Abstract
In this chapter, the autogenic process of alluvial fan under various discharges and sediment feed rates were studied. Meanwhile, the discharge and sediment feed rate were suddenly changed during some experiments to analyze alluvial fan’s delayed response to external disturbances. The results demonstrate that progradation processes both slow down when water discharge or sediment feed rate is halved separately. When reducing both water discharge and sediment feed rate simultaneously, progradation process become even slower. Widening process of alluvial fan is less sensitive to change of discharge and sediment concentration than progradation process. When sediment feed rate is reduced by half, alluvial fan aggradation processes along flow path slows down obviously. When water discharge is reduced by half, upstream aggradation processes accelerate while downstream aggradation processes decelerate. The alluvial fan fluvial cycle and the channel morphology react to discharge reduction differently with sediment concentration reduction. The rate law can be employed to describe the actual response process of the deposition thickness. Calculated results demonstrate that the response rate of the upper-fan siltation layer is higher than that of the mid-fan and lower-fan. The response rate of the fine-sand alluvial fan is higher than that of coarse-sand. The response of the deposition layer to discharge increase is faster than that of discharge decrease. Alluvial fan development is influenced by both fan reaction mode and the status of fan development.
Access provided by Autonomous University of Puebla. Download conference paper PDF
Similar content being viewed by others
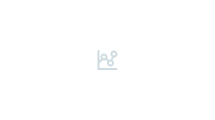
Keywords
1 Introduction
Alluvial fan is formed by sediment deposition from sediment-laden flow (Nemec and Steel 1988). The continuous expansion of alluvial fans is an important mechanism for the formation of alluvial plains. The essence of this process is the symbiotic development of alluvial rivers and surrounding sedimentary geomorphology. The plain landforms formed by continuous development of alluvial fans can be divided into two types. The first type is characterised by the deposition which area is wide and the development of alluvial plan which is not restricted laterally. The other type is characterised by its development in narrow river valleys, which is subject to obvious lateral constraints. Many research studies focused on the development of the first type of alluvial fan, including geological field surveys, physical model tests, numerical simulations and so on (Hashimoto et al. 2011; Dirszowsky and Desloges 2004; Van Dijk et al. 2012; Caldwell and Edmonds 2014). Due to the appearance of boundary wall constraints, the development process of the laterally constrained alluvial fan is more complex. Most studies are concentrated on geological field surveys, while less attention is paid to the symbiotic development of sediment accumulation and river evolution (Ravazzi et al. 2013; Taha and Anderson 2008).
Zhang et al. (2016) studied the development of an alluvial fan under lateral confinement, as well as the evolution process of river pattern on the fan, based on a flume experiments with a total duration of 183 h. In order to shorten the experiment duration, a series of tests are carried out in a small experiment tank. Multiple sets of input water and sediment conditions were designed to study the influence of upstream boundary conditions on the alluvial fan autogenic process. The short term response of alluvial fans to changes in input water flow and sediment supply under lateral confinement was mainly discussed.
With the increase of human activities in the valley plain, the geomorphic evolution in the region is more closely related to human production and life. Landforms change frequently due to human activities, not only interferes with the normal evolution process of alluvial fans, but also increases the probability of natural disasters. Therefore, the research on the development process of alluvial fans under the lateral confinement can help to improve the understanding of the evolution law of alluvial fans and provide effective guidance for disaster risk management in the region.
2 Experimental Methods
2.1 Experiment Setup and Materials
Given that the experimental model has a long history in alluvial fan research and provides a range of advantages unavailable to other methods of scientific investigation, it was used in this chapter to study the autogenic process of alluvial fan under various discharges and sediment feed rates (Clarke 2015).
This experiment was conducted in the experimental tank at Wuhan University. The experimental setup of the small tank is shown in Fig. 1. The tank also includes three regions: an inlet region, a deposition shelf (3 m × 0.7 m) with an adjustable gradient and a narrow sediment feeder channel (0.5 m × 0.05 m × 0.26 m). The depth was controlled by an overflow weir. The slope of deposition shelf is 0. The downstream water depth is 0.
