Abstract
Rationale
Validating preclinical biomarkers that predict treatment efficacy remains a critical imperative for neuropsychiatric drug discovery. With the establishment of novel in vivo imaging methods, it has become possible to think how such translational proof-of-concept studies may look.
Objectives
The aim of this study was to use in vivo oxygen (O2) amperometry to simultaneously assess the regional and event/task-related O2 changes induced by ketamine challenge in rats, and to determine whether both of these signals are equivalently affected by the mGlu2/3 receptor agonist LY379268.
Methods
O2 signals were measured via carbon paste electrodes implanted in the anterior cingulate cortex (ACC) of rats trained to perform a simple reaction time task (SRT). SRT performance, event-related ACC O2 responses, and regional ACC O2 signal were recorded simultaneously in animals treated with ketamine (10 mg/kg) and/or LY379268 (3 mg/kg).
Results
A consistent relationship was observed between baseline SRT performance and related ACC O2 signals, suggesting that ACC engagement is likely to be a requirement for optimal task performance. Ketamine induced a robust and consistent slowing in reaction times that was reflected by a delayed event-related ACC O2 signal increase compared to vehicle controls. Ketamine also produced a regional and task-independent 60-min increase in ACC O2 levels which was effectively attenuated by LY379268. However, LY379238 failed to reverse alterations in event-related O2 signals and associated SRT task performance.
Conclusions
These findings raise questions about the degree to which such reversals of regional ketamine O2 signals could potentially be claimed to predict drug treatment efficacy.
Similar content being viewed by others
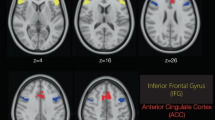
Avoid common mistakes on your manuscript.
Introduction
Despite remarkable advances in genetics and imaging technologies, there have been few truly innovative chemical entities approved for use in the treatment of neuropsychiatric diseases in recent years. Many assets have simply failed to meet primary endpoint criteria in clinical trials, often as late as phase III (Arrowsmith and Miller. 2013). A potential root cause of these failures has been the lack of translational biomarkers capable of predicting treatment efficacy (Morgan et al. 2012).
Validation of preclinical biomarkers that can predict efficacy remains a critical imperative for neuropsychiatric drug discovery. With the establishment of novel in vivo imaging methods, such as blood oxygen level-dependent pharmacological magnetic resonance imaging (BOLD phMRI) and constant potential oxygen (O2) amperometry, it has become possible to think how such translational proof-of-concept studies may look. Recently, attempts have been made to modulate the resting-state BOLD phMRI and O2 amperometry responses to the NMDA receptor antagonist ketamine with various classes of pharmacology including novel putative antipsychotics, in both rodents (Chin et al. 2011; Baker et al. 2012; Li et al. 2014) and humans (Doyle et al. 2013). While offering a valuable pharmacodynamic biomarker that may facilitate dose selection and perhaps indirectly index target engagement, such reversal studies have prompted discussion about the degree to which predictions of treatment efficacy should be concluded from the attenuation of regional ketamine O2 responses in resting-state subjects.
Given that many of the behavioural effects of ketamine have been considered to inform about mechanistic processes underlying aspects of schizophrenia symptomatology (Gilmour et al. 2012), it can be tempting to speculate that modulation of ketamine-induced neuronal activation also predicts that the pharmacology under study will modulate the behavioural effects of ketamine, and by extension, the symptoms of schizophrenia itself. At present, such speculation has rarely, if ever, been confirmed by experiment. Constant potential in vivo O2 amperometry may serve as a better surrogate of the BOLD fMRI signal in this context, as it allows the monitoring of regional brain tissue O2 levels in freely moving animals (Lowry et al. 1997) and has already been successfully employed in rodent behavioural paradigms (McHugh et al. 2011; Francois et al. 2012; Francois et al. 2014), some of which have homologues that could be applied in clinical settings.
Therefore, the aim of this study was to use in vivo O2 amperometry to simultaneously assess the regional task-independent and task-dependent O2 signal responses induced by ketamine in rats, and to determine whether both of these signals are equivalently affected by the mGlu2/3 receptor agonist LY379268. LY379268 was the agent chosen for this study, as compounds of this class have been reported to have putative antipsychotic and procognitive effects in a number of neuropsychiatric research paradigms, including NMDA receptor antagonist-induced behavioural alterations in rodents (Moghaddam and Adams. 1998; Cartmell et al. 1999; Imre et al. 2006; Rorick-Kehn et al. 2007; Pitsikas and Markou. 2014) and human volunteers (Krystal et al. 2005). LY379268 has also been reported to attenuate a ketamine-induced regional BOLD signal in an awake rodent phMRI study (Chin et al. 2011). To test the generalizability of drug effects against the session-averaged regional ketamine O2 signal to that of a regionally selective, event-related O2 signal, a simple reaction time (SRT) task was chosen. Given the overall aims of the study and despite known effects of mGlu2/3 receptor agonists in working memory paradigms (Moghaddam and Adams. 1998; Krystal et al. 2005; Pitsikas and Markou. 2014), the SRT task offered several advantages, including the following: (i) translational potential and ease of implementation in an implanted animal methodology; (ii) known deficits in reaction time across a wide range of diseases, including schizophrenia (Vinogradov et al. 1998; Ngan and Liddle. 2000; Birkett et al. 2007; Dickinson et al. 2007); (iii) known disruptive effects of ketamine on SRT measures (Gastambide et al. 2013); and (iv) prior knowledge that efficient performance of the behavioural domain tested by SRT is dependent on engagement of ACC (Naito et al. 2000; Paus. 2001; Mulert et al. 2003; Drummond et al. 2005; Stuss et al. 2005; Shallice et al. 2008).
