Abstract
Sulphur hexafluoride (SF6) is currently the preferred dielectric gas used in medium and high voltage power equipment. However, due to the extremely greenhouse effect by SF6, the search for alternative gas comprising a fluoro-organic gas with low environmental concern mixed with a buffer gas (N2, CO2 and O2) have received considerable attention from the power industry. Trifluoroiodomethane (CF3I) is identified to have an excellent insulation performance with a very low GWP can replace SF6. This paper presents the basic lightning impulse performance of CF3I mixed with CO2 as an alternative to SF6 insulation gas. The 50% breakdown voltage (U50) under both positive and negative polarities were measured at various gas pressure and mixing ratio of CF3I–CO2. The results show that U50 of CF3I–CO2 gas mixtures increases linearly with the pressure, and the difference increases with gap length. Furthermore, an increment of CF3I content in the mixtures increases the insulation strength. It was also found that U50 with negative polarity is much higher than that with positive polarity under the needle-plane electrode and vice versa under the plane-plane configuration. The V-t characteristics for CF3I–CO2 were also analysed under these two different electric fields. The V-t characteristics for plane-plane electrode are more distributed along the wavetail of the lightning impulse under both U50 polarities. As opposed to the V-t waveforms under needle-plane electrode, the instantaneous breakdowns tend to happen earlier. Overall, the results provide a basis for considering the application of CF3I–CO2 as an insulating gas in high voltage apparatus.
Access provided by Autonomous University of Puebla. Download conference paper PDF
Similar content being viewed by others
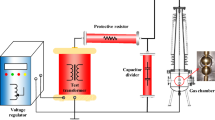
Keywords
1 Introduction
Sulphur hexafluoride (SF6) is widely recognised as the best insulation gas in high voltage apparatus due to its outstanding insulation strength and arc quenching properties. But, the longtime excessive usage of SF6 may increase the percentage of its global warming potential (GWP) and have an extremely long atmospheric lifetime [1, 2]. For these reasons, limiting the application of SF6, or eventually replaced it by using alternative gases is necessary for the developed countries such as EU, US and China [3].
Much research in recent years has focused on gases or mixed gas, especially the fluorocarbons with halogenated gases or fluorinated compounds, which have similar or better dielectric strength to that of SF6. Although some perfluorocarbons (PFCs) and hydrofluorocarbons (HFCs) offer an excellent breakdown strength, their GWP remains high, typically in the range of 5000–12,000 [4, 5]. Their lifetime is also relatively higher than SF6, thus limiting them from consideration. One of the most promising low GWP alternatives is trifluoroiodomethane (CF3I). Its GWP is less than 5, and the insulation strength of CF3I is 1.2 times higher than that of SF6 [6, 7].
CF3I is colorless, odorless and non-flammable have considered being an environmentally friendly insulation gas [8, 9]. Due to the high liquefaction temperature, CF3I needs to be mixed with buffer gases (N2 or CO2) that have relatively low boiling point [10, 11]. Thus, the problem of high liquefaction temperature of CF3I can be avoided and the mixtures could possess identical features like economical cost, good dielectric performance and provide less environmental concerns.
In this paper, the fundamental lightning impulse withstand of CF3I–CO2 gas mixtures are measured at a pressure range of 1.0 bar to 2.0 bar. In addition, the effect of various mixture ratios on the insulation performance of CF3I–CO2 gas mixtures was also analysed. From this study, as the CF3I content increased, the dielectric strength is more significant in the plane-plane electrode. Meanwhile, in the needle-plane electrode, the increment in the dielectric properties is noticed only in the small gap length.
2 Experimental Arrangement and Method
Figure 35.1 shows the complete block diagram of the test setup. The specific details of the lightning impulse generation, structural of pressure vessel, as well as electrode geometry are described in this section.
2.1 Lightning Impulse Test
The impulse voltages were generated by a four (4) stage Marx impulse generator. The maximum output voltage level is 400 kV, delivering 100 kV per stage [12]. The impulse voltage was then measured by a 50 ns rise time capacitive voltage divider and recorded using a digital oscilloscope. The up-and-down method was used to obtain the 50% breakdown voltage (U50) with at least 20 impulse shots for each test condition.
