Abstract
A common approach to cancer therapy is the combination of a natural product with chemotherapy to overcome sustained cell proliferation and chemotherapy resistance obstacles. Diosgenin (DG) is a phytosteroidal saponin that is naturally present in a vast number of plants and has been shown to exert anti-cancer activities against several tumor cells. Herein, we assessed the chemo-modulatory effects of DG on volasertib (Vola) as a polo-like kinase 1 (PLK1) inhibitor and doxorubicin (DOX) in hepatocellular carcinoma (HCC) cell lines. DOX and Vola were applied to two human HCC cell lines (HepG2 and Huh-7) alone or in combination with DG. The cell viability was determined, and gene expressions of PLK1, PCNA, P53, caspase-3, and PARP1 were evaluated by RT-qPCR. Moreover, apoptosis induction was determined by measuring active caspase-3 level using ELISA method. DG enhanced the anticancer effects of Vola and DOX. Moreover, DG enhanced Vola- and DOX-induced cell death by downregulating the expressions of PLK1 and PCNA, elevating the expressions of P53 and active caspase-3. DG showed promising chemo-modulatory effects to Vola and DOX against HCC that may be attributed partly to the downregulation of PLK1 and PCNA, upregulation of tumor suppressor protein P53, and apoptosis induction. Thus, DG combination with chemotherapy may be a promising treatment approach for HCC.
Similar content being viewed by others
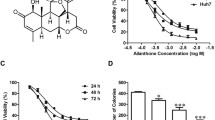
Avoid common mistakes on your manuscript.
Introduction
Hepatocellular carcinoma (HCC) is ranked as the fifth most prevalent malignancy and the third principal cause of cancer related mortality worldwide (Hsu et al. 2015). HCC is commonly diagnosed at an advanced stage because of the obstacle in the precise and reliable diagnosis of HCC at early-stage (Bashir et al. 2020). Strategies for treatment such as liver transplantation, surgery, and local ablative therapy are inappropriate for advanced HCC cases (Yoon and Choi 2023). Chemotherapy, despite being one of the most essential treatment modalities for advanced HCC (Ikeda et al. 2017), may have an only palliative effect (Brito et al. 2012). Systemic chemotherapy failure is mainly returned to excessive intrinsic and acquired drug resistance of HCC (Lin et al. 2012; Fan et al. 2022). Systemic doxorubicin (DOX) as the most broadly used agent in clinical practice provides a partial response in only less than 10% of patients, without improving overall survival (Brito et al. 2012). Poor bioavailability and multidrug resistance hinder the use of single-drug chemotherapy, and high drug dose may be the key to effective therapy. However, the high dose of chemotherapy is restricted by severe adverse side effects (Wakharde et al. 2018). Combining chemotherapy with a natural product has been extensively developed since it can increase therapeutic efficacy while avoiding unacceptable side effects as a result of lowering chemotherapy dose (Cao et al. 2016; Yousef et al. 2023). All of these make it crucial to find out chemosensitizers which can achieve more tumoricidal efficiency against HCC without elevating the dose of chemotherapy.
Polo-like kinase 1 (PLK1), a ubiquitously expressed serine/threonine protein kinase, is extensively recognized as an oncogene that drives cell proliferation by inducing mitosis and cytokinesis. It is commonly considered as a tumor biomarker, as excessive PLK1 expression has been related to poor prognosis, radioresistance, and chemo resistance in numerous types of cancer (Yousef et al. 2020). PLK1 can inhibit pro-caspase-8 processing upon Fas stimulation by direct phosphorylation at S305. When PLK1 is repressed, pro-caspase-8 is processed and caspase-3 is activated, which in turn leads to apoptosis (Wu et al. 2019). Meanwhile, the constitutive expression of PLK1 has been found in cancerous liver cells but not in healthy liver tissues (Sun et al. 2014). According to previous published work, patients with HCC have a lower chance of survival when PLK1 downstream effector proteins such Myc, BUBR1, and Akt are overexpressed in cancer cells (Jang et al. 2012; Chen et al. 2017). Additionally, the inhibition of PLK1 might sensitize several cancer cells such as esophageal squamous cell carcinoma, pancreatic cancer, Ewing sarcoma cells, and HCC to chemotherapy (Li et al. 2016, Weiß et al. 2016, Li et al. 2019a, Wu et al. 2019, Yousef et al. 2020).
Diosgenin (DG), a phytosteroidal saponin extracted from a wide number of plants such as fenugreek (Iranmanesh et al. 2018; Rohani et al. 2019), has been traditionally used for the treatment of inflammation, vascular calcification, thrombosis, atherosclerosis, acute lung injury, diabetes, hypercholesterolemia, neurodegeneration, and gastrointestinal disorders (Li et al. 2010; Cai et al. 2018; Chen et al. 2018b; Gong et al. 2019). Several studies have proved the promising anticancer activities of DG (Binesh et al. 2018; Chen et al. 2018b; Peng et al. 2023; Ruan et al. 2023). The anticancer activities of DG has been detected in several cancer origins such as breast cancer (Khanal et al. 2022), colorectal cancer (Li et al. 2021), osteosarcoma (Huang et al. 2019), gastric cancer (Gu et al. 2021), HCC (da Silva et al. 2022), and prostate cancer (Peng et al. 2023). However, the chemosensitizing activity of DG in HCC and the underlying mechanism of action are needed to be elucidated. Thus, this study aimed to investigate the ability of DG to sensitize HepG2 and Huh-7 cells to DOX- and Vola-induced cell death alongside unravelling its mechanism of action.
Materials and methods
Research work was agreed by the Mansoura University’s ethics committee (Ethics code: 2023–83).