Methods and materials
See Supplementary information for more detailed description of the methods used in these studies.
Study overview
This study consisted of three independent blocks of experiments, which shared overlapping aspects of methodology as described below. The first two experiments were designed to confirm the engagement of ACC circuitry in the rodent SRT task, using reversible inactivation of the ACC via infusion of the GABAA receptor agonist muscimol (Experiment 1) and the measurement of task-related O2 responses in the ACC via implantation of carbon paste electrodes (Experiment 2). Session-averaged regional and task-related changes induced by ketamine were also assessed during these studies. The third experiment was designed more specifically to investigate the effects of the mGlu2/3 receptor agonist LY379268 on both ketamine-induced behavioural and O2 changes (Experiment 3).
Drugs and study design
For the ACC inactivation experiment (Experiment 1), the GABAA receptor agonist muscimol (Sigma-Aldrich, UK) was dissolved in 0.9 % physiological saline at a 1 μg/μl dose and infused intra-ACC 30 min before SRT testing. For the ketamine experiments (Experiments 1, 2 and 3), the NMDA receptor antagonist S-(+)-ketamine (Sigma-Aldrich, UK) was dissolved in 5 % glucose and dosed subcutaneously at a 10 mg/kg dose 30 min before testing. For the ketamine reversal study (Experiment 3), the mGlu2/3 receptor agonist LY379268 (Lilly Research Labs) was dissolved in a NaOH 1 M and 5 % glucose solution and dosed intraperitoneally at a 3 mg/kg dose 60 min before testing. Dose and route of administration of muscimol, ketamine and LY379268 were chosen on the basis of previous studies (Imre et al. 2006; Ragozzino and Rozman. 2007; Gilmour et al. 2009; Gastambide et al. 2013). To increase statistical power and reduce variance associated with individual differences, a within-subject design involving treatment of animals over periods of several weeks was used for most of the experiments, except for the first ketamine study (Experiment 1) where a between-subject design was used. To minimise carryover effects, drug administrations were performed no more than once weekly.
Subjects
All experiments were conducted in accordance with the regulations laid down in the United Kingdom Animals (Scientific Procedures) Act, 1986, with the approval of the Lilly Research Laboratories Institutional Animal Care and Use Committee. Upon arrival, adult male Wistar rats (200–250 g; Charles River, UK) were housed in standard housing conditions (0700 to 1900 hours light phase, controlled temperature and humidity, ad libitum water) for a period of 7 days before behavioural training started. During this time, they were acclimated to the food restriction regime (i.e., maintained at no less than 85 % of their free-feeding weight) and were handled regularly. Sample sizes were as follows: Experiment 1, n = 16 for the muscimol inactivation study and n = 32 for the ketamine study; Experiment 2, n = 16; and Experiment 3, n = 24.
Simple reaction time task (Experiments 1, 2 and 3)
For all three experiments, the SRT task was conducted in standard operant chambers housed in sound and light attenuation chambers (Med Associates, USA). Animals were trained daily during 50 min sessions to respond for food reward by making a head entry following presentation of a visual stimulus in the food magazine, according to the protocol described by Gastambide et al. (2013). During the final stage of training and testing sessions, each trial was initiated by illumination of the house light (preparative cue) followed after a 5 s variable interval (range 4–6 s) by illumination of the magazine light (imperative cue). A response during the imperative cue was counted as a trial completed and resulted in the delivery of food reward. An omission was recorded if no response was made during the 10 s imperative cue. Omissions and trials completed were all followed by a 20 s intertrial interval during which both house and magazine lights were switched off. The number of completed trials, omissions and head entries were recorded as well as response latencies (i.e., reaction times). Prior to statistical analyses, logarithmic transformations of response latency measures were conducted to ensure normality of distribution. All parameters were subjected to one-way repeated measures ANOVAs with drug treatment as within-subjects factor and dosing week as repeated measure. Planned comparisons were conducted as appropriate and in all cases, p < 0.05 indicated a significant difference.
Reversible inactivation of anterior cingulate cortex by muscimol (Experiment 1)
Intracranial guide cannula (26 gauge; Bilaney Consultants Ltd, Sevenoaks, UK) were implanted bilaterally, aiming at the ACC (from bregma: AP + 2.0 mm, ML ± 0.5 mm, DV: −1.0 mm). Physiological saline or muscimol was slowly infused at a total volume of 0.5 μl per side, over a period of 2 min. One minute post-infusion, the animal was placed back into its transport cage for 30 min before behavioural testing started.
In vivo oxygen amperometry (Experiments 2 and 3)
Constant potential amperometry
Changes in extracellular tissue oxygen concentration were measured using constant potential amperometry (CPA) at carbon paste electrodes (CPE) as described previously (Lowry et al. 1997). Briefly, a negative potential (−650 mV) was applied to the CPE to allow the electrochemical reduction of dissolved oxygen to occur at the tip of the electrode. Changes in the measured current that are produced by the electrochemical reduction of O2 are directly proportional to the local extracellular tissue O2 concentration (Hitchman 1978).