2.2 Pressurized Vessel
The cylindrical-shaped pressure vessel was built with a height of 500 mm, having a radius of 250 mm, and a thickness of 10 mm, giving a volume around 0.0982 m3, or 98.2 L. The main constituent material of the vessel is mild steel, a non-alloy structural steel that can withstand specific high pressure and high voltage stresses. Meanwhile, the vessel window is made of polycarbonate, in which able to observe the experimental phenomena easily.
2.3 Electrode Configuration
A needle-plane and plane-plane electrode were used in this study. The diameter of the needle electrode is 12 mm, having a tip curvature diameter of 1 mm, while the diameter of the plane electrode is 90 mm, the thickness is 15 mm, and the chamfer radius is 5 mm. All electrodes are made from brass. The electrodes surface was mirror finished to ensure no effect from protrusion, compromising the test results.
3 Result and Discussion
This section focuses on both positive and negative lightning impulse breakdown testing. The effect of various gas pressure and different mixture ratios on the impulse breakdown characteristics of CF3I–CO2 were briefly discussed.
3.1 Breakdown Performance of CF3I–CO2 Mixture Under Different Pressures
Figure 35.2 shows the impulse breakdown voltage-pressure relationship of 30%CF3I–70%CO2 gas mixture under positive and negative polarity at different gas pressures and various electrode gap distances. The U50 of CF3I mixture increase with the increasing pressure range between 1.0 bar and 2.0 bar (abs). It is noted that the saturation value is approached more gradually at a higher pressure, especially in 5 cm gap under both U50 polarities.
The V-t characteristics in Fig. 35.3 indicate the positive lightning impulse breakdown properties of CF3I–CO2 gas mixtures at the respective pressure range studied. In the case of positive impulse polarity under a non-uniform (needle-plane electrode) configuration, most of the breakdowns appeared around the wavefront duration corresponding to the peak value, less than 8 µs. A closer examination of the results in Fig. 35.3a reveals that the duration of the breakdown voltage at 1 cm gap length under 1.0 bar pressure occurs near the peak value in the region of 1–2 µs. When the gas pressure is changed to 1.5 bar and 2.0 bar, the breakdown voltages are more dispersed throughout the time scale, reach to 6 µs, as shown in Fig. 35.3b, c, respectively. At a pressure of 2.0 bar, shown in Fig. 35.3c, the V-t plots for a 4 cm gap is almost similar to that for a 5 cm gap with only 2 kV difference. This behaviour shows the saturation phenomenon under positive lightning impulse at higher pressure and gap length.
Figure 35.4 shows the corresponding V-t waveforms of CF3I–CO2 mixtures under negative polarity in needle-plane electrode configuration. As observed in Fig. 35.2, 50% breakdown voltages under negative lightning impulse are significantly higher than those under positive impulses in an expanded range of pressures. The differences in U50 increase as the gap increase. Furthermore, the increase rate of U50 is more significant over the gap distance between electrodes at all pressures. The increasing trend can be noticed in Fig. 35.4 when compared to Fig. 35.3. A relatively significant time lag was observed at lower pressure of 1.0 bar in the negative impulse under the non-uniform field. However, the V-t waveforms at higher pressure are concentrated near the peak value with a duration of less than 6 µs.
3.2 Breakdown Performance of CF3I–CO2 Mixture Under Different Pressures
Further impulse breakdown tests were carried out for three (3) different mixture ratios, which are 20%–80%, 30%–70% and 40%–60% of CF3I–CO2 at a pressure of 1.0 bar. The tests involved two (2) different electrode arrangements, including the needle-plane and the plane-plane configuration.
Effects of CF3I Content in Needle-Plane Configuration. The breakdown voltage (U50) at different CF3I–CO2 gas mixing ratios for various gap distance is plotted in Fig. 35.5. It is noted that the increment of U50 is nearly linear with the increasing electrode gap under positive impulse voltage and gradually increase for negative impulse voltage. However, as expected in a needle-plane electrode, U50 under the negative impulse is probable to be higher than those under positive polarity. This phenomenon shows that the electron emission from the cathode surface was remarkable at the higher electric field under a non-uniform configuration. Therefore, U50 would have the maximum value to initiate a complete breakdown.
