration. After spatial normalization, the intensities of the postictal CBF maps were normalized to the baseline CBF maps based on global mean CBF value and standard deviation. This was done to account for potential global changes between the two scanning time points.
The global mean was calculated for baseline (M1) and postictal (M2) CBF maps. The difference in means (∆M) was calculated (∆M = M1 − M2) and added to the postictal data in a voxel-wise manner (\( \overset{\sim }{\mathrm{Post}} \)). Standard deviation was calculated for baseline (σ1) and \( \overset{\sim }{\mathrm{Post}} \) (σ2). A ratio (α) of the standard deviation was calculated (α = \( \frac{\sigma_1}{\sigma_2} \)). Finally, the normalized postictal scan was produced as follows:
After intensity normalization, the CTP quantification maps were smoothed by a 5-mm full-width-half-maximum Gaussian kernel to improve signal to noise and reduce small-scale registration errors. Global thresholds were applied to baseline and postictal CBF maps where voxels with CBF values below 0 CBF units were brought up to 0, and voxels with CBF values above 100 CBF units were brought down to 100 to reduce artefactual hypo- and hyperperfusion resulting from vessel artifacts. Using these co-registered CBF maps, a subtraction CBF map (baseline minus postictal) was generated for each patient. Additional cluster filtering (at 400 voxels in 3D) was applied to reduce noise from small registration errors and remaining vessel artifacts. Note that reported changes in CBF values are after performing intensity normalization and may be an underestimation of the absolute CBF difference. The resulting subtraction map was superimposed onto the patient’s CT anatomical average map to identify the anatomical brain areas with postictal CBF reductions of at least 15 CBF units relative to their own baseline. A threshold of 15 CBF units was used based on animal studies showing a 30% reduction of CBF [6]. The reverse subtraction analysis (postictal minus baseline), looking for hyperperfusion, was not performed as our previous animal and human ASL studies showed that hyperperfusion did not play a significant role at the SOZ during the postictal period [6].
Concordance of CTP hypoperfusion and presumed seizure onset zone
The subtraction CTP (sCTP) maps were examined by one reviewer (PF) to determine the anatomical region of maximal hypoperfusion. Although the reviewer was not blinded to the clinical information, this was an objective measure as the location of the maximal hypoperfusion was identified on the quantitative sCTP maps. The location of the maximal hypoperfusion was defined based on hemisphere and lobar regions, which were subdivided into discrete regions as follows:
-
a.
Frontal lobe: divided into orbitofrontal, mesial frontal, and lateral frontal
-
b.
Temporal lobe: divided into equal halves; anterior and posterior segment
-
c.
Parietal lobe: no subdivisions were made
-
d.
Occipital lobe: no subdivisions were made
-
e.
Insula
Hypoperfusion seen on sCTP was classified on a three-tier basis:
-
1.
Unilateral versus bilateral findings
-
2.
Distribution of the findings: focal (small area within a lobe, ~ 3 cm3), lobar (involving > 3 cm3 of a lobe), regional (involving two adjacent lobes), hemispheric (involving three or more adjacent lobes or two non-adjacent lobes in a single hemisphere), multifocal (involving two or more lobes in both hemispheres), and no change (no hypoperfusion > 15 CBF units seen)
-
3.
If applicable, subcategorization of areas of focal prominence within widespread regions of hypoperfusion was performed. Specifically, if among regional, hemispheric, and multifocal areas of hypoperfusion, there were areas of hypoperfusion that were especially prominent because of their size or degree of hypoperfusion; these changes were subcategorized further as “with focal prominence”
A confidence score from 1 to 5 (1, not confident; 5, very confident) was also used to rate the confidence that the reviewer had that the observed areas of hypoperfusion were genuine as opposed to artifact.
An epileptologist (P Federico) and epilepsy fellow (J Peedicail) reviewed all clinical data for each patient and reviewed the expert consensus report from our weekly multidisciplinary epilepsy surgery rounds to confirm the location of the presumed SOZ. To assess concordance, the areas showing postictal hypoperfusion on sCTP maps were compared to the location of the presumed SOZ. Only the most prominent area of hypoperfusion was considered. Concordance was classified as one of three groups:
-
1.
Fully concordant: CTP hypoperfusion was observed in the same lobe as the presumed SOZ.
-
2.
Partially concordant: CTP hypoperfusion was observed in a different lobe in the same hemisphere as the presumed SOZ.
-
3.
Discordant: CTP hypoperfusion was observed in the hemisphere contralateral to the presumed SOZ.
The PPV and sensitivity were additionally calculated by comparing sCTP localization results to the presumed seizure onset zone based on clinical consensus (Table 3).
Areas of seizure spread and concordance with EEG-defined areas of seizure propagation
An epileptologist (PF) and epilepsy fellow (JP) reviewed the VEEG of the captured seizure for each patient and identified areas of seizure propagation based on EEG. Areas of hypoperfusion in the sCTP maps seen outside to the area of maximal hypoperfusion were further assessed as potential areas of seizure spread. Areas of hypoperfusion possibly reflecting seizure spread was classified as concordant with EEG spread if the clusters were in the same hemisphere and lobe(s) or discordant with EEG spread if they were in different lobe(s) in the same hemisphere or on the contralateral side.
Region of interest analysis of maximal postictal hypoperfusion
The cluster(s) with the maximal hypoperfusion in the presumed SOZ in the subtraction CTP maps was selected and isolated as a 3D ROI. Cluster maps were viewed with a minimum threshold of 15 CBF units. Adjacent voxels above 15 CBF units were considered to be in the same cluster. No ROIs were generated in the patient that had no significant postictal hypoperfusion based on sCTP analysis (patient 11). In patients where multiple isolated clusters were seen within the presumed SOZ, the two largest clusters were considered for ROI analysis (patients 5 and 14). The average CBF value of the cluster, representing the average difference between the baseline and postictal scans, was calculated.