2.2 Data Collection
One camera was fixed on the ceiling to take photographs simultaneously every 2 min in order to record planform and fluvial morphology of the fan. The fan topography was measured using a stylus profilometer with a vertical measuring resolution of 0.6 mm and a lateral measuring resolution of 10 mm. The measurement of flow velocity used the buoy method and a LH-1 water level meter developed by Wuhan University was utilized to measure water level. The geometric parameters of the tank can be extracted from the photograph or measured directly in the experiment.
Three kinds of sediment were adopted in the experiments, which are the coarse sediment, the median sediment and the fine sediment. The median size was 0.4, 0.3 and 0.17 mm correspondingly. The density of the sediment is 2600 kg/m3.
2.3 Test Conditions
7 sets of experiments were conducted in this study. Parameters in each test are shown in Table 1.
3 Influences of Water Discharge and Sediment Supply on Alluvial Fan Development
3.1 Influences of Upstream Boundary Conditions on the Sedimentation
The progradation process curve of the alluvial fan is shown in Fig. 2. When the upstream flow discharge and the sediment amount are halved separately, the progradation process of the alluvial fan slows down significantly, and the progradation curves after the slowing down are very close to each other. When the amount of water and sand in the upstream is halved at the same time, the progradation process of alluvial fan slows down further than that of water and sand separately halved.
The change process curve of alluvial fan width over time is shown in Fig. 3. The widening process of alluvial fan is less affected by the change of water and sand conditions. When the amount of sediment is halved solely, the lateral advancing speed of the alluvial fan slows down slightly due to insufficient sediment supply. When the flow is halved solely, the broadening speed increases slightly. This is because halving the flow increases the lateral flow rate by increasing the transverse slope. In addition, halving the flow rate reduces the water depth in the tank and the lateral spreading of sediment is less impeded. When the flow and the sediment are halved at the same time, the expansion speed of the alluvial fan is also slowed down, and its broadening process is very similar to that of sand halved solely. Comparing the above three situations, it can be found that when the sediment amount is small, the impact of flow change can be ignored. However, when the flow is small to a certain extent, the impact of reducing the amount of sand is even greater. It can be seen that the influence of sediment on the expansion of the alluvial fan is greater than that of the flow discharge.
Figure 4 shows the aggradation process of alluvial fans under different boundary conditions. When the sediment discharge is reduced by half, the aggradation processes of cross sections along the alluvial fan are obviously slowed down. When the flow is reduced solely, the upstream aggradation process is accelerated and the downstream aggradation process may be slowed down. When water and sand are reduced simultaneously, the aggradation process becomes more complicated. This is because halving water flow and halving sediment have different effects on the sediment accumulation process of alluvial fans. Halving sediment concentration directly reduces sediment deposition, while halving sediment concentration only changes the spatial distribution of sediment.
3.2 Influences of Upstream Boundary Conditions on the Fluvial Cycle
Table 2 shows the fluvial cycle and river channel geometry parameters under different conditions. When the upstream sediment amount is halved separately, the fluvial cycle duration of the river channel on the alluvial fan becomes longer. On the one hand, the reduction of sediment volume makes the water carrying sediment stronger and the river channel can be eroded more deeply. As the flow does not increase, the width of the channel does not change significantly. As a result, the channel morphology is more mature, the width-depth ratio is smaller. The narrow and deep channel makes the straight channel more stable and its proportion in the river pattern cycle increases. All these make the fluvial cycle to have the tendency of getting longer. On the other hand, the decrease in sediment has resulted in a slight decrease in the longitudinal slope. This causes the flow rate to decrease, which in turn reduces the Froude number Fr. These factors make the backsilt of the river easier to occur, and the fluvial cycle tends to decrease. The influence of the increase of the water depth on the river cycle is dominant, which makes the river cycle longer and causes the proportion of the straight channel to increase.
When the flow discharge is halved solely, the duration of the river cycle becomes shorter. This is also the result of a combination of two factors. The decrease of flow rate makes the longitudinal slope of alluvial fan larger. The steeper terrain makes retrogressive deposition more difficult. On the other hand, the decrease of flow makes the flow rate and water depth smaller. The sediment propulsion dynamics is weaker and more prone to silting in river channels. This tends to reduce the fluvial cycle. Finally, the effect of reducing the flow rate on the river cycle is dominant. However, due to shorter fluvial cycle, the proportion of straight channel increases.
