Abstract
Summary
A daughter’s bone mineral density (BMD) is significantly correlated with her mother’s BMD, but the daughter’s body mass index (BMI) could modulate this association. Maternal inheritance dominantly affects daughters with a lower BMI, but BMI could compensate for hereditary influences in daughters with a higher BMI in terms of daughter’s BMD.
Introduction
Achieving optimal peak bone mass at a young age is the best way to protect against future osteoporosis and subsequent fractures. Although environmental components influence bone mass accrual, but peak bone mass is largely programmed by inheritance. The aims of this study were to investigate the influence of maternal inheritance on the daughter’s bone mass and to assess whether these influences differ according to the daughter’s body mass index (BMI).
Methods
We used data obtained from the 2010 Korean National Health and Nutrition Examination Survey V and included 187 mother–daughter pairs. Bone mineral density (BMD) was measured at the lumbar spine (LS), femur neck (FN), and total hip (TH) by using dual-energy X-ray absorptiometry (DXA). The daughter group was stratified into two groups according to the mean BMI (21.4 kg/m2).
Results
The daughters’ BMD correlated significantly with both their BMI and their mothers’ Z-score for each skeletal site. In the daughters with a lower BMI (≤21.4 kg/m2), the BMDs at the FN and TH were affected more by the mothers’ Z-score than by the daughters’ BMI. Meanwhile, the influence of the daughters’ BMI on their BMD was higher than that of their mothers’ Z-score in daughters with a higher BMI (>21.4 kg/m2). Moreover, the mothers’ Z-scores were a significant predictor of their daughters having Z-scores < −1.0 only in daughters with a lower BMI.
Conclusions
This study suggests that maternal inheritance is an important determinant of the daughters’ bone mass, but that this hereditary factor may vary according to the daughters’ BMI.
Similar content being viewed by others
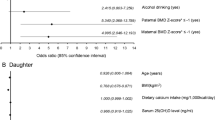
Avoid common mistakes on your manuscript.
Introduction
Osteoporosis is an important public health problem because osteoporotic fractures threaten quality of life, and life expectancy, and increase medical costs worldwide [1–3]. Worldwide, more than 200 million people have osteoporosis, and 1.5 million fractures are reported annually in the USA [4]. The increasing trend in the number of osteoporotic fractures is more evident in Asian countries than in Western countries [5–7]. Osteoporosis is a silent disease but may give lead to serious results in elderly populations.
Osteoporosis is caused by weakening of bone strength, which is determined by bone density and bone quality [8]. Bone density in the elderly is determined by both peak bone mass and subsequent bone loss [9, 10]. Therefore, both achievement of adequate peak bone mass early in life and prevention of accelerated bone loss during aging are important preventive strategies for maintaining bone health throughout one’s life span [8]. The former strategy, which is achieving adequate peak bone mass, requires knowledge about the major determinants and those influences on peak bone mass.
Many genetic and epigenetic factors that affect bone mineral density (BMD) have been investigated [10, 11]. Among these factors, heritability provides the important information for predicting parameters related to bone strength, including peak bone mass. Several studies of mother–daughter pairs have shown that the mother’s BMD is strongly associated with the daughter’s bone mass [12–15]. A meta-analysis reported that parental history of fractures increases the risks of any fracture, osteoporotic fracture, and hip fracture in their offspring [16]. On the basis of this finding, the parents’ hip fracture history is included as one of the major predictors of future osteoporotic fractures in the 10-year fracture risk assessment model (FRAX) [17]. In other words, inheritance primarily affects parameters related to bone strength in a given individual.
Various epigenetic factors related to anthropometric characteristics, environmental effects, and nutritional support that could affect bone mass or fractures have been examined widely [18–20]. Among these factors, body mass index (BMI) has been identified as a major determinant of bone density; that is, a higher BMI increases BMD by inducing greater mechanical loading on skeletal sites [21, 22]. Therefore, in women, a low BMI at the age range at which the bone mass peaks may negatively affect future bone health.
Both hereditary transmission and epigenetic modulation are important for determining peak bone mass and bone strength. However, we do not know which factors are more dominant in determining peak bone mass and bone strength, or whether BMI, an epigenetic factor, can compensate for the effects of inherited determination. This study aimed to investigate the maternal inheritance and BMI on peak bone mass and to assess whether the hereditary influence on peak bone mass is modulated according to BMI.