O2 recordings started after the animals recovered from surgical implantation of the CPEs in the ACC (from bregma: AP + 2.0 mm, ML ± 0.5 mm, DV: −2.0 mm). Two separate analyses were performed to assess the drug effect on (1) the session-averaged regional O2 signal and (2) the task-related signal. For the regional signal, time zero was taken as the time of the injection. To compensate for different baselines between channels, data were normalised by subtracting the average value from a 120-s period preceding the injection. Data were analysed for the entire testing session (i.e., from the drug administration to the end of SRT testing phase). For the task-related signal, each trial type (completed trial or omission) was analysed separately and time zero was taken as the time of houselight/preparatory cue presentation with a 1 s preceding period used as a baseline. Data were analysed for 25 s post-cue onset. As well as analyzing by response type, a second analysis was conducted according to reaction time (RT) distributions. Based on measurement of the median, 5th and 95th percentile RT responses, three RT ranges were initially chosen for analysis (RT < 0.2 s, 0.25 s < RT < 0.75 s, RT > 2 s) but were then narrowed to ensure an equal number of trial responses per range: fastest responses (RT < 0.2 s), median responses (0.4 s < RT < 0.7 s) and slowest responses (RT > 2 s). Although a regression analysis would have been preferable here, the present dataset is limited in this regard by the need for trial averaging to increase signal-to-noise ratio. For all analyses, the area under the curve (AUC), the maximum amplitude (Ypeak) and the time at which the maximum amplitude occurred (Xpeak) were calculated (see illustration in Supplementary Fig. 2A). These extracted measures were analysed using a two-way ANOVA followed when appropriate by a Fisher’s LSD post hoc test for multiple comparisons.
Histology
For all experiments, histological verification of guide cannula or CPE location was performed after behavioural testing on thionin for Nissl substance stained slices as previously described (Francois et al. 2012; Francois et al. 2014).
Results
Histology
Supplementary Figs. 1A and 1B illustrate both intended and representative guide cannula placements in the ACC for muscimol infusion, respectively (Experiment 1). For in vivo O2 amperometry experiments (Experiments 2 and 3), electrode placements were deemed to be correct when they were localised to the anterior extent of the cingulate cortex. Following exclusion of rats with either misplaced electrodes or unstable/noisy O2 signals, nine animals (Experiment 2) and 16 animals (Experiment 3) were deemed appropriate to include in subsequent analyses. Reconstructions of electrode placements in the ACC are shown in Supplementary Fig. 1C (Experiment 2) and Supplementary Fig. 1D (Experiment 3).
Experiment 1
Intra-ACC muscimol infusion and systemic ketamine injection both induced slower and more variable reaction times.
As depicted in Fig. 1a, muscimol inactivation of the ACC resulted in a significant decrease in the number of SRT trials completed (F 1,14 = 6.2, p < 0.05) and a RT slowing (F 1,14 = 18.9, p < 0.001). A more detailed analysis of RT distributions showed that muscimol-induced response slowing was driven by a significantly smaller number of fast responses (treatment by RT interaction F 2,90 = 6.54, p < 0.01; 250 ms < RT < 750 ms muscimol vs. vehicle: p < 0.001) and a trend towards an increase in the number of slow responses (treatment by RT interaction F 2,90 = 6.54, p < 0.01; RT > 2 s vehicle vs. muscimol: p = 0.1).
Intra-ACC muscimol infusion (a) and systemic ketamine injection (b) both induced slower and more variable reaction times (Experiment 1). a Muscimol infusion in the ACC (grey bars) induced a significant decrease in the number of trials completed and an increase in RT compared to vehicle controls (white bars). RT distribution was shifted rightwards, with a decrease in the number of fast responses (250 ms < RT < 750 ms) and an increase in the number of slow responses (RT > 2 s); *p < 0.05; **p < 0.01 compared to vehicle controls. b Ketamine injection (grey bars) induced a significant decrease in the number of trials completed while it significantly increases RT compared to vehicle controls (white bars). RT distribution was also altered with a decrease in the number of fast responses (250 ms < RT < 750 ms) and an increase in the number of slow responses (RT > 2 s); *p < 0.05; **p < 0.01 compared to vehicle controls. All data are presented as mean ± SEM
Similarly to muscimol-induced ACC inactivation, systemic injection of ketamine also significantly decreased the number of trials completed (F 1,27 = 10.06, p < 0.01) and slowed RTs (F 1,26 = 19.1, p < 0.001) (Fig. 1b). Ketamine-induced response slowing was driven both by a significantly smaller number of fast responses (treatment by RT interaction F 2,81 = 32.29, p < 0.0001; 250 ms < RT < 750 ms vehicle vs. ketamine: p < 0.01) and a significantly larger number of slow responses (treatment by RT interaction F 2,81 = 32.29, p < 0.0001; RT > 2 s vehicle vs. ketamine: p < 0.01)
Experiment 2
Relationship between SRT performance and ACC O2 signals at baseline.
As shown in Fig. 2a, SRT-related O2 signals measured in the ACC differed significantly as a function of response type, where the Xpeak measure was significantly faster for trials completed relative to omissions (F 1,9 = 9.37, p < 0.05), concomitant with no significant change in either AUC or Ypeak measures (F 1,9 = 0.56, p > 0.1; F 1,9 = 1.29, p > 0.1, respectively). In qualitative terms, ACC O2 signals for completed trials started to increase during the inter-cue/preparatory period, reaching peak amplitude at 12.7 ± 1.3 s (mean ± SEM) following house light onset. In comparison, the increase in ACC O2 signal during an omission event was delayed until a point after magazine light onset, and reached peak amplitude 24.5 ± 0.5 s following house light onset.