Potential confounders
Several cofounders were considered including late CTP data acquisition (> 65 min postictal), postictal CTP scanning following focal aware seizures, epilepsy etiology, seizure duration, and duration of epilepsy.
Results
Patient selection and demographics
Fourteen adult patients were enrolled in the study. One patient did not undergo a baseline CTP scan. Additionally, two patients had their baseline scans collected using our hospital’s clinical stroke protocol (5 mm slice thickness) instead of the study protocol (1.25 mm slice thickness); these two patients were still analyzed and included in the study cohort. Thus, 13 complete sets (postictal and baseline) and one extra postictal scan were collected leading to a total of 14 postictal datasets and 13 baseline datasets being visually evaluated. Similarly, 13 patients were fully analyzed using the quantitative subtraction pipeline. No adverse events were reported.
Patient characteristics
Patient demographic data, clinical information, and investigations are summarized in Table 4. The mean age of the patients at the time of the study was 36.6 years (range 22–60 years). Nine patients (60%) were female. The mean duration of epilepsy was 23.7 years (range 6–47 years). Patients had seizures ranging from multiple per day to monthly. Patients 3 and 9 had previous surgical resections (left temporal lobectomy, and right anterior insular resection). MRI was non-lesional in four patients, ictal SPECT was captured for 11 patients (seven of which were on the same event as the postictal CTP study), and PET was obtained for ten patients (Table 4).
Localization of the presumed seizure onset zone
The presumed SOZ was identified by the Calgary Epilepsy Program in all 14 patients using all available information. The SOZ was clearly identified in ten patients and it was less clear in four (patients 8, 10, 12, and 14), who were ultimately classified. Overall, the SOZ was found to be temporal in seven patients, frontal in three, temporofrontal in two, hemispheric in one, and bilateral in one (Table 4).
Recorded seizures
Table 5 summarizes the details of the seizures that were used for the postictal CTP measurements. Eight patients experienced a focal seizure with impaired awareness, four had seizures evolving to bilateral convulsions, and two had a focal aware seizure. The seizures lasted an average of 102 s (range 29–208 s). Postictal CTP scans obtained an average of 52.4 min following seizure termination (range 35–73 min).
Localization of postictal hypoperfusion: visual assessment
Table 6 summarizes the visual evaluations of the two reviewers. Reviewers consistently detected more localized hypoperfusion with higher concordance to pre-clinical investigations when provided with two scans (baseline and postictal scan side-by-side) versus a single scan (baseline or postictal) in both slice thickness evaluations.
In 1.25- and 5-mm-thick slices on side-by-side comparisons, global postictal hypoperfusion was detected by both reviewers in 4/13 patients and 5/13, respectively. One patient was globally hypoperfused in the postictal period relative to the baseline (Fig. 1a, bottom right images) and had localized maximal hypoperfusion in a similar region to where hyperperfusion was seen on ictal SPECT of the same seizure. The remaining patients had similar global CBF ranges postictally and interictally and also displayed localized hypoperfusion (Fig. 1b). Patient 11 did not have any EEG change with her seizure and visual review of the CTP data was non-localizing in all assessments between both reviewers.
Examples of localizing visually detected postictal hypoperfusion. a An example of global hypoperfusion relative to baseline, with more pronounced focal hypoperfusion in the right mid-posterior temporal region (5 mm). Ictal SPECT of the same seizure showed right temporal hyperperfusion (arrow). b An example of focal postictal hypoperfusion with no global CBF differences (1.25 mm). Ictal SPECT of the same seizure showed right temporal hyperperfusion (arrow)
Table 3 summarizes the statistical sensitivity, positive predictive value, and kappa coefficient for each reviewer in each viewing condition. Kappa coefficients of interrater reliability were on average highest in the 1.25-mm-thick slices compared to 5-mm-thick slices. For both thicknesses, interrater agreement was on average highest in the side-by-side comparison and lowest in viewing single baseline scans. Localization, PPV, and sensitivity were highest in side-by-side comparisons and lowest in independent baseline scans. Indeed, visually reviewing one scan was minimally localizing, particularly for the interictal scans.
Patient with baseline hypoperfusion (patient 14)
Reviewers 1 and 2 independently assessed patient 14 as showing localized baseline hypoperfusion in the 5- and 1.25-mm postictal versus baseline comparison. The postictal scan when viewed alone was non-localizing and globally uniform. Baseline hypoperfusion was subtle and was localized to the right temporal region. Interestingly, the localization of hypoperfusion in the baseline was the same for both reviewers and was partially concordant with the EEG onset location of the seizure that was captured for the postictal scan and in the same lobe as identified in the subtraction CTP results. Although partially concordant with the EEG of the captured seizure, the location of the baseline hypoperfusion was ultimately contralateral to the final presumed SOZ (left hemispheric, orbitofrontal). Both reviewers also gave a lower confidence score on the assessments of baseline CTP images.
Subtraction CTP
Localization of postictal hypoperfusion and areas of seizure spread: quantitative sCTP
Table 7 summarizes the results of sCTP localization of postictal hypoperfusion and additional areas of seizure spread. Localized postictal hypoperfusion was detected in 12/13 patients (92%; patients 1–10, 12, and 14). One patient (patient 11) who had a focal aware seizure without any EEG changes showed no significant perfusion changes. Nine patients had unilateral postictal hypoperfusion, with a focal distribution in two (patients 6 and 7), multifocal distribution with focal prominence in four (patients 1, 2, 8, and 9), hemispheric distribution in two (patients 3 and 12) with patient 12 having focal prominence, and regional distribution with focal prominence in one (patient 4). Three patients had bilateral and multifocal postictal hypoperfusion (patients 5, 10, and 14) with patient 5 having focal prominence. Additional clusters of hypoperfusion identified as areas of seizure spread were seen in 12/13 patients. In addition, the degree of postictal hypoperfusion was positively correlated with seizure duration (Fig. 2; Pearson r = 0.70, two-tailed significance; P = 0.006).