The growth trend of U50 curves is obvious under negative impulse polarity with the increase of CF3I content. The comparison can be made between the highest and the lowest concentration of CF3I in CF3I–CO2 gas mixtures. The U50 of 40%CF3I–60%CO2 mixture is about 21% higher than that of 20%CF3I–80%CO2 at a 1 cm gap distance. For the 3 cm and 5 cm gap, U50 of the highest CF3I content is 12 and 10% higher. While a slight effect on the positive impulse breakdown voltages can be seen when changing the proportion of CF3I electronegative gas from 20 to 40% in CF3I–CO2 mixtures. There is only 11% increase of U50 at 1 cm gap, 6% at 3 cm gap, and 5% at 5 cm gap distance. From the result, the effect of CF3I content in the CF3I–CO2 mixtures was noted on smaller gaps under both polarities.
Figure 35.6 shows the V-t plots of CF3I–CO2 under non-uniform field electrode at 1 cm gap for both positive and negative standard lightning impulse. As mentioned in previous discussion, the breakdown voltages under a positive polarity tend to happen earlier, near the peak value in the range of 1–2 µs under 1.0 bar gas pressure. This occurrence can be seen for all CF3I–CO2 mixing ratios shown in Fig. 35.6a, caused by the developing speed of discharge under a non-uniform electrode configuration. Under the negative polarity, the duration of the instantaneous voltages is longer and more scattered along the negative U50 wavetail shown in Fig. 35.6b. Among the three mixture ratios of CF3I–CO2, the V-t-plot for 40%CF3I–60%CO2 gas mixture is the highest under both impulse polarities.
Effects of CF3I Content in Plane-Plane Configuration. The effect of CF3I content in CF3I–CO2 mixtures was further observed under a more uniform electric field, which represents by the plane-plane electrode configuration. The tests involved gap lengths between 1 and 3 cm. Based on the data demonstrated in Fig. 35.7, the standard impulse breakdown voltages (U50) under a uniform electrode contrast from those under a non-uniform electrode, which means the U50 with positive standard lightning impulse is higher. In all mixtures, U50 values increase linearly with increasing the gap distance between electrodes.
Furthermore, it is noted that a slight increment in U50 between positive and negative impulse polarity when CF3I content was increased. Under positive polarity, the relationship between U50 and the gap length at different mixing ratios of CF3I–CO2 is almost linear. An increase from 20 to 40% of CF3I in the mixture shows significantly less changes for the slope of the U50. About 20% increase of U50 at 1 cm gap, 22% at 2 cm gap, and 22% at 3 cm gap distance. A smaller percentage difference in U50 also can be found under negative polarity while varying the proportion of CF3I content in the mixture. The U50 of 40%CF3I–60%CO2 mixture is higher than 20%CF3I–80%CO2 about 15% at a 1 cm gap, 12% and 10% at a corresponding 3 cm and 5 cm gap.
Figure 35.8 shows the V-t waveforms at 1 cm plane-plane electrode gap for both lightning impulse polarities. In contrast to the non-uniform electrode arrangement, the instantaneous breakdowns in a more uniform field electrode are more distributed along the wavetail time duration under both positive and negative polarity. Among all mixture ratios, the V-t characteristic for CF3I–CO2 at 40%–60% remains highest similar to the V-t plots in the needle-plane electrode.
4 Conclusion
The dielectric strength of CF3I–CO2 mixtures is significant at higher pressures. The 50% breakdown voltages, U50 for both lightning impulse polarities increase over the pressure range under the plane-plane and the needle-plane electrode arrangement. When the gap between the electrode is varied, U50 of CF3I–CO2 mixtures shows the same increment trend as the change in pressure. Furthermore, the increment in CF3I concentration increase the dielectric strength of CF3I–CO2, obviously in the plane-plane configuration compared to the needle-plane configuration. On the other hand, the V-t characteristics for CF3I–CO2 under uniform electric field (plane-plane electrode) are more dispersed along the U50 wavetail duration for both impulse polarities. Unlike the V-t waveforms under the non-uniform field (needle-plane electrode), the instantaneous voltages tend to occur earlier at the peak value of the wavefront duration. However, in all mixture ratios, V-t-characteristic for CF3I–CO2 at 40%–60% is the highest under both lightning impulse polarities. Overall, the insulation performance of CF3I–CO2 gas mixtures has been proved and can be considered a possible substitute to SF6 as a new insulating medium.
