Abstract
Penetration of the cranium typically results in secondary projectile formation (bone and primary projectile fragments); the formation of a temporary cavity and resulting increase in internal pressure which is enhanced by the confined space of the cranium and the stiff bones of the skull [1]. This enhanced internal pressure can result in fractures of the base of the cranium, because it is not as strong as other parts of the skull [1]. Radial fractures are typical at the impact point, but concentric fractures can also occur connecting the radial cracks due to flexing of the skull. This through-thickness failure is commonly referred to as cratering [2–4]. Bullet wipe is reported on the scalp in forensic case studies e.g. [2–4]. The fact that bullet wipe can be identified to specific bullets is of interest forensically when investigating attempted murder or murder incidents in civilian and military scenarios [5].
This section draws heavily on a published paper and is reproduced with permission by Springer: Carr DJ, Lindstrom A, Jareborg A, Champion S, Waddell JN, Miller D, Teagle M, Horsfall I, Kieser J. Development of a skull/brain model for military wound ballistics studies. Int J Leg Med. 2014. doi:10.1007/s00414-014-1073-2 (License number: 3563580567511, 7 Feb 2015).
Access provided by Autonomous University of Puebla. Download chapter PDF
Similar content being viewed by others
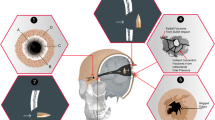
Keywords
These keywords were added by machine and not by the authors. This process is experimental and the keywords may be updated as the learning algorithm improves.
1 Introduction
Penetration of the cranium typically results in secondary projectile formation (bone and primary projectile fragments); the formation of a temporary cavity and resulting increase in internal pressure which is enhanced by the confined space of the cranium and the stiff bones of the skull [1]. This enhanced internal pressure can result in fractures of the base of the cranium, because it is not as strong as other parts of the skull [1]. Radial fractures are typical at the impact point, but concentric fractures can also occur connecting the radial cracks due to flexing of the skull. This through-thickness failure is commonly referred to as cratering [2–4]. Bullet wipe is reported on the scalp in forensic case studies e.g. [2–4]. The fact that bullet wipe can be identified to specific bullets is of interest forensically when investigating attempted murder or murder incidents in civilian and military scenarios [5].
In combat, head wounds are recognised as accounting for substantial mortality and morbidity; the head accounts for 9 % of the human body, 20 % of penetrating combat injuries and its wounding accounts for 50 % of combat deaths [6]. The major cause of injury during warfare is from fragments and therefore, military helmets are designed to protect from fragments; when they are perforated it is most commonly by high kinetic energy projectiles e.g. high-velocity rifle bullets or fragments due to blast (secondary blast) [7–10].
While a number of models for bullet/fragment-head impact recreations have been proposed, these are not anatomically correct e.g. [3, 4, 11]. The models include a polyurethane sphere filled with 10 % gelatine conditioned at 4 °C [3], a cylinder composed of a plastic/ wood/polymer outer filled with gelatine [4] and polymeric spheres containing 10 % gelatine [11]. These models reportedly compared well to actual gunshot injuries or secondary blast injuries; however, it would not be possible to mount a helmet on them.
Literature describing ballistic rate impacts on human skulls with or without a simulated brain is sparse [2, 12, 13]. In 1970, Miller reported on the penetration of skulls by steel cubes and spheres, this government report is not accessible in the open literature. However, some of Miller’s data are mentioned in another report which describes a model for projectiles impacting skulls [12]. The fact that dried skulls and fresh skull caps performed differently, and the need to consider the additional contribution of hair and the scalp in live human impacts with respect to these projectiles were noted [12]. Some early work that is easily accessible was published by Watkins et al. [13]. Watkins used dried AsiaticFootnote 1 skulls filled with 20 % gelatine and covered with two layers of chamois covered in gelatine (no details of the chamois were provided and presumably the gelatine used was 20 % by mass). Watkins’ work included a series of nine impacts; the majority of this work involved ball bearings at velocities up to ~1000 m/s, but Watkins did note one head was tested with a 7.62 mm ball round (no further details given) at 750 m/s (Table 15.1).
Reports on penetrating ballistic head injuries in the forensic literature are dominated by case studies of suicides; the penetrating ammunition usually being .22 rimfire or shotgun. There are some exceptions that report outcomes of interest to the current work [2, 14] (Table 15.2). Analysis of skull fracture patterns resulting from self-inflicted gunshot injury identified key patterns of injury including the formation of linear fractures and fragmentation with reference to the anatomical structures of the skull [2]. A further interesting study that includes a high-velocity rifle impact considers the reconstruction of the head after injury and does not discuss skull fractures [15].