Materials and methods
Subjects and data collection
This study was based on the data obtained from the first year of the Korean National Health and Nutrition Examination Survey (KNHANES) V, conducted in 2010. KNHANES is a nationwide cross-sectional survey of nationally representative non-institutionalized civilians in the Republic of Korea. This study comprises a health interview, health examination, and nutrition survey. A stratified, multistage probability sampling design was used to select household units, and the civilian, non-institutionalized population of Korea was included in the survey. Details of this survey were recently reported [23]. A total of 8958 participants of 3479 households completed the 2010 KNHANES V. Among those households, 260 households that comprised daughter participants in an age range of 20–35-year-old in which the bone mass peaks were included in this study. Finally, 187 households that had BMD data for both mothers and daughters were analyzed. Any daughters or mothers with renal insufficiency (serum creatinine concentration >1.4 mg/dL) or a history of malignancy were excluded. The subjects who used antiresorptive agents such as raloxifene, bisphosphonate, or hormone-replacement therapy were also excluded in both groups. Natural menopause in mothers group was defined as at least 12 consecutive months of amenorrhea. The daughters were stratified into two groups by their mean BMI: lower BMI group (≤21.4 kg/m2) and group with higher BMI (>21.4 kg/m2). The KNHANES was reviewed and approved by the ethics committee of the Korea Centers for Disease Control and Prevention (KCDC) (2010-02CON-21-C).
BMD measurement
BMD (g/cm2) was measured at the lumbar spine (L1–L4, LS), femoral neck (FN), and total hip (TH) using dual-energy X-ray absorptiometry (DXA, QDR4500A; Hologic Inc., Bedford, MA, USA). The DXA equipment was housed in mobile examination centers. The left hip was scanned routinely, but in participants with a left hip fracture or device, the right hip was scanned. The T-scores in the mothers group were calculated using the mean value and standard deviation (SD) for young normal individuals, and the Z-scores in the mothers and daughters groups were calculated using the mean values and standard deviation (SD)s for each decade from the recently published Korean reference data [24] by the following formula:
The DXA data were analyzed using Hologic Discovery software version 12.1 in its default configuration.
Statistical analysis
Statistical analyses were performed using SPSS version 19 (IBM SPSS Statistics; IBM Corp., Armonk, NY, USA). The ages of mothers ranged from 45 to 67 years, and their menopausal status comprised both with premenopause and menopause. Therefore, we used Z-scores instead of T-scores to eliminate the effects of the contribution of the older ages of mothers to their lower BMD values. Pearson’s correlation coefficient was used to examine the correlation of between the BMD of daughters and the Z-scores of mothers. The mothers group was further stratified into three groups, according to their Z-score, Z-score ≤ −1.0, −1.0 < Z-score ≤ +1.0, and Z-score > +1.0. Linear regression analysis was used to evaluate the relationships between BMD and BMI of the daughters and Z-score of the mothers. The t value was used in regression analysis to estimate the contributiveness of BMI of the daughters or Z-scores of the mothers on daughters’ BMD. A multiple logistic regression analysis for the independent factor of a daughters’ Z-score of <−1.0 was performed to estimate the Exp(β) of mothers’ Z-score in the lower and higher BMI groups. The daughters group was divided into four groups according to their mean BMI or mother Z-score: BMI ≤ 21.4 kg/m2 and mother Z-score < −1.0, BMI ≤ 21.4 kg/m2 and mother Z-score ≥ −1.0, BMI > 21.4 kg/m2 and mother Z-score < −1.0, and BMI > 21.4 kg/m2 and mother Z-score ≥ −1.0. ANOVA was used to compare BMDs at each skeletal site in those four groups. Tests for interaction of daughters’ BMI between mothers’ Z-scores and daughters’ BMD were performed using interaction terms as well as main effects of those two factors in the regression models. P < 0.05 was considered significant.
Results
Baseline characteristics of daughters and their mothers
We analyzed the data for 187 daughter–mother pairs selected from the 2010 KNHANES V. The clinical characteristics of the daughters and mothers groups are shown in Table 1. The mean ages were 26.4 ± 3.9 years in the daughters and 53.1 ± 4.7 years in the mothers. The mean BMI of the daughters was 21.4 kg/m2 (21.4 ± 3.4 kg/m2). The 25-hydroxy vitamin D levels were 14.6 ± 5.4 ng/mL in daughters group and 17.4 ± 6.5 ng/mL in mothers group. Twenty-six (13.9 %) subjects continued regular exercise and 16 (8.6 %) subjects were current smokers in the daughters group. There were no subjects who had experienced fractures in daughters group, whereas 13 (7.0 %) subjects in mothers group had histories of previous fractures. One hundred and thirty-six subjects (73.3 %) in mothers group were postmenopausal, whereas the remaining 50 mothers were premenopausal. The T-scores of mothers group were −0.94 at the LS, −0.94 at the FN, and 0.03 at the TH.