Cell lines and culture
HepG2 and Huh-7 human HCC cell lines used in this study were obtained from VACSERA, Dokki, Giza, Egypt. The cells were cultured in Dulbecco’s modified Eagle’s medium (Lonza, Vervies, Belgium) containing 10% fetal bovine serum (Seralab, Brazil) and 1% 100 U/mL penicillin/streptomycin solution (Lonza, Vervies, Belgium) and incubated in a humidified atmosphere containing 5% CO2 at 37 °C.
Reagents and materials
DG and Vola (BI-6727) were bought from Cayman (Ann Arbor, MI, USA). DOX vial (10 mg/5ml) was a market-available pharmaceutical product. Dimethyl sulfoxide (DMSO) was purchased from Elgomhoria company (Mansoura, Egypt) and used as a vehicle for preparing DG and Vola solutions.
Cell viability assay
In 96-well tissue culture plate, 2 × 104 HepG2 or Huh-7 cells were seeded in each well. The next day, cells were exposed to DOX concentrations (10–1.25 µM), Vola concentration (10–1.25 µM), or DG concentrations (100–6.25 µM) in triplicates. The crystal violet assay was used in the next day to determine cell viability (El-Mesery et al. 2013). The optical density (OD) was measured at an absorbance wavelength of 570 nm using plate reader. The data were presented as the cell viability percentage of each group as compared to the control group of untreated cells (100% viability). The following formula was used to calculate cell viabilities:
Analyses of synergy
In experiments, where DG was used as a chemosensitizer, 10 µM of DG (Chen et al. 2011) was added to the cells 30 min after DOX (0.625–10 μM) and Vola (0.625–10 μM). Then, cell viability was assessed as described previously.
IC30 of DG, DOX, and Vola to achieve a 30% growth inhibition in combination and of the individual drugs were calculated by nonlinear regression analysis using the excel software based on the dose–response curves. Finally, interactions on combining DG with either DOX or Vola were investigated and the combined growth-inhibitory effect at 30% cell death was assessed by calculating the combination index (CI). The CI was defined by
\(\mathrm{CI }= ({\text{dA}}/{\text{D}}30{\text{A}}) + ({\text{dB}}/{\text{D}}30{\text{B}})\), where D30A is the concentration of drug A (DG) necessary to cause 30% cell death, and dA is the drug A concentration which will produce 30% cell death in combination with drug B(chemotherapy). Likewise, D30B is the drug B concentration necessary to cause 30% cell death, and dB is the drug B concentration which will produce 30% cell death in combination with drug A (Yamashita et al. 2016; Nishi et al. 2023). The combination effect was symbolized as follows: CI < 1, synergistic effect; CI = 1, additive effect, and CI > 1, antagonistic effect (Sarkar et al. 2011; Morisaki et al. 2013).
Real-time reverse transcription-PCR (qRT-PCR)
In 6-well plate, 1 × 106 cells of HepG2 or Huh-7cells were seeded in each well and treated with vola (1 µM), DOX (5 µM), and/or DG (10 µM) alone or in combination for 24 h. Subsequently, total RNA was isolated using TRIZOL (Zymoresearch Co., Murphy Ave. Irvine, CA92614, USA) and was purified using the Direct-zol RNA miniPREP kit (Zymoresearch Co., Murphy Ave. Irvine, CA92614, U.S.A.). Samples (10 µl) of total RNA was reverse transcripted to cDNA using the sensiFASTcDNA synthesis kit (bioline Inc., Taunton, USA). Primers were designed according to gene sequences obtained from PubMed (Entrez Gene) and ordered from Invitrogen (CA, USA.). The Applied Biosystems 7500 Fast Real-Time PCR System (Applied Biosystems, Woburn, MA, USA) was used to conduct the PCR using the SYBR Green master mix (Bioline Inc., Taunton, USA). In accordance with the manufacturer’s recommendations, the thermal cycling program was conducted. Using the 2−ΔΔCT method, the relative expression of the genes under study was calculated. The GAPDH gene was used as a reference gene to quantify expression changes in relation to its level of expression. Primer sequences are as previously described (Yousef et al. 2020).
Active caspase-3 concentration determination
In 6-well plate, 1 × 106 cells of HepG2 or Huh-7cells were seeded and exposed to Vola (1 µM), DOX (5 µM), and/or DG (10 µM) alone or in combination for 24 h. Subsequently, cells were collected, washed two times using cold PBS, resuspended in protein lysis buffer, and kept for 30 min on ice. Later, centrifugation was conducted for 10 min at 1500 rpm. Cell lysates (100 μL) have been transferred to 96-well plates in triplicates and kept for 2 h at 37 °C. Concentration of active caspase-3 in HepG2 and Huh-7 cells lysates was measured through the Invitrogen ELISA Kit (CA, U.S.A.) based on the manufacturer's instruction.
Statistical analysis
The standard error of the mean (SEM) was used to represent the data. The SPSS software version 16.0 (SPSS, Inc., Chicago, IL, USA) was used to calculate statistical significance differences between samples using a one-way analysis of variance (ANOVA) followed by a Tukey’s post hoc test or Student’s t-test. The GraphPad Prism (GraphPad Software Inc., San Diego, USA) was used to create the graphs. The threshold for statistical significance predefined as p ≤ 0.05.