The degree of postictal hypoperfusion is directly related to seizure duration. Scatter plot of the relation between the absolute decrease of CBF (baseline minus postictal scans) in the suspected SOZ and seizure duration. A significant positive correlation was seen between these two measurements. (Pearson r = 0.70; two-tailed significance; P = 0.006)
Concordance with seizure onset zone and areas of seizure spread
Postictal hypoperfusion was seen in 12/13 patients (92%) and the location of hypoperfusion was fully concordant with the presumed SOZ in four patients (patients 1, 4, and 8–9) and partially concordant in six (patient 2–3, 5–6, 10, and 14; Table 7). Specifically, sCTP correctly localized the ictal hemisphere in ten patients and additionally the lobe in five. An example of unilateral focal hypoperfusion concordant with the presumed SOZ is seen in Fig. 3. By comparing to the clinical consensus, sCTP had a sensitivity of 0.91 and a PPV of 0.83; both of which were improvements over the qualitative mode of analysis.
Example of unilateral focal hypoperfusion seen on sCTP in a 60-year-old female with intractable epilepsy ipsilateral to the final presumed SOZ. FDG-PET (top left) localized to the left mesial temporal lobe and hippocampus (arrow). Ictal EEG recording (bottom left) showing seizure onset in the left temporal region (arrow)
The location of maximal postictal hypoperfusion was discordant with the presumed SOZ in two patients (patients 7 and 12). Patient 12 had a presumed SOZ in the left hemisphere, but he also experienced left hemiparesis for over 24 h, suggesting a right-hemispheric involvement. Interestingly, localized postictal hypoperfusion was seen in the right postcentral gyrus in this patient (Fig. 4). The other discordant patient (patient 7) had a focal aware seizure with the shortest seizure duration (29 s) of all patients.
Example of unilateral multifocal hypoperfusion contralateral to the final presumed SOZ. Ictal SPECT (top left) of a different seizure showed right-hemispheric hyperperfusion. Ictal EEG recording (bottom left) of seizure used for CTP study showing seizure onset in the left frontotemporal region (arrow). sCTP (right) demonstrated hypoperfusion in the right hemisphere, contralateral to EEG onset, including the right precentral gyrus. Notably, this patient had left hemiparesis for over 24 h following the same seizure
The location of postictal hypoperfusion reflecting seizure spread was concordant with EEG patterns of seizure spread in all 12 patients that showed postictal hypoperfusion (Table 7). Figure 5 shows an example where the presumed SOZ is highlighted as the most significant cluster with the additional areas of hypoperfusion reflecting seizure spread.
Comparison with clinical imaging data
Of the 12 patients where significant postictal hypoperfusion was seen, eight had lesional MRIs. sCTP was concordant with the MRI findings in six of these patients (patient 1–3, 6–7, and 14) (Table 7). Of all 13 patients studied, sCTP provided similar localization to MRI in five patients (patients 1, 3, 6, and 9–10), superior localization in three (patients 4, 5, and 8), and inferior localization in five (patients 2, 7, and 11–14).
PET was performed in 10/13 patients with significant postictal hypoperfusion and sCTP provided similar localization to PET in five patients (patients 1–5), superior localization in one (patient 8), and inferior localization in four (patients 9, 11–12, and 14; Table 7).
Ictal SPECT was performed in 11/13 patients and sCTP provided similar localization to ictal SPECT in five patients (patients 4–6, 8, and 12), superior localization in two (patients 3 and 9), and inferior localization in four (patients 2, 7, 11, and 14). In seven patients (patients 2–5, 7–8, and 11), postictal CTP was performed on the same seizure as the ictal SPECT. This facilitated direct comparison of postictal CTP to ictal SPECT. sCTP localization in these cases was similar in three (patients 4–5 and 8), superior in one (patient 3), and inferior in three (patients 2, 7, and 11; Table 7). Interestingly, 2/3 patients where sCTP was inferior to ictal SPECT had focal aware seizures and the remaining patient had sCTP localizing to right temporal lobe whereas ictal SPECT localized to right frontal lobe.
Patients with previous epilepsy surgery
Two patients had previous epilepsy surgery. Patient 3 had a previous left temporal lobectomy and amygdalohippocampectomy and patient 9 had a previous right anterior insular resection. In both cases, sCTP displayed unilateral multifocal perfusion patterns that were fully concordant with the presumed SOZ and with the EEG of the captured seizure.
Potential confounders
Several cofounders were considered including late CTP data acquisition (> 65 min postictal), postictal CTP scanning following focal aware seizures, epilepsy etiology, seizure duration, and duration of epilepsy. Of these potential confounders, late acquisition and seizure type may have been the greatest contributors to the absence of significant postictal hypoperfusion. Postictal CTP studies were performed more than 65 min after seizure termination in three patients (patients 7, 12, and 14). The location of postictal hypoperfusion for two of these patients was discordant with the presumed SOZ (patients 7 and 12). A focal aware seizure was captured for two patients; one patient did not have any EEG changes and showed no postictal hypoperfusion (patient 11) and the other had sparse regions of hypoperfusion in parasagittal regions contralateral to the presumed SOZ and was thus discordant (patient 7).