The aim of this work summarised here was to develop an anatomically correct skull and brain model for use in military wound ballistic studies incorporating helmets.
2 Materials and Methods
Anatomically correct polyurethane skulls were manufactured from rapid prototype data obtained by 3D mapping of both the internal and external surfaces of a human skull (Fig. 15.1a). The polyurethane used to manufacture the skulls had a hardness of 85 Shore D, a tensile strength of 70 MPa and an impact strength of 10 kJ/m2. Tensile strength of bone from human skulls has been reported to be 225 MPa. However, these properties are reported for quasi-static rates; as testing conducted on the polymeric skulls was at ballistic strain rates, the difference in these mechanical properties is not critical, although further work should explore these differences at the appropriate loading rates.
A two-part silicone mould made from a human brain was used to cast 10 % (by mass) gelatine brains (Fig. 15.1b); 10 % gelatine was chosen as the density was similar to reported values for human brain tissue [16]. A thin polymeric bag was inserted into the brain mould and gelatine poured in, the gelatine was allowed to set for 24 h and then conditioned at 4 °C for 24 h [17, 18]. The polymeric bag was softened by the warm gelatine and thus excellent definition of the mould surface was achieved; additionally, the polymeric bag acted as a representation of the meninges (Fig. 15.1c).
Models were shot using 7.62 × 39 mm M43 ball (Chinese, mild steel core, Factory 71 made in 1984) ammunition at a range of 10 m from a No. 3 Enfield proof mount fitted with an AK-47 barrel (Table 15.3). Projectile velocity was tracked using a Weibel Doppler, and impacts filmed using a Phantom V12 high-speed video; projectiles were soft-captured after model perforation using a PermaGelTM block.
Fractured skull pieces were collected and weighed post ballistic testing. Skull pieces showing signs of bullet wipe and bullets soft-captured after skull perforation were subjected to further analysis. The presence and distribution of inorganic residues was confirmed by using scanning electron microscopy coupled with energy-dispersive x-ray spectroscopy (SEM EDS).
3 Results and Discussion
Mean projectile impact velocity was 675 m/s (s.d. = 6 m/s). All skulls were perforated as intended. Bullet impact wounds demonstrated cratering damage with radial cracks. Inorganic residues representative of bullet wipe were found at impact sites. Results are presented in Table 15.3 and a typical impact sequence is presented in Fig. 15.2.
Without a ‘brain’ (skulls 1 and 2), minimal damage to the skull occurred due to the lack of development of a temporary cavity when a projectile passes through the brain and the associated increase in pressure in the cranial vault which leads to multiple fractures of the skull [1]. Damage was confined to the impact and exit sites and associated radial cracking. Models comprising of a skull and brain (skulls 3–6) fragmented into multiple pieces of diverse size and shape. These models were reconstructed and compared to data in the forensic anthropology literature. Probably, the most useful comparisons can be found among the case studies reported by Fenton et al. [2] and Betz et al. [14], although the types of ammunition used were reportedly different to that in the current paper. Of particular interest were the complete perforation of the skull, the relatively large pieces of fractured bone, the bilateral fractures occurring adjacent to buttresses, the fractures to the inferior and posterior of the skull and the ejected brains. All of these features were observed in the skull and brain models developed in the current paper. Thus, the presence of a ‘brain’ resulted in different fracture patterns (compared to shooting a skull only) that related well to previously proposed models and to data reported in the literature of actual incidents. As the skulls used in the current work were anatomically correct, the various thicknesses of the polymer varied at different points in the skull mimicking a human skull. Post-testing analysis identified that fractures occurred at weaker areas dictated by actual anatomical features. In particular, fractures were influenced by the various buttresses within the skull, as reported in case studies of actual events, e.g. [2]. The amount of gelatine used in the skull to represent the brain affected the severity of the fracture patterns. Use of an anatomically correct brain (skull 5) resulted in the least severe result; although the skull was extensively fractured, the cranial base remained intact. Completely filling the cranial cavity with either gelatine or a gelatine brain and water combination resulted to similar fracture patterns (skulls 3, 4, 6), which were both more severe than with the gelatine brain alone. In particular, it was noticeable that a fully filled cranial cavity resulted in fractures to the inferior and a more catastrophic result.
4 Conclusions
An anatomically correct model for studying military wound ballistic and secondary blast events to the human skull and brain has been developed. The advantage of using an anatomically correct skull model in combination with a gelatine brain has been demonstrated by the fracture patterns obtained which compare favourably to the limited reports of actual high velocity rifle wounds to the head. Comparisons with complex secondary blast fragments has not been conducted. Impact and exit wounds were as expected; evidence of cratering and radial cracking was observed. Bullet-wipe was observed at the impact sites. Completely filling the cranial cavity with either gelatine or a gelatine brain and water combination resulted in similar fracture patterns.