Results
DOX, Vola, and DG induce cell death in HepG2 and Huh-7 cell lines
DG is a well-known phytosteroidal saponin that exert antitumor activity in various tumor types. Consequently, its impact to provoke death in HepG2 and Huh-7 cells was investigated. Cells were exposed to the prementioned concentration of DG, and the viability of cell was assessed the next day. As demonstrated in Fig. 1, DG had the capability of inducing significant cell death. According to our results, DG induced death of cells in a dose-dependent manner with an IC30 of 51.63 µM and 65 µM in HepG2 and Huh-7 cells, respectively (Table 1).
Furthermore, Vola, a specific PLK1 inhibitor, was assessed compared with DOX for the inhibition of HCC cell viability. Cell viability was assessed in the next day after treating cells with a series of concentrations (10–0.625 M) of DOX and (10–0.625 M) Vola. DOX repressed cell viability in a dose dependent manner with an IC30 of 3.67 μM and 6.89 μM in HepG2 and in Huh-7 cells, respectively. Also, Vola decreased cell viability in a dose-dependent manner with an IC30 of 3.16 μM and 6.23 μM in HepG2 and Huh-7 cells, respectively (Figure 1).
The inhibitory effect of DG, DOX, and Vola on cell viability in HepG2 and Huh-7 cells. Cells were seeded in 96-well plates, and after 24 h; they were treated for 24 h with the indicated concentrations of DG (100–6.25 M) (a). In 96-well plates, cells were seeded and later treated for 24 h with the indicated concentrations of DOX (10–0.625 µM) (b). In 96-well plates, cells were seeded and incubated for 24 h with the indicated concentrations of Vola (10–0.625 µM) (c). Cell viability was assessed using crystal violet. ***P < 0.001, *P < 0.05 compared to the control group. DG, diosgenin; DOX, doxorubicin; Vola, volasertib
DG significantly enhances DOX and Vola cytotoxicity in HepG2 and Huh-7 cells
The effect of DG on DOX and Vola cytotoxicity was investigated. HepG2 and Huh-7 cell treatments with either DG/DOX or DG/Vola for 24 h induced suppression of the cell viability in a dose-dependent manner. The addition of 10 µM of DG decreased IC30 for DOX and Vola to 2.78 µM and 1.76 µM in HepG2 cells, respectively, and to 4.69 µM and 3.35 µM in Huh-7 cells, respectively (Figure 2).
The capability of these molecules to cause additive or synergistic inhibition was determined using ACI approach. When DG (10 µM) was added to cells incubated with ascending doses of DOX or Vola, the combinational impact improved the inhibition of cell growth in comparison with single drug treatments in both HepG2 and Huh-7 cells. In addition, CI values at IC30 indicated a synergism between DG and either DOX or Vola (Table 2).
DG enhances DOX and Vola cytotoxicity in HepG2 and Huh-7 cells. In 96-well plates, cells were seeded and incubated with DG (10 µM) in combination with ascending doses of vola (0.625–10 μM) or DOX (0.625–10 μM) for 24 h. Cell viability was assessed by crystal violet. #p < 0.05 compared to DOX, $$$p < 0.001, and $p < 0.05 compared to Vola. DOX, doxorubicin; Vola, volasertib
DG potentiates downregulation of PLK1 mRNA expression in HepG2 and Huh-7 cells
Following HCC cell treatments with DG, DOX, Vola, DG/DOX, and DG/Vola for 24 h, DG/DOX revealed a significant reduction in the expression of PLK1 mRNA by 2.75-fold (p < 0.00.1) and 1.65-fold (p < 0.05) as compared to cells treated with DOX in HepG2 and Huh-7 cells, respectively (Figure 3).
DG significantly decreases PLK1 mRNA expression caused by Vola and DOX in HepG2 and Huh-7 cells. In 6-well plates, cells were seeded and incubated with vola (1 µM), DOX (5 µM), and/or DG (10 µM) alone or in combination for 24 h. Effect of DG, DOX, Vola, DG/DOX, and DG/Vola on mRNA expression level of PLK1 was measured by RT-PCR technique. Bars symbolize mean ± SEM. ***p < 0.001, *p < 0.05 compared to the control group, ###p < 0.001 and #p < 0.05 compared to DOX. DG, diosgenin; DOX, doxorubicin; PLK1, polo-like kinase 1; Vola, volasertib
DG potentiates downregulation of PCNA and upregulation of p53, caspase-3, and PARP1 mRNA expression induced by DOX and Vola in HepG2 and Huh-7 cells
To investigate the molecular mechanism of action of DG, mRNA expression of PCNA, p53, PARP1, and caspase-3 were assessed in Huh-7 and HepG2 cells using RT-qPCR.
Interestingly, DG/DOX revealed a significant reduction in PCNA expression by 3.08-fold (p ≤ 0.001) in HepG2 cells, in comparison with cells treated with DOX which came in agreement with the suppression in cell viability when cells were treated with combined treatment (Figure 4a).
Moreover, DG/DOX and DG/Vola demonstrated a significant increase in p53 expression by 1.37-fold (p < 0.05) and 2-fold (p < 0.001) in comparison with cells treated with DOX and Vola, respectively in HepG2 cells (Figure 4b).
As shown in Fig. 4c, DG/Vola showed 2.36-fold (p ≤ 0.001) significant rise in caspase-3 mRNA expression in comparison with Vola in HepG2 cells. Moreover, DG/DOX and DG/Vola showed significant increase in caspase-3 gene expression by 1.98-fold and 1.6-fold (p ≤ 0.001) as compared with DOX and Vola in Huh-7 cells, respectively.
DG/DOX showed 1.2-fold (p ≤ 0.05) and 1.24-fold (p ≤ 0.05) significant rise in mRNA expression of PARP1 in comparison with DOX in HepG2 and Huh-7 cells, respectively, which came in agreement with the decrease in cell viability when cells were treated with the combined treatment (Figure 4d).