Since halving the flow rate is contrary to the effect of halving sediment amount on the fluvial cycle, the fluvial cycle on the surface of alluvial fan changes slightly when the flow rate and sediment are halved at the same time. However, because the amount of water and sand is smaller and the energy of incision and advancing is weaker, the fluvial cycle is still slightly smaller than before. The reduced cycle length is between the values that prevail when the flow and sediment are halved separately. The proportion of the straight channel becomes greater when the amount of water and sand is halved and bigger than the value after the flow and sediment are halved separately. This is partly because the longitudinal slope of alluvial fan has increased, which makes the straight channel more sustainable. On the other hand, due to the shorter fluvial cycle of river pattern, the proportion of straight channel increases greatly. The influence of water and sediment conditions on the fluvial cycle is similar to that of Straub and Wang (2013).
4 Delayed Response of Alluvial Fan to Sudden Change of Discharge and Sediment Condition
4.1 Delayed Response of Alluvial Fan to Scour
The incoming flow and sediment conditions of natural rivers are constantly changing in natural environment. When the river system is disturbed by external changes, it will always develop towards a new equilibrium state in order to adapt to the new boundary condition, which reflects the equilibrium tendency of the alluvial system. However, the change of flow and sediment conditions is often faster, while the corresponding riverbed erosion and deposition are slower. The self-adjustment process of flow and sediment condition always falls behind the change of external conditions, which is called delayed response (Wu et al. 2007). The changing process is shown in Fig. 5. The delayed response process is divided into three phases, which are the reaction stage, the adjustment stage, and the equilibrium stage. Under some conditions, the system response is so quickly, that the reaction stage can be ignored.
Schematic diagram of adjustment process of river system characteristic variable after disturbance (Knighton 1998)
After the sand feeding rate is reduced to zero, the river bed was scoured in the upstream and the sediment was accumulated in the downstream above the alluvial fan. The sediment in the upstream channel was transported to the downstream. On the one hand, the longitudinal profile of the sediment accumulation body was flatter and the slope was decreased. On the other hand, the length of the fan was increased, which caused the progradation process to accelerate. However, the acceleration was short-lived. When the upstream scour was weakened, the sediment deposition in the downstream decreased gradually. Finally, the progradation process of the alluvial fan is gradually stopped because the upstream is no longer supplied with sediment. The progradation process of alluvium fan was accelerated firstly and then stabilized under the erosion of clear water, which corresponds to the adjustment stage of delayed response.
The delayed response of fluvial fan is quantitatively analyzed by the rate law taking example of the thickness of sediment accumulation.
where:
- y:
-
the characteristic variable.
- ye:
-
the equilibrium value of the characteristic variable;
- t:
-
time.
β is a coefficient which is positive, whose dimension is 1/T. When β is larger, the adjustment rate of riverbed characteristic variable is faster, therefore it is called the adjustment speed parameter of river course.
According to the calculation condition and characteristic, three patterns are put forward in the solution of the rate law of delayed response, which are the universal integration pattern, the single step analytic pattern, and the multistep recursive pattern. In this study, the flow and sediment remained unchanged after they were changed abruptly, until the alluvial fan reaches a new equilibrium. The reaction of the fan had only one period, so the single step analytic pattern was applied to solve the rate law of delayed response.
The single step analytic pattern can be computed as:
where:
- y1:
-
the computed value of characteristic variable after a time interval
- ye1:
-
the equilibrium value of characteristic variable after a time interval
- y0:
-
the initial value of characteristic variable before the disturbing
- ∆t:
-
the duration of the time interval.
Take the siltation thickness as the characteristic variable, \(y_{1}\) is siltation thickness after a time interval, \(y_{0}\) is the initial siltation thickness, ye1is the equilibrium value of the siltation thickness, ∆t is the time interval between the adjacent measurements of topography, \(\beta\) is the adjustment parameter for siltation thickness.
For the alluvial fan test in a small tank, we selected all three sections of the upstream, middle, and downstream of the alluvial fan for sediment thickness statistics (x = 0.25 m section, x = 0.75 m section and x = 1.25 m section) for each working condition. In the midstream and downstream sections, we calculate the average siltation thickness of the section. In the upstream section, we calculate the siltation thickness of the channel bed.