Relationship between SRT performance and ACC O2 signals at baseline (Experiment 2). a Left: average curves of ACC O2 signals associated with animals completing (black circle or bars) or omitting (white circle or bars) a response during the SRT task. Right: extracted parameters of average area under the curve (AUC), maximum amplitude (Ypeak) and time at which the maximum amplitude occurs (Xpeak); **p < 0.001 in comparison to response type. b Left: average curves of ACC O2 signals for different RT ranges. Three RT ranges are depicted: fastest RT range (<0.2 s, white circles and bars); medium RT range (0.4 < RT < 0.7 s, grey circles and bars); and slowest RT range (RT > 2 s black circles and bars). Right: extracted parameters of average area under the curve (AUC), maximum amplitude (Ypeak) and time at which the maximum amplitude occurs (Xpeak) for each RT range. **p < 0.01; ***p < 0.001 in comparison to the fastest RT range. All data are presented as mean ± SEM
ACC O2 signals for completed trials were subjected to more detailed analysis based on a banding of RT distributions in the following ranges: “fastest,” RT < 0.2 s; “medium,” 0.4 s < RT < 0.7 s; and “slowest,” RT > 2 s (Fig. 2b). With regard to AUC, no significant difference could be found between RT ranges. However, the slowest responses had significantly later Xpeak compared to the fastest responses (Xpeak: F 2,286 = 5.13, p < 0.01), and a trend towards greater Ypeak measures (F 2,286 = 1.52, p = 0.07). From a qualitative perspective, it can also be clearly seen that the initial rise in the O2 signal is delayed until well after magazine light onset for the slowest RT range, in comparison to fastest and medium RT ranges. These data suggest that naturally slow RTs are associated with a slowing of the ACC O2 signal increase observed as a trial is completed.
Relationship between SRT performance and ACC O2 signals under ketamine challenge
At the behavioural level, 10 mg/kg ketamine significantly decreased the number of trials completed (F 1,7 = 16.73, p < 0.01, Fig. 3a) and markedly increased RTs from 0.38 ± 0.04 s at baseline to 2.5 ± 0.4 s (F 1,7 = 71.77, p < 0.001), replicating the effects of ketamine administration in Experiment 1 (Fig. 1b). With regard to ACC O2 signals associated with completed trials, ketamine significantly delayed the O2 signal increase as indexed by the Xpeak measure (F 1,7 = 8.71, p < 0.05; Fig. 3b). AUC measures were unchanged after ketamine treatment (F 1,7 = 0.0062, p = 0.94). At the session-length timescale, ketamine induced a long-lasting and significant increase in the ACC O2 level compared to vehicle controls, as reflected by the AUC measure (F 1,7 = 12.18, p < 0.01; Fig. 3c). Together, these findings suggest that ketamine induces alterations in both session-averaged regional and SRT task-related ACC O2 signal responses.
Ketamine effects on SRT performance and related ACC O2 signals (Experiment 2). a Ketamine-induced alterations in SRT task performance. Graphs depict the number of completed trials (left) and median RTs (right) 30 min after ketamine administration (ket) compared to vehicle controls (veh); **p < 0.01; ***p < 0.001 compared to vehicle controls. b Ketamine-induced changes in SRT task-related ACC O2 signals. Average curves (left) and extracted AUC and Xpeak measures for completed trials are presented. For the average curves, the dashed line represents the house light onset while the solid line represents magazine light onset; *p < 0.05 compared to vehicle controls. c. Ketamine-increased session-averaged ACC O2 signals. The dashed line on the left graph represents the time of the injection. Bar graphs on the right represent the average area under the curve (AUC) extracted from the average curve; *p < 0.05 compared to vehicle controls. All data are presented as mean ± SEM
Experiment 3
Replication of baseline SRT performance and ACC O2 signals
In an attempt to replicate previous findings, O2 signals were recorded at baseline (i.e., the day prior to drug administration) and analysed with respect to SRT response type. As previously observed in Experiment 2, the O2 signal differed significantly according to response type for the Xpeak measure (F 1,7 = 14.89, p < 0.01) but not for AUC or Ypeak (F 1,7 = 0.61, p > 0.1; F 1,7 = 2.49, p > 0.05, respectively). ACC O2 signals started to increase as early as the inter-cue/preparatory phase for completed trials, in contrast to omissions for which O2 increases were substantially delayed (Supplementary Fig. 2B). Again, ACC O2 responses were analysed with respect to predefined RT distribution ranges (Supplementary Fig. 2C). Compared to the fastest RT range, the slowest RT range was associated with significantly greater AUC (F 2,475 = 5.45, p < 0.01), Ypeak (F 2,475 = 8.55, p < 0.001) and Xpeak measures (F 2,475 = 6.12, p < 0.001), whereas the medium RT range only showed a significantly greater Ypeak (F 2,475 = 8.55, p < 0.01).
LY379268 attenuation of ketamine-induced session-averaged, task-independent ACC O2 signal increase
LY379268 alone did not have a significant effect on session-averaged ACC O2 levels (AUC: F 3,20 = 2.3, p > 0.1) when analysed during the first 30 min following LY379268 administration and prior to ketamine injection (Fig. 4a). As previously observed in Experiment 2, ketamine induced a robust and long-lasting increase in the session-averaged, task-independent ACC O2 levels (AUC: F 3,28 = 13.31, p < 0.001; veh/veh vs veh/ket : p < 0.001 Fig. 4b). Pretreatment with a 3 mg/kg dose of LY379268 significantly attenuated the ketamine-induced session-averaged ACC O2 signal increase (AUC: F 3,28 = 13.31, p < 0.001; veh/ket vs 268/ket: p < 0.01 Fig. 4b).