Discussion
To our knowledge, this is the first systematic study of immediate postictal hypoperfusion detected using CTP both qualitatively through visual assessments by expert reviewers and quantitatively through subtraction of postictal and baseline images. We have shown that sCTP can be safely and rapidly performed in patients during the postictal period and is able to detect localized hypoperfusion corresponding to the presumed SOZ and areas of seizure propagation. When baseline and postictal CBF images were visually compared side-by-side, localized hypoperfusion was detected by at least one reviewer in 9/13 (69%) of patients. Reviewer 1 assessments were concordant to the presumed SOZ in 57% (1.25 mm) and 65% (5 mm) of patients; reviewer 2 assessments were concordant to the presumed SOZ in 69% (1.25 mm) and 61% (5 mm). When quantitatively subtracted from the patient’s baseline perfusion scan, CTP demonstrated postictal reductions of > 15 CBF units in 12/13 patients (92%). The location of the hypoperfusion was partially concordant with the presumed SOZ in 6/12 (50%) of these patients and fully concordant with the presumed SOZ in 4/12 (33%) of these patients. Additionally, all patients localized additional clusters of hypoperfusion in areas of seizure propagation concordant with ictal VEEG.
Determining the seizure onset zone
Our study was strengthened by comparing postictal CTP CBF images directly to ictal VEEG recordings, which are the current gold standard for SOZ localization. Specifically, we had accurate VEEG data about timing and duration the seizure, as well as the type, clinical features, and EEG localization of the captured seizure. Most previous CTP studies retrospectively investigated patients that presented to stroke centers and subsequently classified seizures and the location of the presumed SOZ based on patient clinical history alone [12,13,14, 17]. In these studies, most patients did not undergo VEEG confirmation, and seizure descriptions were based on witness accounts. A few ASL studies used interictal scalp VEEG to determine the presumed SOZ [10, 18], but none apart from a recent study from our group used concurrent ictal VEEG recordings [15].
Previous studies have shown that interictal SPECT may lead to false localization, has a lower sensitivity (0.44), and correctly localized the SOZ in 49% of patients, whereas ictal SPECT had higher sensitivity (0.97) and correctly localizing in 81% of patients [19, 20]. Given the dynamic nature of CBF changes, interictal haemodynamic changes do not consistently correlate with the SOZ and may not be a dependable marker of the SOZ, especially in patients with structural abnormalities [10, 18]. One ASL study showed interictal hypoperfusion in 81% of patients up to 60 days after seizures [21]. In patients in whom ASL images were acquired ≤ 3 days following seizures, interictal hypoperfusion, when present, was completely or partially concordant in 32% and 67% of cases, respectively. However, none of these patients underwent continuous VEEG immediately prior to the ASL study, so undetected seizures may have confounded these results.
We recently prospectively collected postictal scans within 90 min of electrographically confirmed seizures and had accurate recordings between seizure termination and ASL data collection [15]. Although postictal hypoperfusion detected by ASL was reliably localizing in 80% of patients and does not involve radiation or administration of a contrast agent, it is logistically difficult to gain rapid access to MR scanners. The present study aimed to provide an alternative to ASL imaging, as CTP is more logistically feasible and easier to implement clinically for collecting immediate postictal CBF data.
Timing of postictal hypoperfusion
Currently, there exists no clear definition of the duration of the postictal period. This has led studies to postictal imaging with acquisition times ranging from several minutes to several weeks following a seizure [7, 9, 11,12,13,14, 17, 22]. Additionally, because many of these studies did not employ ictal VEEG, seizure onset was based on witness accounts leading to inaccurate measures of the time passed between seizure termination and image acquisition.
A recent animal study from our center systematically evaluated postictal hypoperfusion and hypoxia and found that these changes consistently lasted for approximately 60 min at the SOZ before returning to pre-seizure values [6]. This temporal characterization of postictal hemodynamic changes may explain why previous postictal studies obtained conflicting or non-localizing results. Previous ASL measurements in other studies that yielded postictal hypoperfusion or hyperperfusion may have been affected by the different time points at which ASL data were obtained. A previous study showed that CTP performed within approximately 30–90 min of seizure termination is associated with persisting localizing hyperperfusion in all patients with status epilepticus and lateralizing hypoperfusion in only 30% of patients during the postictal period [13]. The authors did not report the timing of CTP acquisition for each patient nor was seizure termination confirmed by VEEG. Another study found that patients scanned more than 2 h after seizure termination showed significantly fewer abnormal perfusion patterns than those scanned within 2 h [12]. These findings further illustrate the transient and variable nature of the postictal perfusion changes and suggest that the timing of postictal image acquisition is critical.
We recently completed a systematic study of postictal CBF changes in patients with drug-resistant focal epilepsy using ASL measurements obtained within 90 min of a seizure confirmed by VEEG [15]. Postictal hypoperfusion was seen in 71% of patients, and in 80% of these patients, the area of maximal hypoperfusion corresponded to the presumed SOZ. Patients who had late postictal scans (> 60 min) showed no significant hypoperfusion. Similarly, the present study obtained postictal CTP within 80 min of seizure termination and partial or fully concordant postictal hypoperfusion corresponding to the presumed SOZ was seen in 83% of patients. Thus, both studies emphasize the need to perform postictal studies prospectively, within an accurately measured and predetermined time window and under continuous VEEG monitoring.
