Abstract
A low Reynolds number hypersonic flow boundary layer tripping on a conical body is studied. The experiments are performed in a conventional shock tunnel at conditions providing flight realistic combination of Reynolds number and Mach number. It is observed that it is difficult to trip such a flow even with diamond trip heights as much as five times the local boundary layer thickness. Heat flux measurements over the cone indicate that though trip causes local disturbance in the flow field, the boundary layer seems to relaminarize toward the end of the cone.
Access provided by Autonomous University of Puebla. Download conference paper PDF
Similar content being viewed by others
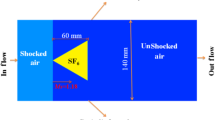
1 Introduction
The aerodynamics of vehicles moving at hypersonic speeds is always affected by the boundary layer behavior at such speeds. In most cases, for realistic vehicle length scales, boundary layer transition from laminar to turbulent is a critical phenomenon that can drastically affect the heat flux to the vehicle wall. Though this increase in heat flux when the boundary layer goes to turbulent needs to be avoided, in certain cases having a turbulent boundary layer becomes necessary. This is the case for SCRAMJET inlets where a turbulent boundary layer is preferred to avoid the problem of laminar separation due to shock impingement that can lead to unstart of the engine [1]. For this reason, such inlet studies work on tripping the boundary layer into transition to get the required turbulent boundary layer [1, 2].
Tripping of boundary layer in hypersonic flow has been studied in some detail using either distributed roughness or isolated three-dimensional elements [3]. These studies show that effectiveness of the trips is strongly dependent on the boundary layer edge Mach number Me, and few studies address this problem at hypersonic values of Me. The general trend is that the higher the Me, the more drastic the trip height needs to be, to be critical at all. This poses an issue on the effectiveness of the trip at hypersonic Me where the trip height to boundary layer height ratio k/δ requirements can be as high as 2 [2].
Apart from Me, free-stream Reynolds number also affects this phenomenon though it is usually taken into account as the parameter Rek, which is the Reynolds number based on trip height and conditions at the trip height. Again at hypersonic Me, the Rek requirements are much higher than for supersonic Me [3] which makes for further complicated correlations that need more data at hypersonic speeds. To add to that, most studies have high enough Re∞, or the unit length Reynolds number in the free stream to study the transition caused by roughness, but very little data is produced at the actual Re∞ that vehicles moving at hypersonic speeds at the corresponding altitudes may have. Figure 1 shows a plot redrawn from Heiser and Pratt [4], which shows an example of a flight corridor, for a range of dynamic pressures that the vehicle is allowed to experience in flight, as the shaded region. On that, constant Re∞ curves have been overlapped to show that most hypersonic flight has low Re∞ to contend with at such altitudes. This makes hypersonic boundary layer tripping studies at lower, more realistic Reynolds numbers, imperative.
Flight corridor for hypersonic vehicle taken from Heiser and Pratt [4] with constant Re∞ curves overlapped
With this background, the current study aims to study tripping of such a condition at free-stream Mach number of 8 with a Re∞ of 3.2 million/m. This condition falls in the flight corridor shown in Fig. 1, though only in terms of Mach number and unit length Reynolds number. This corresponds to Mach 8 flow at 30 km altitude. An axisymmetric model is chosen to further provide a challenging (though still realistic) scenario as compared to flat plate, the latter being more well studied as well as easier to trip the flow on. Heat flux measurements have been employed to track the tripped boundary layer behavior in a hypersonic shock tunnel at LHSR.
2 Experimental Setup
2.1 Test Model
The experiments on hypersonic boundary layer tripping have been performed on a 12 deg. half-angle right circular cone with 800 mm axial length. The cone is shown in Fig. 2 along with an example of fixing trips on the cone surface. The cone has an array of flush-mounted MACOR inserts on the top surface which have platinum thin-film sensors mounted onto them. Data from the sensors on the second half of the cone have been used to study the boundary layer behavior. Wall heat flux measurements have not been made in the vicinity of the trips.
The cone, made of Duralumin, has a surface smooth finish and the cone tip is sharp, made of stainless steel with a machining tolerance of ±0.05 mm. This study is focused only on tripping the boundary layer using diamond trips as they have been found to work best for such flows [1]. Cubes of edge lengths 1.5 mm, 3 mm, 4 mm, and 5 mm have been used to create different heights of the trips at the appropriate locations. To fix the trip to any location, a small piece of Kapton tape, that is, 150 μm thick, is attached to the surface of the cone, and the required trip is fixed onto the tape using a thin layer of super glue such that there is no spilling of the glue around the trip. By using this method trips could be placed at any location on the cone surface.
2.2 Experimental Facility and Test Conditions
All experiments were done in the hypersonic shock tunnel 4 (HST4) at LHSR. The facility is a gas-driven shock tunnel that has a nozzle exit diameter of 1 m with interchangeable Mach 7 and 8 throat inserts. The shock tube diameter is 165 mm, the driven length is 10 m, and the driver is 3 m long. A sample signal from the nozzle inlet reservoir sensor and the corresponding pitot sensor signal inside the tunnel for a condition used in the current study are shown in Fig. 3.
Figure 3 shows the reservoir supply pressure to be steady for more than 2 ms, and the pitot pressure shows the test time of around 1.2 ms. The flow parameters obtained for this condition are tabulated in Table 1.