The comparison between measured results and simulated results of sediment thickness response process is in Fig. 6. It can be seen that by choosing appropriate parameters, we can use the rate law to better describe the actual response process of the deposition thickness. The β value of the upstream section is often larger than that of the middle and lower reaches, indicating that the adjustment rate of the upstream deposition thickness is greater. This is because the flushing caused by clear water in the upper reaches is the most severe, and the river bed needs to be adjusted more to reach a new equilibrium form. Further downstream, the sediment content of the flow recovers. The resulting erosive intensity gradually weakens and the bed response process becomes slower.
4.2 Delayed Response of Alluvial Fans to Discharge Variation
In the small sink operating conditions W3S1 (Qs halved, Qw periodically changing), the upstream flow rate periodically varies between 1.11 × 10−4 and 0.56 × 10−4 m3/s. Through this design, we studied the continuous response process of the deposition thickness after continuous discharge changes. Two changes in upstream discharge are generalized into two separate response periods. The solution to the variability equation still uses the single step analytic pattern. At the x = 0.25 m section, the sedimentation thickness of the riverbed surface over time is shown in Fig. 7. We use the rate law to simulate the two stages of the delayed response process, and the calculated results agree well with the measured data.
Simulation of delayed response of deposition thickness after flow change is in Fig. 7. The calculation results show that the two delayed response speeds of the alluvial fan thickness are significantly different when the upstream discharge rate changes continuously. At the first mutation, when the discharge increased from 0.56 × 10−4 to 1.11 × 10−4 m3/s, the bed surface response speed is very fast, β value is about 5. At the second mutation, the discharge decreased from 1.11 × 10−4 to 0.56 × 10−4 m3/s, and the riverbed response speed slowed down significantly. The β value is only 1.3. On the one hand, this is related to the response of alluvial fans. When the discharge suddenly increases, the bed is flushed and undercut. The undercut area is concentrated in the channel, making the bed thickness more variable. When the discharge suddenly drops, the channel is quickly filled with sediment. The water flow covers most of the alluvial fan area, making the sediment deposition range significantly larger and the deposition thickness change naturally smaller. On the other hand, this may be related to the different state of the alluvial fan before the two discharge mutations. Before the first discharge mutation, the alluvial fan has a smaller size and a larger vertical slope. The evolution process is more sensitive to changes in water and sediment conditions. Before the second discharge mutation, the alluvial fan slope became very small and the velocity was slow. This means that alluvial fans encounter more terrain resistance when responding, and are more resilient to changes in the external environment.
5 Conclusions
In this chapter, the influence of water discharge and sediment concentration on the alluvial fan autogenic process is studied experimentally. Besides, alluvial fan’s delayed response to external disturbances is demonstrated by changing discharge and sediment feed rate during experiments. Conclusions are as follows:
-
(1)
Fan progradation process becomes slower when either discharge or sediment feed rate is reduced. When discharge and sediment feed rate are reduced simultaneously, the process is much slower. The widening process of alluvial fans is less sensitive to water discharge and sediment feed rate variation.
-
(2)
When sediment discharge is reduced, aggradation processes slows down along the fan. When discharge is reduced, spatial distribution of sediment varies along the fan, while the overall sedimentation amount stays the same. The upstream aggradation process accelerates while downstream aggradation process slows down.
-
(3)
The reaction of the fluvial cycle and channel morphology to water discharge reduction is the opposite of that to sediment reduction. When sediment is reduced, the water depth in the river channel increases and the velocity decreases, the river cycle becomes longer and the proportion of straight channel increases. When discharge is reduced, the river cycle becomes shorter. Although the duration of straight channel doesn’t increase, the proportion of straight channel increases. When discharge and sediment feed rate decrease simultaneously, the longitudinal slope of alluvial fans and flow velocity increases, while the river cycle becomes slightly shorter. The duration of straight channel is longer, which makes its proportion in the river cycle increase greatly.
-
(4)
The rate law can be employed to describe the actual response process of the deposition thickness. Calculated results demonstrate that the response speed of upper-fan siltation thickness is bigger than that of mid-fan and lower-fan. The response speed of fine-sand alluvial fan is larger than that of coarse-sand fan. The response speed of deposition thickness to water discharge increase is larger than that associated with the decrease of the water discharge. The fan response rate to external disturbance is not only related to the response mode of alluvial fans, but also related to the different state of the alluvial fan before the disturbance.