Statistical analysis of visual assessments
No previous CTP study to our knowledge collected both interictal and acute postictal scans for comparison. All previous studies retrospectively identified patients who presented at stroke centers with neurological deficits and underwent CTP imaging. Single scans were collected, typically outside the acute postictal period (< 60 min of seizure onset). Neuroradiologists visually reviewed the scans for CBF abnormalities relative to the contralateral hemisphere [12, 17, 22]. In comparison, our study prospectively recruited patients and collected two CTP scans (acute postictal and interictal baseline) and directly compared the two scans. Our study also justifies the need to acquire a baseline and postictal scan for optimal visualization of localized postictal hypoperfusion.
Localization, PPV, and sensitivity were highest in the side-by-side comparisons (1.25 mm = 70%, 0.650, 0.613; 5 mm = 92%, 0.825, 0.864; values shown are the average between both reviewers) and lowest in the independent assessment of baseline scans (1.25 mm = 23%, 0.5, 0.146; 5 mm = 15%, 0.250, 0.111). Indeed, visual assessment of a single scan was minimally localizing, especially if it was an interictal scan. Thus, the failure to obtain two scans along with the acquisition of single interictal scans may explain why previous CTP studies did not identify localizing hypoperfusion. In contrast, in the current study, focal hypoperfusion can be visually detected in 70% of patients (for 1.25 mm thickness) in the side-by-side comparisons of baseline and postictal scans.
Kappa values between reviewers were highest in the 1.25-mm-thick slices compared to the 5-mm-thick slices. We believe that reviewers were generally more confident in their assessments of the 1.25-mm slices because genuine CBF changes would persist for multiple slices making them easier to identify and discount. Reviewers also noted that the 5-mm-thick slices were more susceptible to partial volume artifacts. For these reasons, the quantitative analysis was performed on 1.25-mm-thick slices.
Subtraction CTP technique
Previous CTP studies performed one acquisition, oftentimes in the interictal period, and perfusion maps were assessed either by visual inspection or by a statistical comparison of CBF values from one hemisphere to the other [12, 14, 17, 22]. We found that visual assessment of CBF images was minimally localizing when examining only one scan, especially interictal scans. Statistical contralateral comparison also assumes that the contralateral hemisphere is normally perfused. We saw that this is not always the case as sCTP not only highlighted the SOZ but also hypoperfusion in areas of presumed seizure propagation, often in the contralateral hemisphere (Fig. 5). Similarly, most previous ASL studies collected interictal CBF data and used statistical comparisons with healthy controls to quantify CBF changes. However, minor differences in interictal CBF maps between patients and controls may provide inaccurate localization of the SOZ. One ASL study from our lab circumvented these limitations by comparing postictal scans obtained within 90 min of seizure termination to interictal scans obtained from the same patients [15].
CBF is dynamic and differences in diet, caffeine consumption, medication changes, and circadian rhythms may influence global CBF. Thus, the challenge of collecting two CBF scans lies in how to quantitatively compare two CBF images collected at different times points in addition to different states of health. During visual assessments, the postictal scans were often found to be globally hypoperfused relative to baseline, with further hypoperfusion within the SOZ. With this global change, a voxel-by-voxel subtraction analysis would prove to be non-localizing when viewed using an absolute threshold. To account for potential global shifts in CBF range, we performed intensity normalization to globally shift postictal scans and baseline scans to be in the same range. This allowed for a direct subtraction that highlighted focal areas of perfusion differences.
Special case: patient 12—prolonged postictal hemiparesis ipsilateral to the side of seizure onset
The location of postictal hypoperfusion detected by sCTP for patient 12 was discordant with the presumed SOZ (Fig. 4). Specifically, sCTP showed areas of hypoperfusion in the right pre- and postcentral gyrus, right orbitofrontal, and right temporal regions. These results were contralateral to the location of EEG onset of the captured seizure and the presumed SOZ which was felt to be in the left frontotemporal region. However, patient 12 experienced postictal left hemiparesis lasting over 24 h following the same event, suggesting significant right hemisphere involvement, either from ictal onset or seizure propagation. Interestingly, ictal SPECT of a different seizure also showed scattered hyperperfusion in the right hemisphere, including the frontal and parietal convexities. Thus, the sCTP results of patient 12, supported by the ictal SPECT, may be an example where conventional presurgical evaluation may provide inaccurate localization of the true epileptogenic zone. Notably, this patient has not undergone surgical resection, so final confirmation of the true epileptogenic zone is not available.
Hypoperfusion on CTP—visual analysis versus subtraction CTP
Visual evaluation was performed to determine if localizing hypoperfusion can be identified in postictal CBF data relative to baseline CBF data. Subtraction analysis was performed in an effort to remove the subjectivity of visual assessments and to provide a quantitative measure of postictal hypoperfusion. Although visual assessment correctly lateralized the SOZ, it lacked spatial resolution as only lobar localization was the most precise localization that could be achieved. sCTP lateralized and localized to the presumed SOZ with higher spatial resolution compared to visual assessments.
sCTP versus neuroimaging data
In our study, CTP offered similar or better localization than MRI, ictal SPECT, and PET in 61%, 63%, and 60% of cases, respectively. More specifically, sCTP was superior to MRI in 3/13 (23%) cases, to SPECT in 2/11 (18%) cases, and to PET in 1/10 (10%) cases. sCTP was inferior to these studies (MRI 38%, ictal SPECT 36%, PET 40%), possibly for the following reasons: a focal aware seizure was captured which may be associated with less postictal hypoperfusion (patients 7 and 11), late postictal scan (patient 14), or diffuse EEG onset (patient 2). In previous studies, ictal SPECT has yielded variable success in localizing the SOZ, ranging 66–97%, depending on temporal or extratemporal localization [23,24,25]. In comparison, previous interictal PET studies identified the SOZ varied in 30–60% cases [26].