Relationships between the BMD and BMI of daughters or BMD of daughter and the Z-score of mothers
Body size, represented by height, weight, or BMI, is strongly affected by hereditary factors, thus weight and height should be adjusted when comparing variables that are associated with height or weight in parent-offspring pairs [25]. The weight and height of mothers and daughters were also positively correlated in the present study (Supplementary Table 1). The results of the BMI-adjusted model and weight- and height-adjusted model were not different in the present study (data not shown), thus we adjusted for BMI when analyzing the data. To determine the factors the affected the BMD of daughters significantly, we performed a multiple regression analysis for this parameter. We found significant associations between the LS BMD of daughters and age, BMI, and mother Z-score and between the FN and TH BMD of daughters and BMI and mother Z-score (data not shown). Table 2 shows the correlations between the BMD of daughters and their BMI or the Z-score of mothers in each skeletal site, with or without adjustment for the age and BMI of the daughters or the BMI of the mothers. The BMD of daughter was strongly and positively associated with their BMI in each of the three skeletal sites (r = 0.492 for the LS, r = 0.444 for the FN, and r = 0.523 for the TH, p < 0.001). The BMD of daughter was also correlated positively with the Z-score of mothers at each skeletal site; however, this correlation was not as strong as those observed with BMI (r = 0.429 between mother LS Z-score and the LS BMD of daughter; r = 0.315 between mother FN Z-score and the FN BMD of daughter; r = 0.417 between mother TH Z-score and the TH BMD of daughters; p < 0.001) (Table 2). The significant associations observed between the BMD of daughter and mothers’ Z-score were similarly present after adjusting for the age and BMI of daughters, and the BMI of mothers and the relationships were strongest between the same skeletal sites (Table 2).
Relationships between BMD of daughters and the Z-score of mothers in each skeletal site according to the BMI of daughter
Tables 3 shows the correlations between the daughters’ BMD and their mothers’ Z-score in the same skeletal sites in the lower (BMI ≤ 21.4 kg/m2) and higher (BMI > 21.4 kg/m2) BMI groups. In the lower BMI group, daughters’ BMI was significantly positively correlated with their BMD. However, the relationships were weaker than those of the mothers’ Z-scores in the same skeletal site. These patterns were similarly observed in the LS, FN, and TH. The findings of weaker relationships with BMI but stronger associations with mothers’ Z-score were more prominent in the FN and TH. In the higher BMI group, the mothers’ Z-scores in the same skeletal sites also correlated positively with the daughters’ BMD. The correlations between the daughters’ BMI and their BMD were stronger in the higher BMI group compared with the lower BMI group and compared with the mothers’ Z-scores in the FN and TH (Table 3).
The degree of contribution of the BMI of daughters or the Z-score of mothers to the BMD of daughters was compared using the t values obtained from regression analysis (Table 4). In the lower BMI group, the BMD of daughters in the LS was affected more by the LS Z-score of mothers than by their BMI (β = 0.017, t = 2.443, p = 0.017 for the BMI of daughter, β = 0.039, t = 4.665, p < 0.001 for the LS Z-score of mothers). In contrast, the effect of BMI was increased and that of the LS Z-score of mothers was decreased in the daughters with a higher BMI (BMI > 21.4 kg/m2). Furthermore, the effects of BMI and of the LS Z-score of mothers on the BMD of daughters became almost similar in this group (β = 0.015, t = 3.579, p < 0.001 for the BMI of daughter and β = 0.161, t = 3.958, p < 0.001 for the LS Z-score of mothers).