DG potentiates downregulation of mRNA expression of PCNA and upregulation of p53, caspase-3 and PARP1 provoked by DOX and Vola in HepG2 and Huh-7cells. In 6-well plates, cells were seeded and incubated with vola (1 µM), DOX (5 µM), and/or DG (10 µM) alone or in combination for 24 h. Effects of DG, DOX, Vola, DG/DOX, and DG/Vola on mRNA expression level of PCNA, p53, caspase-3, and PARP1 were assessed using RT-PCR technique. Bars symbolize mean ± SEM. ***p < 0.001, *p < 0.05 compared to the control group, $$$p < 0.001compared to Vola, ###p < 0.001 and #p < 0.05 compared with DOX. DG, diosgenin; DOX, doxorubicin; PARP1, poly [ADP-ribose] polymerase 1; PCNA, proliferating cell nuclear antigen; Vola, volasertib
DG increases active caspase-3 concentration caused by DOX and Vola in HepG2 and Huh-7 cells
Ultimately, apoptosis was evaluated by measuring the concentration of active caspase-3 in lysates of HepG2 and Huh-7 cells. According to our results, there is an increase in the concentration of active caspase-3 in HepG2 cells treated with DG/DOX and DG/Vola by 31.46% and 127.66% as compared with cells treated with DOX and Vola, respectively. In addition, Huh-7 cells treated with DG/DOX and DG/Vola demonstrated a rise in the concentration of active caspase-3 by 35.65% and 121.33% in comparison with cells treated with DOX and Vola, respectively, that came in agreement with the decrease in cell viability when cells were treated with the combined treatment (Fig. 5).
DG increases active caspase-3 concentration caused by Vola and DOX in HepG2 and Huh-7 cells. The impact of 10 µM DG alone or combined with either 5 µM DOX or 1 µM Vola on active caspase-3 concentration for 24 h in the lysates of HepG2 and Huh-7 cells was assessed by ELISA. Bars symbolize mean ± SEM. ***p < 0.001 compared to control group, $$$p < 0.001compared to Vola, ###p < 0.001compared to DOX. DG, diosgenin; DOX, doxorubicin; Vola, volasertib
Downregulation of PLK1 induced by DG alone or in combination with DOX and Vola correlates with apoptosis induction and consequent cell death
Whereas level of PLK1 was significantly high in the HCC cells, it was interesting to explore how PLK1 correlated with either cell proliferative marker such as PCNA or apoptotic markers such as p53 and active caspase-3 in HepG2 and Huh-7 cells.
According to statistical analysis, PLK1 is inversely correlated with active caspase-3 concentration (r = −0.74, p ≤ 0.001) (Fig. 6a) and p53 (r = −0.67, p ≤ 0.001) (Fig. 6b). Contrary, PLK1 is directly correlated with PCNA (r = 0.73, p ≤ 0.001) (Fig. 6c) in HepG2 cell line.
Furthermore, PLK1 is inversely correlated with active caspase-3 concentration (r = −0.76, p ≤ 0.001) (Fig. 6d) and p53 (r = −0.87, p ≤ 0.001) (Fig. 6e). Contrary, PLK1 is directly correlated with PCNA (r = 0.7, p ≤ 0.001) (Fig. 6f) in Huh-7 cell line illustrating the role of PLK1 inhibition in activating caspase cascade and inducing apoptosis.
Correlation of PLK1 with markers of apoptosis and proliferation of cell in the HepG2 and Huh-7 cell lines. The expression of PLK1 correlated with active caspase-3 concentration, p53 mRNA expression, and cell proliferation in the HepG2 and Huh-7 cell lines either untreated or treated with DG, DOX, Vola, DOX/DG, or Vola/DG.
Discussion
Cell proliferation is considered a critical biological process to all living organisms owing to its role in the growth and maintaining tissue homeostasis (Bologna-Molina et al. 2013). The imbalance between cell proliferation and apoptosis is a pivotal mechanism for tumorigenesis and cancer progression that can directly affect the biological cancer activities (Fang et al. 2019; Morana et al. 2022). Alterations in p53 expression and/or activation are common in HCC cells, which participate in their resistance to chemotherapy (Brito et al. 2012). DOX has been extensively used as the front-line chemotherapeutic agent in HCC (Fan et al. 2017). DOX resistance is one of the major reasons for chemotherapy failure in the clinic (Chen et al. 2018a). Since the outcome of chemotherapy treatment for HCC patients is not satisfactory, it is critical to develop chemosensitizer to improve therapeutic efficiency. Previous studies have shown that DG could sensitize cancer cells to chemotherapy (Li et al. 2010; Lepage et al. 2011; Sethi et al. 2018).
PLK1, a key regulator of the cell cycle, is reported to be implicated in HCC tumorigenesis, metastasis, and drug resistance (Pellegrino et al. 2010; Sun et al. 2014; Liu et al. 2017; Mao et al. 2018). Its overexpression is a common feature of human cancers and its expression correlates often with poor prognosis (Louwen and Yuan 2013). Moreover, PLK1 inhibition can suppress cancer proliferation, reverse the cancer cells drug resistance, and enhance sensitivity to radiotherapy and chemotherapy (Liu et al. 2017). PLK1 activation is based on the catalytic activity of Akt downstream of phosphatidylinositol 3-kinase (PI3K) (Kasahara et al. 2013). Inhibition of PI3K and Akt significantly downregulated PLK1 and induce apoptosis (Kasahara et al. 2013; Mao et al. 2016). DG is also reported to suppress PI3K/Akt activation in several cell types such as prostate cancer, and HCC (Chen et al. 2011; Li et al. 2015). Furthermore, recent research has shown that blocking PI3K/AKT/mTOR signaling made cancer cells more susceptible to chemotherapeutics (Li et al. 2019b). This was supported in our study as DG treated alone significantly decreased the viability of HCC cells at the doses of 50 and 100 μΜ, which was consist with previous studies (Chen et al. 2018b; Yu et al. 2018). Furthermore, combined treatment of DG with DOX or Vola improved their cytotoxicity in HCC cells, suggesting that DG has the capability of potentiating DOX and Vola anti-tumor activity. Also, DOX and Vola decreased the PLK1 expression, which was increased in the presence of DG (Figure 3).