References
Caldwell, R. L., & Edmonds, D. A. (2014). The effects of sediment properties on deltaic processes and morphologies: A numerical modeling study. Journal of Geophysical Research: Earth Surface, 119(5), 961–982.
Clarke, L. E. (2015). Experimental alluvial fans: advances in understanding of fan dynamics and processes. Geomorphology, 244, 135–145.
Dirszowsky, R. W., & Desloges, J. R. (2004). Evolution of the moose lake delta, british Columbia: Implications for Holocene environmental change in the Canadian Rocky Mountains. Geomorphology, 57(1), 75–93.
Hashimoto, M., Fukushima, Y., & Fukahata, Y. (2011). Fan-delta uplift and mountain subsidence during the Haiti 2010 earthquake. Nature Geoscience, 4(4), 255–259.
Knighton, D. (1998). Fluvial forms and processes: A new perspective [M]. New York: Wiley.
Nemec, W., & Steel, R. J. (1988). What is a fan delta and how do we recognize it. Fan Deltas: Sedimentology and Tectonic Settings, 3–13.
Ravazzi, C., Marchetti, M., Zanon, M., et al. (2013). Lake evolution and landscape history in the lower Mincio River valley, unravelling drainage changes in the central Po Plain (N-Italy) since the Bronze Age. Quaternary International, 288, 195–205.
Straub, K. M., & Wang, Y. N. (2013). Influence of water and sediment supply on the long-term evolution of alluvial fans and deltas: Statistical characterization of basin-filling sedimentation patterns. Journal of Geophysical Research: Earth Surface, 118, 1602–1616.
Taha, Z. P., & Anderson, J. B. (2008). The influence of valley aggradation and listric normal faulting on styles of river avulsion: a case study of the Brazos River, Texas, USA. Geomorphology, 95(3), 429–448.
Van Dijk, M., Kleinhans, M. G., Postma, G., et al. (2012). Contrasting morphodynamics in alluvial fans and fan deltas: effect of the downstream boundary. Sedimentology, 59(7), 2125–2145.
Wu, B. S., Wang, G. Q., & Xia, J. Q. (2007). Case study: Delayed sedimentation response to inflow and operations at sanmenxia dam. Journal of Hydraulic Engineering ASCE.
Zhang, X. F., Wang, S. Q., Wu, X., Xu, S., & Li, Z. Y. (2016). The development of a laterally confined laboratory fan delta under sediment supply reduction. Geomorphology, 257, 120–133.
Acknowledgements
The authors wish to thank Xi Wu, Shun Xu, Zhang yong Li for their group effort to make this experiment possible. We also thank Shi Ren, Wenting Yang, Yi Hu, Mengji Qi for their language help that greatly improved the readability of the chapter. This study was supported by the National Key R&D Program of China (2016YFC0402305, 2016YFC0402309, 2017YFC0405304, 2017YFC0405306), the Special fund for basic scientific research business of central public research institutes (Grant No. CKSF2017010/HL, CKSF2015049/HL), Scientific scheduling research project of Three Gorges Reservoir (SXSN/4277), the National Natural Science Foundation of China (Grant No. 51,609,009).
Author information
Authors and Affiliations
Editor information
Editors and Affiliations
Rights and permissions
Copyright information
© 2020 Springer Nature Singapore Pte Ltd.
About this paper
Cite this paper
Yuan, Y., Zhao, J., Luo, X., Zhou, Y., Wang, S. (2020). Laboratory Study of Short Term Response of Alluvial Fans to Changes in Input Water Flow and Sediment Supply Under Lateral Confinement. In: Nguyen, K., Guillou, S., Gourbesville, P., Thiébot, J. (eds) Estuaries and Coastal Zones in Times of Global Change. Springer Water. Springer, Singapore. https://doi.org/10.1007/978-981-15-2081-5_39
Download citation
DOI: https://doi.org/10.1007/978-981-15-2081-5_39
Published:
Publisher Name: Springer, Singapore
Print ISBN: 978-981-15-2080-8
Online ISBN: 978-981-15-2081-5
eBook Packages: Earth and Environmental ScienceEarth and Environmental Science (R0)