Similar to sCTP, subtraction ASL (sASL) was superior or equal to MRI in 52% of cases, to ictal SPECT in 60% of cases, and to PET in 71% of cases [15]. Among patients who demonstrated perfusion changes, both sCTP and sASL lateralized to the same hemisphere as the final presumed SOZ in approximately 70% of cases (sCTP = 10/14 patients, sASL = 10/15). Thus, CTP offers similar SOZ localizing information to subtraction ASL, ictal SPECT, and interictal PET and is more cost-effective.
Clinical applicability
Although our study cohort was small (see “Limitations”), sCTP can be a reliable method of localizing the hemisphere and oftentimes the lobe of the presumed SOZ as determined by other conventional means. In general, approximately 60% of patients with temporal lobe epilepsy and 25–40% of patients with extratemporal epilepsy achieve long-term postoperative seizure freedom [27, 28]. Thus, there is a need for additional tools to aid in the localization of the SOZ. While we acknowledge that sCTP has some limitations, its performance is similar or superior to MRI, ictal SPECT, or PET in about 60% of cases. Thus, sCTP can be a reliable alternative to other tests in patients in whom routine investigations are uninformative or to confirm results of these investigations. Importantly, sCTP may be particularly useful in centers that do not have some tests readily available (e.g., ictal SPECT or PET). sCTP may also help clarify the results of other tests and distinguish between areas of seizure onset and seizure spread.
CTP should be relatively easy to implement in most epilepsy centers. It is a readily available tool as most hospitals have CT scanners and CTP protocols already in place that are available 24/7, especially if they care for patients with strokes or transient ischemic attacks. sCTP can be quickly and easily performed following the collection of two scans. Once manual selection of the arterial input function is complete for each scan, the remainder of the sCTP analysis pipeline is automated and results can be easily interpreted by specialists, generalists, or medical trainees. sCTP provides a practical clinical alternative to SPECT given the lower cost, comparative logistical ease of data collection, and equivalent to superior performance. With additional investigation, sCTP has the potential to be a valuable diagnostic tool that can improve the accuracy of localizing the SOZ.
Confounding factors
Previous surgery
Because we did not exclude patients with previous surgery, visual reviewers may have been biased in their assessments by the presence of obvious post-surgical changes. The anatomical changes may have also influenced registration of CBF maps during quantitative analysis. If the areas of resection are mis-registered, then peri-resection artefactual CBF differences may be observed. Both patients that had previous surgeries had unilateral multifocal sCTP localization (patients 3 and 9). Through subtraction analysis, patients 3 and 9 were partially and fully concordant with the presumed SOZ, respectively. Ultimately, we believe that previous surgery did not significantly alter the final localization of the SOZ as determined by CTP in these patients.
Focal aware seizures
Two patients experienced focal aware seizures with short durations (patients 7 and 11), and one had no EEG change (patient 11). These factors may have influenced why patient 7 displayed very small clusters of hypoperfusion, which were discordant with the presumed SOZ, and why patient 11 had no clusters of significant postictal hypoperfusion. These results are consistent with our previous ASL study [15] and with ictal SPECT in which focal aware seizures are associated with little, if any, localized hyperperfusion [29].
Timing of CTP
Previous animal work suggested that the period of most severe hypoxia following a seizure occurs between 20 and 60 min [6], highlighting the importance of the accurate timing for investigating postictal hypoperfusion. Our cohort involved three patients that had postictal CTP scans longer than 65 min after seizure termination (patients 7, 12, and 14). Patients 7 and 14 experienced a focal aware seizure and focal impaired awareness seizure, respectively, and both showed minimal hypoperfusion. In contrast, patient 12 had a bilateral tonic-clonic seizure and displayed multifocal hypoperfusion, likely reflective of the more significant seizure type that was experienced compared to the other two patients.
Interictal baseline EEG
Five patients had frequent interictal discharges. Although this was not a factor in all patients with multifocal postictal hypoperfusion, 4/5 patients with an active interictal baseline EEG had multifocal postictal hypoperfusion, and the remaining patient experienced a focal aware seizure and showed mild hypoperfusion in the subcortical structure that was ultimately discordant with the presumed SOZ. We suspect that frequent interictal epileptiform discharges could possibly lead to widespread mild CBF reductions as documented in recent studies [10, 30]. Thus, comparing such a patient’s baseline CBF to postictal CBF may not yield significant CBF differences. Alternatively, areas that would normally be cancelled out might be overrepresented, leading to widespread CBF changes.
Intensity normalization
Global CBF values can be different at different time points even within healthy individuals, posing a challenge when performing a subtraction analysis between two sets of CBF data. The effects of hyper- or hypo-ventilation during a seizure or drug/caffeine consumption may also influence postictal or baseline CBF measurements. To address this issue, an intensity normalization step was performed whereby the intensity of postictal images was globally shifted to have the same average and standard deviation as the baseline images. By doing this, differences between the image sets will be more likely related to pathological changes from the ictal event rather than physiological differences related to acquiring data at two different time points. A limitation of this approach, however, is the possibility that the magnitude/extent of postictal hypoperfusion may be underestimated and that the relationship between the degree of hypoperfusion and seizure duration may be altered (Fig. 2).
Limitations
Despite showing good concordance of postictal hypoperfusion to the presumed SOZ, our study cohort was small and could not be validated by post-surgical outcome, as only one patient underwent surgery to date. Thus, a larger scale study with longer term follow-ups is needed to determine if sCTP can systemically lead to equivalent or superior detection of the SOZ compared to conventional methods.