Proliferating cell nuclear antigen (PCNA), a critical regulator of the cell cycle, is a 36-kDa molecule, which is highly conserved among species (Khalil et al. 2015). The PCNA expression is elevated during G1 phase through S phase of the cell cycle, and it plays an essential role in regulating DNA replication (McKay et al. 2002). Therefore, PCNA was found valuable in the study of the proliferative activity in various tumors including HCC (Loor et al. 1997; Murlidhar and Goswami 2012). Also, DG attenuated tumor cell proliferation via lowering the PCNA index in colon tumors (Malisetty et al. 2005). Likewise, in our study, DG enhanced the antiproliferative activity of DOX and Vola by increasing the suppression of PCNA expression (Figure 4).
Apoptosis is a programmed cell death that is important for normal development, host defense, and oncogenesis suppression (Lee et al. 2023). Apoptosis dysregulation is a crucial component of tumor pathogenesis and is widely believed to be a defining feature of the majority types of tumor (Yao et al. 2019). Therefore, promoting apoptosis in cancer cells may have a vital role in cancer therapy (Alimbetov et al. 2018). p53 is a tumor suppressor gene that can induce apoptosis by intrinsic and extrinsic pathways (Yogosawa and Yoshida 2018; Zhou et al. 2019). Moreover, p53 is a vital PLK1 target, and its function is abrogated through physical interaction with PLK1 (Gutteridge et al. 2016). Indeed, p53 downregulation and PLK1 overexpression make cancer cells resistant to chemotherapeutic treatment. As a result, we explored the molecular mechanisms underlying the sensitivity of tumor cells to DG combinations with DOX or Vola by measuring p53, caspase-3, PARP1 mRNA levels, and active caspase-3 concentration. Accroding to our data, DG enhanced the expression of p53 induced by either DOX or Vola, which resulted in caspase 3 activation in HCC cells (Figures 4 and 5).
To investigate the impact of PLK1 expression on tumor proliferation and apoptosis, we performed correlation studies between PLK1 and each of active caspase-3 concentration, p53 and PCNA expressions in HepG2 and Huh-7 cells (Figure 6). According to our results, there was a positive correlation between PLK1 and PCNA expression in both HepG2 and Huh-7. This positive correlation indicates that PLK1 plays a significant role in promoting tumor proliferation in HepG2 and Huh-7 cell lines. In addition, PLK1 inhibition significantly correlated with apoptosis activation as shown by the negative correlation between PLK1 and both of p53 expression and active caspase-3 concentration in both HepG2 and Huh-7.
Thus, conclusively, this study demonstrates that combination of DG with DOX or Vola enhances their antitumor effects in HCC cell lines, by decreasing PLK1 and PCNA expressions and increasing p53 expression. Furthermore, this study provides a novel insight into a prospective combination strategy for HCC treatment by combining DG with chemotherapeutics currently in use (Figure 7).
Data availability
Data are available upon request.
Abbreviations
- ANOVA:
-
One-way analysis of variance
- cDNA:
-
Complementary DNA
- Ct:
-
Threshold cycle
- PLK1:
-
Polo-like kinase 1
- PCNA:
-
Proliferating cell nuclear antigen
- ELISA:
-
Enzyme-linked immunosorbent assay
- GAPDH:
-
Glyceraldehyde 3-phosphate dehydrogenase
- HCC:
-
Hepatocellular carcinoma
- LC:
-
Liver cirrhosis
- DG:
-
Diosgenin
- DOX:
-
Doxorubicin
- Vola:
-
Volasertib
- qPCR:
-
Quantitative polymerase chain reaction
- SEM:
-
Standard error of the mean
References
Alimbetov D, Askarova S, Umbayev B, Davis T, Kipling D (2018) Pharmacological targeting of cell cycle, apoptotic and cell adhesion signaling pathways implicated in chemoresistance of cancer cells. Int J Mol Sci 19:1690–1721
Bashir AO, El-Mesery ME, Anwer R, Eissa LA (2020) Thymoquinone potentiates miR-16 and miR-375 expressions in hepatocellular carcinoma. Life Sci 254:117794
Binesh A, Devaraj SN, Devaraj H (2018) Inhibition of nuclear translocation of notch intracellular domain (NICD) by diosgenin prevented atherosclerotic disease progression. Biochimie 148:63–71
Bologna-Molina R, Mosqueda-Taylor A, Molina-Frechero N, Mori-Estevez AD, Sánchez-Acuña G (2013) Comparison of the value of PCNA and Ki-67 as markers of cell proliferation in ameloblastic tumor. Medicina Oral, Patologia Oral y Cirugia Bucal 18:e174
Brito A, Abrantes A, Pinto-Costa C, Gomes A, Mamede A, Casalta-Lopes J, Gonçalves A, Sarmento-Ribeiro A, Tralhão J, Botelho M (2012) Hepatocellular carcinoma and chemotherapy: the role of p53. Chemotherapy 58:381–386