At the FN and TH of the lower BMI group, the BMD of daughter was not significantly related to their BMI and was significantly associated only with their mothers’ Z-score (β = 0.010, t = 1.603, p = 0.012 for the BMI of daughters, and β = 0.026, t = 3.813, p < 0.001 for the FN Z-score of mothers, and β = 0.010, t = 1.751, p = 0.084 for the BMI of daughters, and β = 0.035, t = 5.426, p < 0.001 for the TH Z-score of mothers). However, in the higher BMI group, BMI was significantly related to BMD, and the effect of BMI was much stronger than that the Z-score of mothers at each skeletal site (β = 0.015, t = 3.872, p < 0.001 for the BMI of daughters and β = 0.038, t = 2.363, p = 0.022 for the FN Z-score of mothers, and β = 0.015, t = 4.244, p < 0.001 for the BMI of daughters and β = 0.044, t = 2.981, p < 0.004 for the TH Z-score of mothers) (Table 4).
Associations between the mothers’ Z-scores and daughters’ risk of having Z-scores < −1.0
In the daughters with a lower BMI, the mothers’ Z-scores were significantly associated with the daughters’ low Z-scores of <−1.0 at all three skeletal sites (Exp(β) = 0.574, 95 % confidence interval [CI] = 0.356–0.942 for the LS, Exp(β) = 0.476, 95 % CI = 0.2880–0.786 for the FN, and Exp(β) = 0.462, 95 % CI = 0.275–0.778 for the TH, p < 0.05, respectively) (Table 5). However, in the daughters with a higher BMI, the significant association observed between the mothers’ Z-scores and daughters’ lower Z-scores at the level <−1.0 disappeared in the LS, FN, or TH (Table 5). After further adjustment for 25-hydroxyvitamin D levels, smoking status, and regular exercise, the results were unchanged in both groups.
Comparisons of the BMD of daughters in the LS, FN, and TH according to their BMI and mothers’ Z-score in the same skeletal sites
We compared the daughters’ BMD in the LS, FN, and TH according to the daughters’ BMI (BMI ≤ 21.4 kg/m2 or BMI > 21.4 kg/m2) and the mothers’ Z-score (Mothers’ Z-score < −1.0 or Mothers’ Z-score ≥ −1.0). The daughters group with lower BMI and mothers’ Z-score of less than −1.0 showed significantly lowest BMD values in the LS, FN, and TH (Fig. 1). Whereas, the daughters group with higher BMI and mothers Z-score of higher than −1.0 provided significant highest BMD values in three skeletal sites. There were no significant differences between the daughters group with lower BMI and mothers Z-score of higher than −1.0 and group with higher BMI but mothers Z-score of less than −1.0 in the LS, FN, and TH (Fig. 1). We examined the interaction term of daughters’ BMI with the mothers’ Z-score on daughters’ BMD in three skeletal sites, and we found significant interactions in all sites (p < 0.05, respectively) (Fig. 1).
The BMD of the daughters in the LS (a), FN (b), and TH (c) according to BMI of the daughters and the Z-scores of the mothers in the same skeletal sites. Values with different superscripts are significantly different (p < 0.05 with Bonferroni correction). Interaction terms were provided as β values with 95 % confidence intervals (*p < 0.05)
Discussion
In the present study, we found an evidence of a hereditary relationship for BMD between mothers and daughters, which reflects the strong genetic influence on the determination of BMD. However, this hereditary relationship was modulated by daughter’s BMI. That is, the hereditary influence was significant and strong in the daughters with lower BMI but was diminished in the daughters with higher BMI. In the daughters with higher BMI, the impact of their BMI on BMD was greater than that of their mothers’ Z-scores. In other words, heritability had greater influence in the lower BMI group, whereas BMI was more influential than hereditary transmission in the higher BMI group in determining bone mass.
The hereditary nature of BMD has been previously reported. Several studies have shown significant associations for BMD between mothers and their daughters both at the lumbar spine and femur [12, 26, 27]. In one study, premenopausal daughters of women with hip fractures showed significantly lower femoral BMD, indicating that the daughters were at higher risk of future hip fractures [28]. The genetic influence on BMD has also been observed in mothers and their prepubertal children, and the relationship between mothers and their offspring was significantly higher in mother–daughter pairs than in mother–son pairs [29]. Recently, the genetic bases of specific diseases have been studied widely by using analysis of single nucleotide polymorphisms (SNPs) [30] whose existence can be used to demonstrate the genetic background of a specific disease [31, 32]. Several SNPs related to bone strength have been reported in previous studies [33–35]. These findings suggest that genetic factors are major determinants of bone health-related parameters.