Similarly, the use of clinical consensus as the gold standard for the presumed SOZ in statistical analysis and comparisons for concordance is another limitation as the true epileptogenic zone can only be determined post-surgically. Thus, there is a possibility that the presumed SOZ may not be concordant with the epileptogenic zone. Indeed, two of the patients underwent further intracranial monitoring, showing that the presurgical assessment using scalp VEEG data may not be conclusive. Another limitation is the fact that EEG electrodes were removed ~ 20 min prior to each CTP scan, leading to the possibility of missing electrographic seizures during transport to the CT scanner and image acquisition.
Additional investigation of the necessary post-processing steps is still required. The difference between partially and fully concordant results may be influenced by registration errors, adjustments of smoothing kernel parameters, and/or normalization techniques.
A limitation of Cohen’s kappa is that it reflects the agreement between the sensitivity and specificity of raters and is therefore dependent upon the trait prevalence. In this study, the statistic would vary based on whether hypoperfusion is expected to be present or not. For interictal scans, hypoperfusion was not expected to be prevalent and most reviews were non-localizing, which resulted in low kappa coefficients despite good overall agreement between reviewers. Additionally, unlike comparative statistics, there is no intrinsic significance associated with interrater reliability metrics. Instead, investigators rely on established benchmarks to report qualitative descriptions of the quantitative measures.
We justified the use of 15 CBF units as an absolute threshold to view sCTP hypoperfusion based on our previous animal work and ASL human data. However, we recognize that other methods of analyzing postictal CBF changes may be considered. Indeed, using a different thresholds or smoothing parameters may yield different results.
Conclusions
Subtraction CTP is a safe, feasible, cost-effective, and readily available imaging modality that can be used to measure postictal hypoperfusion that may help localize the SOZ. When the CTP study is performed within 80 min of the end of a seizure that is not focal aware, hypoperfusion is seen in up to 80% of patients. Although ASL does not involve radiation and has been shown to be an effective modality in detecting postictal hypoperfusion, rapid access to MRI scanners poses a challenge for its clinical implementation. Given the favorable comparisons with subtraction ASL, ictal SPECT, and PET, sCTP may be an additional tool for identifying the SOZ.
Abbreviations
- ASL:
-
Arterial spin labeling
- CBF:
-
Cerebral blood flow
- SOZ:
-
Seizure onset zone
- CTP:
-
Computed tomography perfusion
- SPECT:
-
Single photon emission computed tomography
- PET:
-
Positron emission tomography
- EEG:
-
Electroencephalography
- VEEG:
-
Video-electroencephalography
- PPV:
-
Positive predictive value
- sCTP:
-
Subtraction CTP
- ROI:
-
Region of interest
References
Laxer KD, Trinka E, Hirsch LJ, Cendes F, Langfitt J, Delanty N, Resnick T, Benbadis SR (2014) The consequences of refractory epilepsy and its treatment. Epilepsy Behav 37:59–70
Salmenpera TM, Duncan JS (2005) Imaging in epilepsy. J Neurol Neurosurg Psychiatry 76(Suppl 3):iii2–iii10
Jobst BC, Cascino GD (2015) Resective epilepsy surgery for drug-resistant focal epilepsy: a review. JAMA 313(3):285–293
Choy M, Wells JA, Thomas DL, Gadian DG, Scott RC, Lythgoe MF (2010) Cerebral blood flow changes during pilocarpine-induced status epilepticus activity in the rat hippocampus. Exp Neurol 225(1):196–201
Weinand ME, Carter LP, el-Saadany WF, Sioutos PJ, Labiner DM, Oommen KJ (1997) Cerebral blood flow and temporal lobe epileptogenicity. J Neurosurg 86(2):226–232
Farrell JS, Gaxiola-Valdez I, Wolff MD, David LS, Dika HI, Geeraert BL, Rachel Wang X, Singh S, Spanswick SC, Dunn JF, Antle MC, Federico P, Teskey GC (2016) Postictal behavioural impairments are due to a severe prolonged hypoperfusion/hypoxia event that is COX-2 dependent. Elife 5
Guo X, Xu S, Wang G, Zhang Y, Guo L, Zhao B (2015) Asymmetry of cerebral blood flow measured with three-dimensional pseudocontinuous arterial spin-labeling MR imaging in temporal lobe epilepsy with and without mesial temporal sclerosis. J Magn Reson Imaging 42(5):1386–1397
Lim YM, Cho YW, Shamim S, Solomon J, Birn R, Luh WM, Gaillard WD, Ritzl EK, Theodore WH (2008) Usefulness of pulsed arterial spin labeling MR imaging in mesial temporal lobe epilepsy. Epilepsy Res 82(2–3):183–189
Pizzini FB, Farace P, Manganotti P, Zoccatelli G, Bongiovanni LG, Golay X, Beltramello A, Osculati A, Bertini G, Fabene PF (2013) Cerebral perfusion alterations in epileptic patients during peri-ictal and post-ictal phase: PASL vs DSC-MRI. Magn Reson Imaging 31(6):1001–1005
Storti SF et al (2014) Combining ESI, ASL and PET for quantitative assessment of drug-resistant focal epilepsy. Neuroimage 102(Pt 1):49–59
Wolf RL, Alsop DC, Levy-Reis I, Meyer PT, Maldjian JA, Gonzalez-Atavales J, French JA, Alavi A, Detre JA (2001) Detection of mesial temporal lobe hypoperfusion in patients with temporal lobe epilepsy by use of arterial spin labeled perfusion MR imaging. AJNR Am J Neuroradiol 22(7):1334–1341