Cai B, Seong K-J, Bae S-W, Chun C, Kim W-J, Jung J-Y (2018) A synthetic diosgenin primary amine derivative attenuates LPS-stimulated inflammation via inhibition of NF-κB and JNK MAPK signaling in microglial BV2 cells. Int Immunopharmacol 61:204–214
Cao J, Han J, Xiao H, Qiao J, Han M (2016) Effect of tea polyphenol compounds on anticancer drugs in terms of anti-tumor activity, toxicology, and pharmacokinetics. Nutr 8:762
Chen P-S, Shih Y-W, Huang H-C, Cheng H-W (2011) Diosgenin, a steroidal saponin, inhibits migration and invasion of human prostate cancer PC-3 cells by reducing matrix metalloproteinases expression. PLoS ONE 6:e20164
Chen YL, Chen PM, Ming YZ, Lin PY, Chu CP, Chu PY (2017) Phosphorylated AKT expression in tumor-adjacent normal tissue is associated with poor prognosis in patients with hepatocellular carcinoma. Oncol Lett 14:7461–7466
Chen Z, Xu J, Wu Y, Lei S, Liu H, Meng Q, Xia Z (2018b) Diosgenin inhibited the expression of TAZ in hepatocellular carcinoma. Biochem Biophys Res Commun 503:1181–1185
Chen L, Zeng Y, Zhou S-F (2018a) Role of apoptosis in cancer resistance to chemotherapy. In: Yusuf T (ed) Current Understanding of Apoptosis. IntechOpen, Rijeka, Ch. 7, pp 125–136
Coghi P, Yaremenko IA, Prommana P, Wu JN, Zhang RL, Ng JP, Belyakova YY, Law BYK, Radulov PS, Uthaipibull C (2022) Antimalarial and anticancer activity evaluation of bridged ozonides, aminoperoxides, and tetraoxanes. ChemMedChem 17:e202200328
da Silva MF, de Lima LVA, Zanetti TA, Felicidade I, Favaron PO, Lepri SR, Rondina DBL, Mantovani MS (2022) Diosgenin increases BBC3 expression in HepG2/C3A cells and alters cell communication in a 3D spheroid model. Mutation Research/genetic Toxicology and Environmental Mutagenesis 879:503512
El-Mesery M, Trebing J, Schäfer V, Weisenberger D, Siegmund D, Wajant H (2013) CD40-directed scFv-TRAIL fusion proteins induce CD40-restricted tumor cell death and activate dendritic cells. Cell Death Dis 4:e916
Fan Y-P, Liao J-Z, Lu Y-Q, Tian D-A, Ye F, Zhao P-X, Xiang G-Y, Tang W-X, He X-X (2017) MiR-375 and doxorubicin co-delivered by liposomes for combination therapy of hepatocellular carcinoma. Mol Ther Nucleic Acids 7:181–189
Fan Y, Xue H, Zheng H (2022) Systemic therapy for hepatocellular carcinoma: current updates and outlook. J Hepatocell Carcinoma 9:233–263
Fang H, Jiang W, Jing Z, Mu X, Xiong Z (2019) miR-937 regulates the proliferation and apoptosis via targeting APAF1 in breast cancer. Onco Targets Ther 12:5687–5699
Gong N, Wang Y, Zhang B, Yang D, Du G, Lu Y (2019) Screening, preparation and characterization of diosgenin versatile solvates. Steroids 143:18–24
Gu L, Zheng H, Zhao R, Zhang X, Wang Q (2021) Diosgenin inhibits the proliferation of gastric cancer cells via inducing mesoderm posterior 1 down-regulation-mediated alternative reading frame expression. Hum Exp Toxicol 40:S632-s645
Gutteridge REA, Ndiaye MA, Liu X, Ahmad N (2016) Plk1 inhibitors in cancer therapy: from laboratory to clinics. Mol Cancer Ther 15:1427–1435
Hsu F-T, Liu Y-C, Liu T-T, Hwang J-J (2015) Curcumin sensitizes hepatocellular carcinoma cells to radiation via suppression of radiation-induced NF-κB activity. BioMed Res Int 2015:363671
Huang H, Nie C, Qin X, Zhou J, Zhang L (2019) Diosgenin inhibits the epithelial-mesenchymal transition initiation in osteosarcoma cells via the p38MAPK signaling pathway. Oncol Lett 18:4278–4287
Ikeda M, Morizane C, Ueno M, Okusaka T, Ishii H, Furuse J (2017) Chemotherapy for hepatocellular carcinoma: current status and future perspectives. Jpn J Clin Oncol 48:103–114
Iranmanesh M, Mohebbati R, Forouzanfar F, Roshan MK, Ghorbani A, Nik MJ, Soukhtanloo M (2018) In vivo and In vitro effects of ethanolic extract of Trigonella foenum-graecum L seeds on proliferation, angiogenesis and tube formation of endothelial cells. Res Pharm Sci 13:343
Jang KY, Noh SJ, Lehwald N, Tao G-Z, Bellovin DI, Park HS, Moon WS, Felsher DW, Sylvester KG (2012) SIRT1 and c-Myc promote liver tumor cell survival and predict poor survival of human hepatocellular carcinomas. PLoS ONE 7:e45119
Kasahara K, Goto H, Izawa I, Kiyono T, Watanabe N, Elowe S, Nigg EA, Inagaki M (2013) PI 3-kinase-dependent phosphorylation of Plk1–Ser99 promotes association with 14-3-3γ and is required for metaphase–anaphase transition. Nat Commun 4:1882
Khalil MI, Ibrahim MM, El-Gaaly GA, Sultan AS (2015) Trigonella foenum (Fenugreek) induced apoptosis in hepatocellular carcinoma cell line, HepG2, mediated by upregulation of p53 and proliferating cell nuclear antigen. Biomed Res Int 2015:914645