Body composition such as body weight and BMI correlated strongly with BMD. Higher BMI is known to have a beneficial effect on BMD by inducing mechanical loading on the skeleton [21, 22, 36, 37]. One recent study reported that people with a BMI of 18 kg/m2 had a rapider bone loss rate at femoral site than those with a BMI of 30 kg/m2 [19]. Another study reported that the mean LS BMD increased with increasing body weight quartiles and that the influence of lifestyle factors was stronger in postmenarcheal daughters than in premenarcheal daughters [14]. Birth weight was reported as an independent determinant of adult bone mass [38]. Consistent with previous data, our data indicate that the daughters’ BMI was positively correlated with their BMD.
On the other hand, the effects of the two important determining factors of daughters’ BMD, namely the daughters’ BMI and the mothers’ Z-score, differ according to the daughters’ BMI. BMD in the lower BMI group was more affected by hereditary factors, as represented by the mother’s BMD, than by the daughters’ BMI. The influence of BMI increased, but the inherited influence decreased in the daughter group with higher BMI, and the effect of BMI on BMD was stronger that of hereditary factor in this group. This suggests that the inherited influences on bone mass may vary according to the BMI of specific individuals.
In the present study, the associations of the daughters’ BMI and the mothers’ Z-score with the daughters’ BMD varied according to the skeletal sites. The hereditary influence represent differently according to the skeletal site [39]. Moreover, the effects of body compositional parameters on BMD also provide skeletal site specificity regarding factors related to weight-bearing [40]. Based on these findings, it is conceivable that the relative degree of contribution of BMI and heredity to BMD might be distinguished from the properties of the skeletal sites.
The present study has several strengths. To our knowledge, this is the first study to demonstrate that BMI modulates hereditary influences on BMD. We used data from the 2010 KNHANES, which is a representative nationwide survey, and we included a relatively large number of mother–daughter pairs. We found evidence of a compensatory role of BMI on genetic influences on BMD. However, there are also several limitations in this study. First, consistent with the body composition data for this population [41], the percentage of individuals in the obese population (with a BMI of >25.0 kg/m2) and in the low-weight population (with a BMI of <18.5 kg/m2) was around 15 % in each case. Because of the low percentage of individuals in the obese or low-weight groups, we were not able to analyze the manner in which obesity or low-weight affects hereditary effects on BMD in this population. Instead, we used the mean BMI level as a cut-off for the lower and higher BMI groups. Second, this was a subgroup analysis that included only 5 % of the households from among the whole study population. Therefore, selected participation may have introduced some bias in determining the familial interactions that affecting BMD. The generalizability of the results should be further validated in other study populations. Third, this was a cross-sectional study, and we could not investigate how weight change in the daughters might affect the achievement of peak bone mass and the inherited influences on BMD. Finally, we did not compare the daughters’ BMD according to their mothers’ fracture history because the mothers were relatively young and their rate of fractures was <5 %.
In conclusion, although maternal inheritance is well known as an important determinant of bone mass, this hereditary factors can be somewhat overcome by having a higher BMI. The maintenance of a healthy body weight during the period of attainment of peak bone mass may be effective for preventing later osteoporosis or fractures later in life.
Abbreviations
- LS:
-
Lumbar spine
- FN:
-
Femur neck
- TH:
-
Total hip
- DXA:
-
Dual-energy x-ray absorptiometry
- BMI:
-
Body mass index
References
Cummings SR, Melton LJ (2002) Epidemiology and outcomes of osteoporotic fractures. Lancet 359:1761–1767