LY379268 attenuation of ketamine-induced session-averaged, task-independent ACC O2 signal increase. a Time course (left) and AUC (right) of ACC O2 signals following LY379268 administration alone, measured during the 30 min prior to ketamine injection. b Time course (left) and AUC (right) of ACC O2 signals following ketamine injection in rats pretreated with LY379268 or vehicle; ***p < 0.001 compared to veh/veh group; #p < 0.05 compared to veh/ket group. All data are presented as mean ± SEM
LY379268 failure to normalise ketamine-induced changes in SRT and task-related ACC O2 signals
As previously observed in Experiment 2, systemic ketamine administration induced alterations in both SRT task performance (Fig. 5a) and related O2 signals (Fig. 5b). Indeed, ketamine significantly decreased the number of trials completed (F 3,34 = 36.93, p < 0.001; veh/veh vs. veh/ket: p < 0.01) and slowed RTs (F 3,34 = 14.64, p < 0.001; veh/veh vs. veh/ket: p < 0.01). Ketamine also significantly delayed the O2 signal increases associated with completed trials (Xpeak: F (3,26) = 4.62, p < 0.05; veh/veh vs veh/ket: p < 0.05). Interestingly, LY379268 alone induced very similar alterations. It decreased the number of trials completed (F 3,34 = 36.93, p < 0.001; veh/veh vs. 268/veh: p < 0.001), increased RTs (F 3,34 = 14.64, p < 0.001; veh/veh vs. 268/veh: p < 0.01), and delayed O2 signal increases associated with completed trials (Xpeak: F (3,26) = 4.62, p < 0.05; veh/veh vs 268/ket: p < 0.01). Finally, contrasting with the effects on session-averaged ACC O2 signal, pretreatment with LY379268 failed to normalise both ketamine-induced behavioural and O2 signal changes. It actually further decreased the number of completed trials (F 3,34 = 25.46, p < 0.001; veh/ket vs. 268/ket: p < 0.001), and increased both RTs (F 3,34 = 14.64, p < 0.001; veh/ket vs. 268/ket: p < 0.05) and related ACC O2 signals (AUC: F 3,26 = 5.29, p < 0.01, veh/ket vs. 268/ket: p < 0.01).
LY379268 failure to normalise ketamine-induced changes in SRT and task-related ACC O2 signals. a Completed trials (left) and median RTs (right) measured during the SRT task following ketamine (black bars), LY379268 (dashed bars) and LY379268/ketamine administration (grey bars); *p < 0.05; **p < 0.01; ***p < 0.001 compared to veh/veh group (white bars); #p < 0.05; ###p < 0.001 compared to veh/ket group. b ACC O2 signals associated with SRT task performance. Average curves and extracted AUC and Xpeak measures for completed trials are presented. For the average curves, the dashed line represents the house light onset while the solid line represents magazine light onset; *p < 0.05; **p < 0.01 compared to veh/veh group; ##p < 0.01 compared to veh/ket group. All data are presented as mean ± SEM
Discussion
The present study utilised in vivo O2 amperometry as a proxy for BOLD signals in behaving animals to demonstrate that while the mGlu2/3 receptor agonist LY379268 effectively normalised a session-length, task-independent increase in ACC O2 levels induced by ketamine, it did not normalise alterations in event-related O2 signals or associated SRT task performance. This study demonstrates the possibility of there being a dissociation of drug effect on ketamine-induced changes in session-averaged versus event-related regional O2 signals, and raises caution in this context around interpretation of fMRI studies that do not measure behaviourally evoked effects.
Several factors were important to clarify before conducting the main ketamine reversal study, including confirming relationships between SRT task performance, ACC dependency, O2 signals and disruptor pharmacology. These were determined via independent experiments performed in separate cohorts of animals, thereby also allowing assessment of reproducibility of some of the main study findings. Muscimol inactivation of ACC prior to SRT testing in the first experiment led to slower and more variable RTs, confirming that ACC engagement is likely to be a requirement for optimal task performance. The contribution of ACC was further confirmed in the second and third experiments by recording tissue O2 signals during baseline performance. Transient event-related O2 signal increases were observed when animals completed a trial. These O2 level increases were delayed when animals failed to respond within the allocated time period, suggesting that ACC O2 signal increases may be linked to response production rather than simply presentation of preparatory/imperative cues. A more detailed analysis of completed trial-related O2 signals demonstrated that ACC O2 signals varied based on response speed, where the O2 signal increase was greatly delayed for the slowest versus fastest RT trials. The lack of direct correlation between reaction time and extracted O2 signal parameters might raise concern. However, it is well known that there is a temporal lag between neuronal activity and traditional BOLD responses on the order of seconds (Logothetis et al. 2001), and this same lag is likely to happen also with O2 signals. Such a temporal lag coupled with the need to average across several trials may confound attempts to correlate specific instances of amperometric O2 signals with behaviour. However, these findings are consistent with previous reports in primates and humans showing that lesions of this region produce response slowing in several tasks (Paus. 2001; Stuss et al. 2005; Shallice et al. 2008). Electrophysiological and imaging studies have also demonstrated a link between RT and ACC activation (Naito et al. 2000; Mulert et al. 2003; Drummond et al. 2005; Vallesi et al. 2012). Another noticeable aspect of the present study was that ACC O2 signals began to increase before imperative cue onset for the fastest RT trials, suggesting a potential role for ACC in alerting processes as defined by an ability to increase and maintain response readiness in preparation for an impending stimulus (Yanaka et al. 2010). Finally, muscimol infusion-induced RT slowing was accompanied by a significant increase in omitted trials, potentially implying a role of ACC in error detection and performance monitoring (Carter et al. 1998). The small and delayed O2 signals observed during omission trials may also be consistent with this idea, as their time course is in alignment with imperative cue offset, i.e., the time of potential error detection. Further studies with more systematic manipulation of task parameters would be necessary to disentangle those hypotheses though. It was clear, however, that engagement of ACC was required for efficient performance of the SRT task in rats.