Gelfand JM, Wintermark M, Josephson SA (2010) Cerebral perfusion-CT patterns following seizure. Eur J Neurol 17(4):594–601
Hauf M, Slotboom J, Nirkko A, von Bredow F, Ozdoba C, Wiest R (2009) Cortical regional hyperperfusion in nonconvulsive status epilepticus measured by dynamic brain perfusion CT. AJNR Am J Neuroradiol 30(4):693–698
Mathews MS, Smith WS, Wintermark M, Dillon WP, Binder DK (2008) Local cortical hypoperfusion imaged with CT perfusion during postictal Todd's paresis. Neuroradiology 50(5):397–401
Gaxiola-Valdez I, Singh S, Perera T, Sandy S, Li E, Federico P (2017) Seizure onset zone localization using postictal hypoperfusion detected by arterial spin labelling MRI. Brain 140(11):2895–2911
McHugh ML (2012) Interrater reliability: the kappa statistic. Biochem Med (Zagreb) 22(3):276–282
Shelly S et al (2017) Computed tomography perfusion maps reveal blood flow dynamics in postictal patients: a novel diagnostic tool. Isr Med Assoc J 19(9):553–556
Sierra-Marcos A, Carreño M, Setoain X, López-Rueda A, Aparicio J, Donaire A, Bargalló N (2016) Accuracy of arterial spin labeling magnetic resonance imaging (MRI) perfusion in detecting the epileptogenic zone in patients with drug-resistant neocortical epilepsy: comparison with electrophysiological data, structural MRI, SISCOM and FDG-PET. Eur J Neurol 23(1):160–167
Devous MD Sr et al (1998) SPECT brain imaging in epilepsy: a meta-analysis. J Nucl Med 39(2):285–293
Spanaki MV, Spencer SS, Corsi M, MacMullan J, Seibyl J, Zubal IG (1999) Sensitivity and specificity of quantitative difference SPECT analysis in seizure localization. J Nucl Med 40(5):730–736
Kim BS, Lee ST, Yun TJ, Lee SK, Paeng JC, Jun J, Kang KM, Choi SH, Kim JH, Sohn CH (2016) Capability of arterial spin labeling MR imaging in localizing seizure focus in clinical seizure activity. Eur J Radiol 85(7):1295–1303
Wiest R, von Bredow F, Schindler K, Schauble B, Slotboom J, Brekenfeld C, Remonda L, Schroth G, Ozdoba C (2006) Detection of regional blood perfusion changes in epileptic seizures with dynamic brain perfusion CT—a pilot study. Epilepsy Res 72(2–3):102–110
Lee JJ, Lee SK, Lee SY, Park KI, Kim DW, Lee DS, Chung CK, Nam HW (2008) Frontal lobe epilepsy: clinical characteristics, surgical outcomes and diagnostic modalities. Seizure 17(6):514–523
Weil S et al (2001) Ictal ECD-SPECT differentiates between temporal and extratemporal epilepsy: confirmation by excellent postoperative seizure control. Nucl Med Commun 22(2):233–237
Zaknun JJ, Bal C, Maes A, Tepmongkol S, Vazquez S, Dupont P, Dondi M (2008) Comparative analysis of MR imaging, ictal SPECT and EEG in temporal lobe epilepsy: a prospective IAEA multi-center study. Eur J Nucl Med Mol Imaging 35(1):107–115
Ryvlin P et al (1998) Clinical utility of flumazenil-PET versus [18F]fluorodeoxyglucose-PET and MRI in refractory partial epilepsy. A prospective study in 100 patients. Brain 121(Pt 11):2067–2081
Tellez-Zenteno JF, Dhar R, Wiebe S (2005) Long-term seizure outcomes following epilepsy surgery: a systematic review and meta-analysis. Brain 128(Pt 5):1188–1198
Cohen-Gadol AA, Wilhelmi BG, Collignon F, White JB, Britton JW, Cambier DM, Christianson TJH, Marsh WR, Meyer FB, Cascino GD (2006) Long-term outcome of epilepsy surgery among 399 patients with nonlesional seizure foci including mesial temporal lobe sclerosis. J Neurosurg 104(4):513–524
Ho SS, Berkovic SF, McKay WJ, Kalnins RM, Bladin PF (1996) Temporal lobe epilepsy subtypes: differential patterns of cerebral perfusion on ictal SPECT. Epilepsia 37(8):788–795
Boscolo Galazzo I, Storti SF, del Felice A, Pizzini FB, Arcaro C, Formaggio E, Mai R, Chappell M, Beltramello A, Manganotti P (2015) Patient-specific detection of cerebral blood flow alterations as assessed by arterial spin labeling in drug-resistant epileptic patients. PLoS One 10(5):e0123975
Funding
This study was funded by the Canadian Institutes of Health Research (FRN-156020) and the University of Calgary Brain and Mental Health Strategic Research Fund.
Author information
Authors and Affiliations
Corresponding author
Ethics declarations
Conflict of interest
The authors declare that they have no conflict of interest.
Ethical approval
All procedures performed in studies involving human participants were in accordance with the ethical standards of the institutional and/or national research committee and with the 1964 Helsinki declaration and its later amendments or comparable ethical standards.
Informed consent
Informed consent was obtained from all individual participants included in the study.
Additional information
Publisher’s note
Springer Nature remains neutral with regard to jurisdictional claims in published maps and institutional affiliations.
Y. Agha-Khani, R. Avendano, S. Hanna, W. F. Murphy and N. Pillay to be collectively listed as The Calgary Comprehensive Epilepsy Program collaborators
Rights and permissions
About this article
Cite this article
Li, E., d’Esterre, C.D., Gaxiola-Valdez, I. et al. CT perfusion measurement of postictal hypoperfusion: localization of the seizure onset zone and patterns of spread. Neuroradiology 61, 991–1010 (2019). https://doi.org/10.1007/s00234-019-02227-8
Received:
Accepted:
Published:
Issue Date:
DOI: https://doi.org/10.1007/s00234-019-02227-8