Khanal P, Patil VS, Bhandare VV, Patil PP, Patil BM, Dwivedi PSR, Bhattacharya K, Harish DR, Roy S (2022) Systems and in vitro pharmacology profiling of diosgenin against breast cancer. Front Pharmacol 13:1052849
Lee E, Song C-H, Bae S-J, Ha K-T, Karki R (2023) Regulated cell death pathways and their roles in homeostasis, infection, inflammation, and tumorigenesis. Exp Mol Med 55:1632–1643
Lepage C, Léger D, Bertrand J, Martin F, Beneytout J, Liagre B (2011) Diosgenin induces death receptor-5 through activation of p38 pathway and promotes TRAIL-induced apoptosis in colon cancer cells. Cancer Lett 301:193–202
Li F, Fernandez PP, Rajendran P, Hui KM, Sethi G (2010) Diosgenin, a steroidal saponin, inhibits STAT3 signaling pathway leading to suppression of proliferation and chemosensitization of human hepatocellular carcinoma cells. Cancer Lett 292:197–207
Li Y, Wang X, Cheng S, Du J, Deng Z, Zhang Y, Liu Q, Gao J, Cheng B, Ling C (2015) Diosgenin induces G2/M cell cycle arrest and apoptosis in human hepatocellular carcinoma cells. Oncol Rep 33:693–698
Li J, Wang R, Schweickert PG, Karki A, Yang Y, Kong Y, Ahmad N, Konieczny SF, Liu X (2016) Plk1 inhibition enhances the efficacy of gemcitabine in human pancreatic cancer. Cell Cycle 15:711–719
Li HY, Luo F, Li XY, Fu XF, He JF, Tian YZ, Zhu JJ, Chu XY, Zhao HL (2019) Inhibition of polo-like kinase 1 by BI2536 reverses the multidrug resistance of human hepatoma cells in vitro and in vivo. Anti-Cancer Agents in Medicinal Chemistry (Formerly Current Medicinal Chemistry-Anti-Cancer Agents) 19:740–749
Li N, Zhang Z, Jiang G, Sun H, Yu D (2019b) Nobiletin sensitizes colorectal cancer cells to oxaliplatin by PI3K/Akt/MTOR pathway. Front Biosci (landmark Edition) 24:303–312
Li SY, Shang J, Mao XM, Fan R, Li HQ, Li RH, Shen DY (2021) Diosgenin exerts anti-tumor effects through inactivation of cAMP/PKA/CREB signaling pathway in colorectal cancer. Eur J Pharmacol 908:174370
Lin S, Hoffmann K, Schemmer P (2012) Treatment of hepatocellular carcinoma: a systematic review. Liver Cancer 1:144–158
Liu Z, Sun Q, Wang X (2017) PLK1, a potential target for cancer therapy. Transl Oncol 10:22–32
Loor G, Zhang S-J, Zhang P, Toomey NL, Lee MY (1997) Identification of DNA replication and cell cycle proteins that interact with PCNA. NucleicAcidsRes 25:5041–5046
Louwen F, Yuan J (2013) Battle of the eternal rivals: restoring functional p53 and inhibiting Polo-like kinase 1 as cancer therapy. Oncotarget 4:958–971
Malisetty VS, Patlolla JM, Raju J, Marcus LA, Choi C-I, Rao CV (2005) Chemoprevention of colon cancer by diosgenin, a steroidal saponin constituent of fenugreek. Proc Amer Assoc Cancer Res 46:2473
Mao Y, Xi L, Li Q, Cai Z, Lai Y, Zhang X, Yu C (2016) Regulation of cell apoptosis and proliferation in pancreatic cancer through PI3K/Akt pathway via Polo-like kinase 1. Oncol Rep 36:49–56
Mao Y, Xi L, Li Q, Wang S, Cai Z, Zhang X, Yu C (2018) Combination of PI3K/Akt pathway inhibition and Plk1 depletion can enhance chemosensitivity to gemcitabine in pancreatic carcinoma. Transl Oncol 11:852–863
McKay JA, Douglas JJ, Ross VG, Curran S, Loane JF, Ahmed FY, Cassidy J, McLeod HL, Murray GI (2002) Analysis of key cell-cycle checkpoint proteins in colorectal tumours. J Pathol 196:386–393
Morana O, Wood W, Gregory CD (2022) The apoptosis paradox in cancer. Int J Mol Sci 23:1328
Morisaki T, Umebayashi M, Kiyota A, Koya N, Tanaka H, Onishi H, Katano M (2013) Combining celecoxib with sorafenib synergistically inhibits hepatocellular carcinoma cells in vitro. Anticancer Res 33:1387–1395
Murlidhar M, Goswami T (2012) A review on the functional properties, nutritional content, medicinal utilization and potential application of fenugreek. J Food Process Technol 3:1–10
Nishi H, Gotoh K, Tomimaru Y, Kobayashi S, Sasaki K, Iwagami Y, Yamada D, Akita H, Asaoka T, Noda T (2023) Anti-tumor effect of avadomide in gemcitabine-resistant pancreatic ductal adenocarcinoma. Cancer Chemother Pharmacol 92:303–314
Pellegrino R, Calvisi DF, Ladu S, Ehemann V, Staniscia T, Evert M, Dombrowski F, Schirmacher P, Longerich T (2010) Oncogenic and tumor suppressive roles of polo-like kinases in human hepatocellular carcinoma. Hepatology 51:857–868
Peng Y, Tang R, Ding L, Zheng R, Liu Y, Yin L, Fu Y, Deng T, Li X (2023) Diosgenin inhibits prostate cancer progression by inducing UHRF1 protein degradation. Eur J Pharmacol 942:175522
Rohani FG, Mohadesi A, Ansari M (2019) A new diosgenin sensor based on molecularly imprinted polymer of para aminobenzoic acid selected by computer-aided design. J Pharm Biomed Anal 174:552–560