Systemic administration of ketamine in all three experiments was shown to disrupt SRT task performance and event-related ACC O2 signals. Alterations of SRT task performance were qualitatively similar to those induced by muscimol inactivation, i.e., causing slower and more variable RTs. This response slowing was also reflected in the event-related ACC O2 signals which were significantly delayed. As such, RT increases induced by ketamine are potentially suggestive of the induction of deficits in processing speed. Concomitant with the effect of ketamine on SRT event-related O2 signals, it also produced a session-averaged, task-independent and robust increase in ACC O2 levels lasting for approximately 1 h post-administration, matching the known pharmacokinetic profile of the drug (Gastambide et al. 2013). Such increases in regional O2 signal have previously been attenuated by various classes of pharmacology including glutamatergic agents, as measured by BOLD phMRI in both rodents (Gozzi et al. 2008; Chin et al. 2011) and human volunteers (Doyle et al. 2013). A similar attenuation of the regional signal was also observed in the present study following pretreatment with 3 mg/kg of the mGlu2/3 receptor agonist LY379268. However and despite this reversal, LY379268 did not normalise ketamine-induced changes in SRT task performance or event-related O2 signals. The mGlu2/3 receptor agonist used actually tended to further increase reaction time and omitted trials, with respect to ketamine-treated but also vehicle-treated groups. These behavioural changes were associated with concomitant delays in task-related O2 signals and an increase in their amplitude. These findings therefore demonstrate the possibility of there being a dissociation of LY379268 effect on ketamine-induced changes in session-averaged versus event-related regional O2 signals. Such findings are all the more powerful from the fact that both session-averaged and event-related regional O2 signals were measured simultaneously from the same electrodes, implanted in the same behaving animals. There was no potential confound of signal differences arising from measurement of slightly different regions of tissue, as would have happened if independent electrodes or recording methodologies were utilised. Finally, it is important to mention that similarly to human BOLD fMRI, O2 amperometry indirectly measures a haemodynamic response driven by neurovascular coupling. Although we cannot rule out potential artefacts caused by direct effects of drugs under study on the vasculature itself, it does not seem likely that only vascular changes could explain both session-averaged and event-related regional O2 signal effects.
The results presented in this manuscript represent part of a larger, ongoing effort by our laboratory to understand to what degree in vivo O2 amperometry can offer a means to back-translate human fMRI findings to rodents, and where such translation seems to exist, what predictive utility modulation of these signals has for the discovery of novel treatments for neuropsychiatric disorders. The present results should be considered part of a feasibility assessment of the conduct of such studies, rather than a definitive statement on utility of the disruptor (ketamine) and pharmacological agents (mGlu2/3 receptor agonist) under question. Having said this, it would seem clear from the data already that it could be easy to over-interpret the significance of a drug-induced modulation of a resting-state BOLD ketamine signal.
NMDA receptor antagonists, and specifically ketamine administration, still remains one of the potentially most important and most useful tools for translational neuropsychiatric research. It is one of the few pharmacological tools that can easily be administered to both rodents and human volunteers to induce behavioural and cognitive disruption considered to be relevant to neuropsychiatric disorder, thereby potentially offering a means to index therapeutic utility of a novel agent much earlier during a clinical testing program. Ketamine administration has been studied mostly in the context of schizophrenia, where there has been debate regarding which aspects of the disease it may or may not model (Gilmour et al. 2012). Perhaps a more modern approach to the utility of ketamine-induced disruption is not to consider it all within a DSM/ICD-defined disease framework of schizophrenia, but rather to consider the profile of effects it has across cognitive domains and underlying circuitry (Insel et al. 2010; Insel. 2014). A more complete understanding of such constructs (as exemplified in the present study by ACC-dependent speed of processing measures) may then offer a more valuable indication of therapeutic utility. Future work here should build up a broader picture of drugs effects beyond the speed of processing construct into other relevant cognitive domains such as attention, working memory and executive function.
In conclusion, a consistent relationship was observed between reaction times during SRT performance and ACC O2 signal parameters, suggesting that the O2 amperometric signal is a valid correlate of task-induced regional engagement. Moreover, ketamine induced changes in both session-averaged, task-independent and regional task-dependent O2 signals similar to those obtained from previous human fMRI experiments, corroborating the use of real time in-vivo oxygen amperometry as a viable rodent surrogate of human BOLD fMRI. Finally, despite reducing session-averaged regional O2 increases induced by ketamine, the mGlu2/3 receptor agonist LY379268 failed to attenuate changes in SRT task performance and task-related O2 signals, raising questions about the degree to which such reversals of regional ketamine O2 signals can potentially be claimed to predict broader treatment efficacy.