Bliuc D, Nguyen ND, Milch VE, Nguyen TV, Eisman JA, Center JR (2009) Mortality risk associated with low-trauma osteoporotic fracture and subsequent fracture in men and women. JAMA 301:513–521
Cauley JA (2013) Public health impact of osteoporosis. J Gerontol A Biol Sci Med Sci 68:1243–1251
Reginster JY, Burlet N (2006) Osteoporosis: a still increasing prevalence. Bone 38:S4–S9
Lee YK, Yoon BH, Koo KH (2013) Epidemiology of osteoporosis and osteoporotic fractures in South Korea. Endocrinol Metab (Seoul) 28:90–93
Nojiri S, Burge RT, Flynn JA, Foster SA, Sowa H (2013) Osteoporosis and treatments in Japan: management for preventing subsequent fractures. J Bone Miner Metab 31:367–380
Ballane G, Cauley JA, Luckey MM, Fuleihan GE (2014) Secular trends in hip fractures worldwide: opposing trends east versus west. J Bone Miner Res 29(8):1745–1755
NIH Consensus Development Panel on Osteoporosis Prevention, Diagnosis, and Therapy (2001) Osteoporosis prevention, diagnosis, and therapy. JAMA 285:785–795
Harvey N, Dennison E, Cooper C (2014) Osteoporosis—a lifecourse approach. J Bone Miner Res 29(9):1917–1925
Rizzoli R, Bonjour JP, Ferrari SL (2001) Osteoporosis, genetics and hormones. J Mol Endocrinol 26:79–94
Pollitzer WS, Anderson JJ (1989) Ethnic and genetic differences in bone mass: a review with a hereditary vs environmental perspective. Am J Clin Nutr 50:1244–1259
Seeman E, Hopper JL, Bach LA, Cooper ME, Parkinson E, McKay J, Jerums G (1989) Reduced bone mass in daughters of women with osteoporosis. N Engl J Med 320:554–558
Barthe N, Basse-Cathalinat B, Meunier PJ, Ribot C, Marchandise X, Sabatier JP, Braillon P, Thevenot J, Sutter B (1998) Measurement of bone mineral density in mother-daughter pairs for evaluating the family influence on bone mass acquisition: a GRIO survey. Osteoporos Int 8:379–384
Kuroda T, Onoe Y, Miyabara Y, Yoshikata R, Orito S, Ishitani K, Okano H, Ohta H (2009) Influence of maternal genetic and lifestyle factors on bone mineral density in adolescent daughters: a cohort study in 387 Japanese daughter-mother pairs. J Bone Miner Metab 27:379–385
Danielson ME, Cauley JA, Baker CE, Newman AB, Dorman JS, Towers JD, Kuller LH (1999) Familial resemblance of bone mineral density (BMD) and calcaneal ultrasound attenuation: the BMD in mothers and daughters study. J Bone Miner Res 14:102–110
Kanis JA, Johansson H, Oden A et al (2004) A family history of fracture and fracture risk: a meta-analysis. Bone 35:1029–1037
McCloskey E, Kanis JA (2012) FRAX updates 2012. Curr Opin Rheumatol 24:554–560
Ohta H, Kuroda T, Onoe Y, Nakano C, Yoshikata R, Ishitani K, Hashimoto K, Kume M (2010) Familial correlation of bone mineral density, birth data and lifestyle factors among adolescent daughters, mothers and grandmothers. J Bone Miner Metab 28:690–695
Emaus N, Wilsgaard T, Ahmed LA (2014) Impacts of body mass index, physical activity, and smoking on femoral bone loss: the tromso study. J Bone Miner Resh 29(9):2080–2089
Runyan SM, Stadler DD, Bainbridge CN, Miller SC, Moyer-Mileur LJ (2003) Familial resemblance of bone mineralization, calcium intake, and physical activity in early-adolescent daughters, their mothers, and maternal grandmothers. J Am Diet Assoc 103:1320–1325
Gunn CA, Weber JL, Kruger MC (2014) Diet, weight, cytokines and bone health in postmenopausal women. J Nutr Health Aging 18:479–486
Lloyd JT, Alley DE, Hawkes WG, Hochberg MC, Waldstein SR, Orwig DL (2014) Body mass index is positively associated with bone mineral density in US older adults. Arch Osteoporos 9:175
Kweon S, Kim Y, Jang MJ, Kim Y, Kim K, Choi S, Chun C, Khang YH, Oh K (2014) Data resource profile: the Korea National Health and Nutrition Examination Survey (KNHANES). Int J Epidemiol 43:69–77
Park EJ, Joo IW, Jang MJ, Kim YT, Oh K, Oh HJ (2014) Prevalence of osteoporosis in the Korean population based on Korea National Health and Nutrition Examination Survey (KNHANES), 2008–2011. Yonsei Med J 55:1049–1057
Seeman E, Hopper JL, Young NR, Formica C, Goss P, Tsalamandris C (1996) Do genetic factors explain associations between muscle strength, lean mass, and bone density? A twin study. Am J Physiol 270:E320–E327
Lutz J, Tesar R (1990) Mother-daughter pairs: spinal and femoral bone densities and dietary intakes. Am J Clin Nutr 52:872–877