3 Results and Discussion
3.1 Case Without Trips
The main measurement for the present study is the wall heat flux estimated by employing the thin-film sensors. This provides a good indication if the boundary layer goes into transition and turbulent heat flux would be much higher than its laminar counterpart. Figure 4 shows the heat flux measurements on the 800 mm cone for a shot without the trips, and clearly the given condition has laminar boundary layer. The number of heat flux data points per shot is limited to 12 given a change in equipment during the course of the campaign.
3.2 Case with Trips
For boundary layer flow in a shock tunnel with hypersonic Me, it has been found that k/δ of roughly 2 is required for the trip to be effective and of roughly 1 to be critical [2]. This is a trend that is commonly observed [3] for flat plates. But for conical bodies very less data is present at hypersonic Me with low Reynolds numbers.
The HIFiRE 1 conical model was tested at Mach 6 with a single trip element at a low Reynolds number [5], but the Me was supersonic such that a k/δ of 0.5 was sufficient for effective tripping. In this context, various k/δ ratios were tried, starting with more than critical values reported in the literature. Figure 5 shows the behavior of the wall heat flux in the presence of various k/δ ratios for a single element and a trip with three elements of diamond trip placed in line perpendicular to the flow direction. The separation of the elements is more than three times the cube face diagonal for the trip as proposed by Whitehead [6] (Fig. 2 shows lower separation case, which is from earlier shots). From Fig. 5 it is not very clear if multielement trip is much different from single 3D element. Also for a k/δ comparable to the case of flat plate [2], there looks to be a lot of fluctuation of heat flux between 300 mm and 600 mm, but the heat flux does not tend toward turbulent values downstream. This is also clear from the laminar nature of the heat flux signal toward the end of the cone which is not observed to be unsteadily fluctuating, as in the case of transitional or turbulent boundary layer. Next, the trip heights were increased to beyond two times the local boundary layer height; results of the heat flux are shown in Fig. 6.
It can be readily observed from Fig. 6 that trip height criterion is no more a critical factor at lower Reynolds numbers. Though the heat flux shows a lot of fluctuations in the midsection of the cone, all cases settle down to a narrow range of heat flux which hints at relaminarization of the boundary layer. The higher k/δ ratio cases show lower heat flux in the midsection owing to the greater spread of the vortex wake that misses the sensors directly in line with the central trip element. Finally, for all cases there is no evident departure from orderly temperature rise, with no heat spikes or fluctuations to point toward turbulent spots or trip wake breakdown, also hinting at no clear transitional behavior in the boundary layer.
4 Conclusion
A study on tripping of boundary layer with high edge Mach number and low free-stream Reynolds number is carried out on an axisymmetric body. It was observed that the trips, earlier reporter to be effective for flat plate cases, cause only local disturbance to the boundary layer in the case of this conical body. Increasing trip height to as much as five times the local boundary layer height does not lead to clear transition. In fact, much of the data hints at relaminarization toward the rear end of the cone. This behavior may be attributed to more stable trip wake at higher Mach numbers along with the disturbance relaxation caused by the axisymmetric flow nature, both of which are known effects in this area of investigation. This study brings forward the issues in tripping hypersonic boundary layer over axisymmetric bodies at flight realistic Reynolds numbers. More investigations must be conducted in different tunnels at such conditions to clarify such phenomenon and address it for applications such as the SCRAMJET inlets where these studies become exceedingly important.
References
S.A. Berry, A.H. Auslender, A.D. Dilley, J.F. Calleja, Hypersonic boundary-layer trip development for hyper-X. J. Spacecr. Rocket. 38 (2001)
D.J. Wise and M.K. Smart, Forced transition of hypervelocity boundary layers, in 18th AIAA/3AF International Space Planes and Hypersonic Systems and Technologies Conference , 2012
S.P. Schneider, Effects of roughness on hypersonic boundary layer transition. J. Spacecr. Rocket. 45 (2008)
W.H. Heiser and D.T. Pratt, Hypersonic Airbreathing Propulsion, (AIAA, 1994), pp. 37–39
Roger L. Kimmel, Roughness considerations for the HIFiRE-1 vehicle, in AIAA 38th Fluid Dynamics Conference and Exhibit, 2008
Allen H. Whitehead Jr., Flow-field and drag characteristics of several boundary-layer tripping elements in hypersonic flow, NASA TN D-5454
Author information
Authors and Affiliations
Editor information
Editors and Affiliations
Rights and permissions
Copyright information
© 2019 Springer Nature Switzerland AG
About this paper
Cite this paper
Singh, T., Reddy, K.P.J. (2019). Hypersonic Boundary Layer Tripping to Turbulence on a Conical Body. In: Sasoh, A., Aoki, T., Katayama, M. (eds) 31st International Symposium on Shock Waves 2. ISSW 2017. Springer, Cham. https://doi.org/10.1007/978-3-319-91017-8_110
Download citation
DOI: https://doi.org/10.1007/978-3-319-91017-8_110
Published:
Publisher Name: Springer, Cham
Print ISBN: 978-3-319-91016-1
Online ISBN: 978-3-319-91017-8
eBook Packages: EngineeringEngineering (R0)