References
Arrowsmith J, Miller P (2013) Trial watch: phase II and phase III attrition rates 2011–2012. Nat Rev Drug Discov 12:569. doi:10.1038/nrd4090
Baker S, Chin CL, Basso AM, Fox GB, Marek GJ, Day M (2012) Xanomeline modulation of the blood oxygenation level-dependent signal in awake rats: development of pharmacological magnetic resonance imaging as a translatable pharmacodynamic biomarker for central activity and dose selection. J Pharmacol Exp Ther 341:263–273. doi:10.1124/jpet.111.188797
Birkett P, Sigmundsson T, Sharma T, Toulopoulou T, Griffiths TD, Reveley A, Murray R (2007) Reaction time and sustained attention in schizophrenia and its genetic predisposition. Schizophr Res 95:76–85
Carter CS, Braver TS, Barch DM, Botvinick MM, Noll D, Cohen JD (1998) Anterior cingulate cortex, error detection, and the online monitoring of performance. Science 280:747–749
Cartmell J, Monn JA, Schoepp DD (1999) The metabotropic glutamate 2/3 receptor agonists LY354740 and LY379268 selectively attenuate phencyclidine versus d-amphetamine motor behaviors in rats. J Pharmacol Exp Ther 291:161–170
Chin CL, Upadhyay J, Marek GJ, Baker SJ, Zhang M, Mezler M, Fox GB, Day M (2011) Awake rat pharmacological magnetic resonance imaging as a translational pharmacodynamic biomarker: metabotropic glutamate 2/3 agonist modulation of ketamine-induced blood oxygenation level dependence signals. J Pharmacol Exp Ther 336:709–715. doi:10.1124/jpet.110.173880
Dickinson D, Ramsey ME, Gold JM (2007) Overlooking the obvious: a meta-analytic comparison of digit symbol coding tasks and other cognitive measures in schizophrenia. Arch Gen Psychiatry 64:532–542
Doyle OM, De Simoni S, Schwarz AJ, Brittain C, O’Daly OG, Williams SC, Mehta M, Doyle OM, De Simoni S, Schwarz AJ, Brittain C, O’Daly OG, Williams SC, Mehta MA (2013) Quantifying the attenuation of the ketamine pharmacological magnetic resonance imaging response in humans: a validation using antipsychotic and glutamatergic agents. J Pharmacol Exp Ther 345:151–160. doi:10.1124/jpet.112.201665
Drummond SP, Bischoff-Grethe A, Dinges DF, Ayalon L, Mednick SC, Meloy MJ (2005) The neural basis of the psychomotor vigilance task. Sleep 28:1059–1068
Francois J, Conway MW, Lowry JP, Tricklebank MD, Gilmour G (2012) Changes in reward-related signals in the rat nucleus accumbens measured by in vivo oxygen amperometry are consistent with fMRI BOLD responses in man. Neuroimage 60:2169–2181. doi:10.1016/j.neuroimage.2012.02.024
Francois J, Huxter J, Conway MW, Lowry JP, Tricklebank MD, Gilmour G (2014) Differential contributions of infralimbic prefrontal cortex and nucleus accumbens during reward-based learning and extinction. J Neurosci 34:596–607. doi:10.1523/JNEUROSCI.2346-13.2014
Gastambide F, Mitchell SN, Robbins TW, Tricklebank MD, Gilmour G (2013) Temporally distinct cognitive effects following acute administration of ketamine and phencyclidine in the rat. Eur Neuropsychopharmacol 23:1414–1422. doi:10.1016/j.euroneuro.2013.03.002
Gilmour G, Pioli EY, Dix SL, Smith JW, Conway MW, Jones WT, Loomis S, Mason R, Shahabi S, Tricklebank MD (2009) Diverse and often opposite behavioural effects of NMDA receptor antagonists in rats: implications for “NMDA antagonist modelling” of schizophrenia. Psychopharmacology (Berl) 205:203–216. doi:10.1007/s00213-009-1530-7
Gilmour G, Dix S, Fellini L, Gastambide F, Plath N, Steckler T, Talpos J, Tricklebank M (2012) NMDA receptors, cognition and schizophrenia—testing the validity of the NMDA receptor hypofunction hypothesis. Neuropharmacology 62:1401–1412. doi:10.1016/j.neuropharm.2011.03.015
Gozzi A, Large CH, Schwarz A, Bertani S, Crestan V, Bifone A (2008) Differential effects of antipsychotic and glutamatergic agents on the phMRI response to phencyclidine. Neuropsychopharmacology 33:1690–1703
Hitchman ML (1978) Measurement of dissolved oxygen. Wiley, Canada
Imre G, Salomons A, Jongsma M, Fokkema DS, Den Boer JA, Ter Horst GJ (2006) Effects of the mGluR2/3 agonist LY379268 on ketamine-evoked behaviours and neurochemical changes in the dentate gyrus of the rat. Pharmacol Biochem Behav 84:392–399
Insel TR (2014) The NIMH Research Domain Criteria (RDoC) project: precision medicine for psychiatry. Am J Psychiatry 171:395–397. doi:10.1176/appi.ajp.2014.14020138
Insel T, Cuthbert B, Garvey M, Heinssen R, Pine DS, Quinn K, Sanislow C, Wang P (2010) Research domain criteria (RDoC): toward a new classification framework for research on mental disorders. Am J Psychiatry 167:748–751. doi:10.1176/appi.ajp.2010.09091379
Krystal JH, Abi-Saab W, Perry E, D’Souza DC, Liu N, Gueorguieva R, McDougall L, Hunsberger T, Belger A, Levine L, Breier A (2005) Preliminary evidence of attenuation of the disruptive effects of the NMDA glutamate receptor antagonist, ketamine, on working memory by pretreatment with the group II metabotropic glutamate receptor agonist, LY354740, in healthy human subjects. Psychopharmacology (Berl) 179:303–309. doi:10.1007/s00213-004-1982-8