Jones G, Nguyen TV (2000) Associations between maternal peak bone mass and bone mass in prepubertal male and female children. J Bone Miner Res 15:1998–2004
Seeman E, Tsalamandris C, Formica C, Hopper JL, McKay J (1994) Reduced femoral neck bone density in the daughters of women with hip fractures: the role of low peak bone density in the pathogenesis of osteoporosis. J Bone Miner Res 9:739–743
McKay HA, Bailey DA, Wilkinson AA, Houston CS (1994) Familial comparison of bone mineral density at the proximal femur and lumbar spine. Bone Miner 24:95–107
Wang DG, Fan J-B, Siao C-J, Berno A, Young P, Sapolsky R, Ghandour G, Perkins N, Winchester E, Spencer J (1998) Large-scale identification, mapping, and genotyping of single-nucleotide polymorphisms in the human genome. Science 280:1077–1082
Sladek R, Rocheleau G, Rung J, Dina C, Shen L, Serre D, Boutin P, Vincent D, Belisle A, Hadjadj S (2007) A genome-wide association study identifies novel risk loci for type 2 diabetes. Nature 445:881–885
Doris PA (2002) Hypertension genetics, single nucleotide polymorphisms, and the common disease: common variant hypothesis. Hypertension 39:323–331
Zheng HF, Tobias JH, Duncan E et al (2012) WNT16 influences bone mineral density, cortical bone thickness, bone strength, and osteoporotic fracture risk. PLoS Genet 8:e1002745
Koller DL, Zheng HF, Karasik D et al (2013) Meta-analysis of genome-wide studies identifies WNT16 and ESR1 SNPs associated with bone mineral density in premenopausal women. J Bone Miner Res 28:547–558
Koller DL, Ichikawa S, Lai D et al (2010) Genome-wide association study of bone mineral density in premenopausal European-American women and replication in African-American women. J Clin Endocrinol Metab 95:1802–1809
De Marchi G, Ferraccioli G (2002) Leptin: regulatory role in bone metabolism and in inflammation. Reumatismo 54:217–225
Beck TJ, Petit MA, Wu G, LeBoff MS, Cauley JA, Chen Z (2009) Does obesity really make the femur stronger? BMD, geometry, and fracture incidence in the women’s health initiative-observational study. J Bone Miner Res 24:1369–1379
Dennison EM, Syddall HE, Sayer AA, Gilbody HJ, Cooper C (2005) Birth weight and weight at 1 year are independent determinants of bone mass in the seventh decade: the Hertfordshire cohort study. Pediatr Res 57:582–586
Shaffer JR, Kammerer CM, Bruder JM, Cole SA, Dyer TD, Almasy L, MacCluer JW, Blangero J, Bauer RL, Mitchell BD (2008) Genetic influences on bone loss in the San Antonio Family Osteoporosis study. Osteoporos Int 19:1759–1767
Sukumar D, Schlussel Y, Riedt CS, Gordon C, Stahl T, Shapses SA (2011) Obesity alters cortical and trabecular bone density and geometry in women. Osteoporos Int 22:635–645
Hur YM, Kaprio J, Iacono WG et al (2008) Genetic influences on the difference in variability of height, weight and body mass index between Caucasian and East Asian adolescent twins. Int J Obes (Lond) 32:1455–1467
Acknowledgments
This study was supported by a research grant 02-2013-051 from Seoul National University Bundang Hospital and by a Basic Science Research Program through the National Research Foundation of Korea (NRF) funded by the Ministry of Science, ICT & Future Planning (NRF-2014R1A1A3051310).
Authors’ role: study design: KMK and CSS. Data collection: KMK and YJK. Data analysis: KMK, YJK, and CSS. Data interpretation: KMK, SHC, SL, HCJ, SWK, and CSS. Drafting manuscript: KMK, YJK, and CSS. Approving final version of manuscript: KMK, YJK, SHC, SL, SHC, SWK, HCJ, and CSS. CSS takes responsibility for the integrity of the data analysis.
Author information
Authors and Affiliations
Corresponding author
Ethics declarations
Conflicts of interest
None.
Additional information
K. M. Kim and Y. J. Kim contributed equally to this work.
Electronic supplementary material
Below is the link to the electronic supplementary material.
Supplementary Table 1
(DOCX 17 kb)
Rights and permissions
About this article
Cite this article
Kim, K.M., Kim, Y.J., Choi, S.H. et al. The effects of body mass index on the hereditary influences that determine peak bone mass in mother–daughter pairs (KNHANES V). Osteoporos Int 27, 2057–2064 (2016). https://doi.org/10.1007/s00198-016-3487-9
Received:
Accepted:
Published:
Issue Date:
DOI: https://doi.org/10.1007/s00198-016-3487-9