Notes
- 1.
Watkins’ terminology.
References
Karger B. Penetrating gunshots to the head and lack of immediate incapacitation I. Wound ballistics and mechanisms of incapacitation. Int J Leg Med. 1995;108:53–61.
Fenton TW, Stefan VH, Wood LA, Sauer NJ. Symmetrical fracturing of the skull from midline contact gunshot wounds: reconstruction of individual death histories from skeletonized human remains. J Forensic Sci. 2005;50:1–12.
Thali MJ, Kneubuehl BP, Zollinger U, Dirnhofer R. The skin–skull-brain model: a new instrument for the study of gunshot effects. Forensic Sci Int. 2002;125:178–89.
Lieske K, Janssen W, Kulle K-J. Intensive gunshot residues at the exit wound: and examination using a head model. Int J Leg Med. 1991;104:235–8.
Kieser DC, Carr DJ, Girvan L, Leclair SCJ, Horsfall I, Theis J-C, Swain MVJAK. Identifying the source of bullet wipe—a randomised blind trial. Int J Leg Med. 2013. doi:10.1007/s00414-0130874-z.
Carey ME. Learning from traditional combat mortality and morbidity data used in the evaluation of combat medical care. Mil Med. 1987;152:6–13.
Breeze J, Gibbons AJ, Shieff C, Banfield G, Bryant DG, Midwinter MJ. Combat-related craniofacial and cervical injuries: a 5-year review from the British military. J Trauma. 2011;71:108–13.
Spalding TJW, Stewart MPM, Tulloch DN, Stephens KM. Penetrating missile injuries in the Gulf war 1991. Br J Surg. 1991;78:1102–4.
Eskridge SL, Macera CA, Galarneau MR, Holbrrok TL, Woodruff SI, MacGregor AJ, Morton D, Sahaffer RA. Injuries from combat explosions in Iraq: injury type, location, and severity. Injury. 2012;43:1678–82.
Lewis EA. Between Iraq and a hard plate: recent developments in UK military personal armour. In: IPAC, editor. Personal armour systems symposium 2006 (PASS2006). Leeds: The Royal Armouries, 18–22 Sep 2006.
Schyma C, Greschus S, Urbach H, Madea B. Combined radiocolour contrast in the examination of ballistic head models. Int J Leg Med. 2012;126:607–13.
Sturdivan LM, Bexon R. A mathematical model of the probability of perforation of the human skull by a ballistic projectile. Aberdeen Proving Ground: Chemical Systems Laboratory; 1981.
Watkins FP, Pearce BP, Stainer MC. Physical effects of the penetration of head simulants by steel spheres. J Trauma. 1988;28(1 Supplement):S40–54.
Betz P, Steiefel D, Hausmann R, Eisenmenger W. Fractures at the base of the skull in gunshots to the head. Forensic Sci Int. 1997;86:155–61.
Hejna P, Safr M, Zatopkova L. Reconstruction of devastating head injuries: a useful method in forensic pathology. Int J Leg Med. 2011;125:587–90.
Barber TW, Brockway JA, Higgins LS. The density of tissues in and about the head. Acta Neurol Scand. 1970;46(1):85–92.
Jussila J. Preparing ballistic gelatine – review and proposal for a standard method. Forensic Sci Int. 2004;141:91–8.
Mabbott A, Carr DJ, Champion S, Malbon C, Tichler, C. Comparison of 10 % gelatine, 20 % gelatine and Perma-Gel for ballistic testing. In: International Symposium on Ballistics, Freiberg, 22–26 April 2013.
Acknowledgments
This work was internally funded by The Impact and Armour Group at Cranfield University.
Author information
Authors and Affiliations
Corresponding author
Editor information
Editors and Affiliations
Rights and permissions
Copyright information
© 2016 Springer International Publishing Switzerland
About this chapter
Cite this chapter
Carr, D.J., Champion, S. (2016). Military Wound Ballistics Case Study: Development of a Skull/Brain Model. In: Bull, A., Clasper, J., Mahoney, P. (eds) Blast Injury Science and Engineering. Springer, Cham. https://doi.org/10.1007/978-3-319-21867-0_15
Download citation
DOI: https://doi.org/10.1007/978-3-319-21867-0_15
Published:
Publisher Name: Springer, Cham
Print ISBN: 978-3-319-21866-3
Online ISBN: 978-3-319-21867-0
eBook Packages: MedicineMedicine (R0)