Li J, Ishiwari K, Conway MW, Francois J, Huxter J, Lowry JP, Schwarz AJ, Tricklebank M, Gilmour G (2014) Dissociable effects of antipsychotics on ketamine-induced changes in regional oxygenation and inter-regional coherence of low frequency oxygen fluctuations in the rat. Neuropsychopharmacology 39:1635–1644. doi:10.1038/npp.2014.10
Logothetis NK, Pauls J, Augath M, Trinath T, Oeltermann A (2001) Neurophysiological investigation of the basis of the fMRI signal. Nature 412:150–157. doi:10.1038/35084005
Lowry JP, Boutelle MG, Fillenz M (1997) Measurement of brain tissue oxygen at a carbon past electrode can serve as an index of increases in regional cerebral blood flow. J Neurosci Methods 71:177–182
McHugh SB, Fillenz M, Lowry JP, Rawlins JN, Bannerman DM (2011) Brain tissue oxygen amperometry in behaving rats demonstrates functional dissociation of dorsal and ventral hippocampus during spatial processing and anxiety. Eur J Neurosci 33:322–337. doi:10.1111/j.1460-9568.2010.07497.x
Moghaddam B, Adams BW (1998) Reversal of phencyclidine effects by a group II metabotropic glutamate receptor agonist in rats. Science 281:1349–1352
Morgan P, Van Der Graaf PH, Arrowsmith J, Feltner DE, Drummond KS, Wegner CD, Street SD (2012) Can the flow of medicines be improved? Fundamental pharmacokinetic and pharmacological principles toward improving Phase II survival. Drug Discov Today 17:419–424. doi:10.1016/j.drudis.2011.12.020
Mulert C, Gallinat J, Dorn H, Herrmann WM, Winterer G (2003) The relationship between reaction time, error rate and anterior cingulate cortex activity. Int J Psychophysiol 47:175–183
Naito E, Kinomura S, Geyer S, Kawashima R, Roland PE, Zilles K (2000) Fast reaction to different sensory modalities activates common fields in the motor areas, but the anterior cingulate cortex is involved in the speed of reaction. J Neurophysiol 83:1701–1709
Ngan ET, Liddle PF (2000) Reaction time, symptom profiles and course of illness in schizophrenia. Schizophr Res 46:195–201
Paus T (2001) Primate anterior cingulate cortex: where motor control, drive and cognition interface. Nat Rev Neurosci 2:417–424. doi:10.1038/35077500
Pitsikas N, Markou A (2014) The metabotropic glutamate 2/3 receptor agonist LY379268 counteracted ketamine-and apomorphine-induced performance deficits in the object recognition task, but not object location task, in rats. Neuropharmacology 85:27–35. doi:10.1016/j.neuropharm.2014.05.008
Ragozzino ME, Rozman S (2007) The effect of rat anterior cingulate inactivation on cognitive flexibility. Behav Neurosci 121:698–706
Rorick-Kehn LM, Johnson BG, Knitowski KM, Salhoff CR, Witkin JM, Perry KW, Griffey KI, Tizzano JP, Monn JA, McKinzie DL, Schoepp DD (2007) In vivo pharmacological characterization of the structurally novel, potent, selective mGlu2/3 receptor agonist LY404039 in animal models of psychiatric disorders. Psychopharmacology (Berl) 193:121–136. doi:10.1007/s00213-007-0758-3
Shallice T, Stuss DT, Picton TW, Alexander MP, Gillingham S (2008) Multiple effects of prefrontal lesions on task-switching. Front Hum Neurosci 1:2. doi:10.3389/neuro.09.002.2007
Stuss DT, Alexander MP, Shallice T, Picton TW, Binns MA, Macdonald R, Borowiec A, Katz DI (2005) Multiple frontal systems controlling response speed. Neuropsychologia 43:396–417
Vallesi A, McIntosh AR, Crescentini C, Stuss DT (2012) fMRI investigation of speed-accuracy strategy switching. Hum Brain Mapp 33:1677–1688. doi:10.1002/hbm.21312
Vinogradov S, Poole JH, Willis-Shore J, Ober BA, Shenaut GK (1998) Slower and more variable reaction times in schizophrenia: what do they signify? Schizophr Res 32:183–190
Yanaka HT, Saito DN, Uchiyama Y, Sadato N (2010) Neural substrates of phasic alertness: a functional magnetic resonance imaging study. Neurosci Res 68:51–58. doi:10.1016/j.neures.2010.05.005
Financial disclosure
Jennifer Francois, Francois Gastambide, Michael Warwick Conway, Mark Tricklebank and Gary Gilmour declare being employees of Eli Lilly & Co Ltd. The research leading to these results has received support from the Innovative Medicines Initiative Joint Undertaking under grant agreement no. 115008 of which resources are composed of EFPIA in-kind contribution and financial contribution from the European Union’s Seventh Framework Programme (FP7/2007-2013).
Author information
Authors and Affiliations
Corresponding author
Additional information
Jennifer Francois and Francois Gastambide contributed equally to this work.
Electronic supplementary material
Below is the link to the electronic supplementary material.
ESM 1
(DOC 792 kb)
Rights and permissions
About this article
Cite this article
Francois, J., Gastambide, F., Conway, M.W. et al. Dissociation of mGlu2/3 agonist effects on ketamine-induced regional and event-related oxygen signals. Psychopharmacology 232, 4219–4229 (2015). https://doi.org/10.1007/s00213-015-3948-4
Received:
Accepted:
Published:
Issue Date:
DOI: https://doi.org/10.1007/s00213-015-3948-4