Ruan S, Gu L, Wang Y, Huang X, Cao H (2023) Diosgenin glucoside inhibits the progression of osteosarcoma MG-63 by regulating the PI3K/AKT/mTOR pathway. Anti-Cancer Agents in Medicinal Chemistry (Formerly Current Medicinal Chemistry-Anti-Cancer Agents) 23:1670–1677
Sarkar S, Mazumdar A, Dash R, Sarkar D, Fisher PB, Mandal M (2011) ZD6474 enhances paclitaxel antiproliferative and apoptotic effects in breast carcinoma cells. J Cell Physiol 226:375–384
Sethi G, Shanmugam M, Warrier S, Merarchi M, Arfuso F, Kumar A, Bishayee A (2018) Pro-apoptotic and anti-cancer properties of diosgenin: a comprehensive and critical review. Nutrients 10:645
Sun W, Su Q, Cao X, Shang B, Chen A, Yin H, Liu B (2014) High expression of polo-like kinase 1 is associated with early development of hepatocellular carcinoma. Int J Genomics 2014:312130
Wakharde A, Awad A, Bhagat A, Karuppayil S (2018) Synergistic activation of doxorubicin against cancer: a review. Am J Clin Microbiol Antimicrob 1:1009
Weiß LM, Hugle M, Romero S, Fulda S (2016) Synergistic induction of apoptosis by a polo-like kinase 1 inhibitor and microtubule-interfering drugs in E wing sarcoma cells. Int J Cancer 138:497–506
Wu M, Wang Y, Yang D, Gong Y, Rao F, Liu R, Danna Y, Li J, Fan J, Chen J (2019) A PLK1 kinase inhibitor enhances the chemosensitivity of cisplatin by inducing pyroptosis in oesophageal squamous cell carcinoma. Ebio Med 41:244–255
Yamashita M, Wada H, Eguchi H, Ogawa H, Yamada D, Noda T, Asaoka T, Kawamoto K, Gotoh K, Umeshita K (2016) A CD13 inhibitor, ubenimex, synergistically enhances the effects of anticancer drugs in hepatocellular carcinoma. Int J Oncol 49:89–98
Yao Y, Zhou W-Y, He R-X (2019) Down-regulation of JMJD5 suppresses metastasis and induces apoptosis in oral squamous cell carcinoma by regulating p53/NF-κB pathway. Biomed Pharmacother 109:1994–2004
Yogosawa S, Yoshida K (2018) Tumor suppressive role for kinases phosphorylating p53 in DNA damage-induced apoptosis. Cancer Sci 109:3376–3382
Yoon JH, Choi SK (2023) Management of early-stage hepatocellular carcinoma: challenges and strategies for optimal outcomes. J Liver Cancer 23:300–315
Yousef EH, El-Mesery ME, Habeeb MR, Eissa LA (2020) Polo-like kinase 1 as a promising diagnostic biomarker and potential therapeutic target for hepatocellular carcinoma. Tumor Biology 42:1010428320914475
Yousef EH, El-Magd NFA, El Gayar AM (2023) Carvacrol enhances anti-tumor activity and mitigates cardiotoxicity of sorafenib in thioacetamide-induced hepatocellular carcinoma model through inhibiting TRPM7. Life Sci 324:121735
Yu H, Liu Y, Niu C, Cheng Y (2018) Diosgenin increased DDX3 expression in hepatocellular carcinoma. Am J Transl Res 10:3590–3599
Zhou S, Wen H, Li H (2019) Magnolol induces apoptosis in osteosarcoma cells via G0/G1 phase arrest and p53-mediated mitochondrial pathway. J. Cell Biochem 120:17067–17079
Author information
Authors and Affiliations
Contributions
EHY, L.A.E., M.R.H and M.E.E-M conceptualized the study and developed the protocol. EHY performed the practical work, constructed statistical and data analysis, and finally wrote the manuscript. L.A.E. M.R.H and M.E.E-M. revised the manuscript and supervised the practical work. The authors declare that all data were generated in-house and that no paper mill was used.
Corresponding authors
Ethics declarations
Ethics approval
Research work was agreed by the Mansoura University’s ethics committee (Ethics code: 2023–83).
Competing interests
The authors declare no competing interests.
Additional information
Publisher's note
Springer Nature remains neutral with regard to jurisdictional claims in published maps and institutional affiliations.
Rights and permissions
Springer Nature or its licensor (e.g. a society or other partner) holds exclusive rights to this article under a publishing agreement with the author(s) or other rightsholder(s); author self-archiving of the accepted manuscript version of this article is solely governed by the terms of such publishing agreement and applicable law.
About this article
Cite this article
Yousef, E.H., El-Mesery, M.E., Habeeb, M.R. et al. Diosgenin potentiates the anticancer effect of doxorubicin and volasertib via regulating polo-like kinase 1 and triggering apoptosis in hepatocellular carcinoma cells. Naunyn-Schmiedeberg's Arch Pharmacol 397, 4883–4894 (2024). https://doi.org/10.1007/s00210-023-02894-8
Received:
Accepted:
Published:
Issue Date:
DOI: https://doi.org/10.1007/s00210-023-02894-8