References
S. Xiao, X. Zhang, J. Tang, S. Liu, A review on SF6 substitute gases and research status of CF3I gases. Energy Rep. 4, 486–496 (2018)
B. Khan et al., Analysis of the dielectric properties of R410A gas as an alternative to SF for high-voltage applications. High Voltage 4(1), 41–48 (2019)
M.S. Kamarudin et al., CF3I gas and its mixtures: potential for electrical insulation, in International Conference on Large High Voltage Electric Systems (CIGRE) Session 45, 2014
X. Li, H. Zhao, A.B. Murphy, SF6-alternative gases for application in gas-insulated switchgear. J. Phys. D: Appl Phys. 51(15) (2018)
H.S. Kharal, M. Kamran, R. Ullah, M.Z. Saleem, M.J. Alvi, Environment-friendly and efficient gaseous insulator as a potential alternative to SF6. Processes 7, 1–13 (2019)
M.S. Kamarudin, M. Albano, P. Coventry, N. Harid, A. Haddad, A survey on the potential of CF3I gas as an alternative for SF6 in high voltage applications, in 45th International Universities Power Engineering Conference (UPEC), 2010
X. Zhang, S. Xiao, Y. Han, Q. Dai, Analysis of the feasibility of CF3I/CO2 used in C-GIS by partial discharge inception voltages in positive half cycle and breakdown voltages. IEEE Trans. Dielectr. Electr. Insul. 22(6), 3234–3243 (2015)
X. Li, H. Zhao, J. Wu, S. Jia, Analysis of the insulation characteristics of CF3I mixtures with CF4, CO2, N2, O2 and air. J. Phys. D: Appl. Phys. 46(34), 345203 (2013)
X. Zhang, S. Xiao, J. Zhou, J. Tang, Experimental analysis of the feasibility of CF3I/CO2 substituting SF6 as insulation medium using needle-plate electrodes. IEEE Trans. Dielectr. Electr. Insul. 21(4), 1895–1900 (2014)
S. Zhao, D. Xiao, P. Xue, R. Zhong, Analysis of insulation performance and polar effect of CF3I/CO2 mixtures. IEEE Trans. Dielectr. Electr. Insul. 25(4), 1364–1370 (2018)
B. Zhou, D. Tan, J. Xue, F. Cai, D. Xiao, Lighting impulse withstand performance of CF3I-N2 gas mixture for 252 kV GIL insulation. IEEE Trans. Dielectr. Electr. Insul. 26(4), 1190–1196 (2019)
T. Wen et al., Discussion on lightning impulse test waveform according to breakdown characteristics of SF6 gas gaps. IEEE Trans. Dielectr. Electr. Insul. 24(4), 2306–2313 (2017)
Acknowledgements
The authors gratefully acknowledge the financial supports from the Ministry of Higher Education Malaysia under the Fundamental Research Grant Scheme Vot No. FRGS/1/2018/TK10/UTHM/02/10 and partially sponsored by Universiti Tun Hussein Onn Malaysia.
Author information
Authors and Affiliations
Corresponding author
Editor information
Editors and Affiliations
Rights and permissions
Copyright information
© 2022 The Author(s), under exclusive license to Springer Nature Singapore Pte Ltd.
About this paper
Cite this paper
Kamarudin, M.S., Badrul Sham, N.M., Haddad, A., Baharom, M.N.R., Mohd Yousof, M.F., Othman, N.A. (2022). Impulse Breakdown Characteristics of CF3I–CO2 at Various Gas Pressure and CF3I Content. In: Mustapha, A.B., Shamsuddin, S., Zuhaib Haider Rizvi, S., Asman, S.B., Jamaian, S.S. (eds) Proceedings of the 7th International Conference on the Applications of Science and Mathematics 2021. Springer Proceedings in Physics, vol 273. Springer, Singapore. https://doi.org/10.1007/978-981-16-8903-1_35
Download citation
DOI: https://doi.org/10.1007/978-981-16-8903-1_35
Published:
Publisher Name: Springer, Singapore
Print ISBN: 978-981-16-8902-4
Online ISBN: 978-981-16-8903-1
eBook Packages: Physics and AstronomyPhysics and Astronomy (R0)