Abstract
Enterococcus faecalis, a commensal of the intestinal tract of humans and animals is of great significance as leading opportunistic pathogen, and also prevalent in oral diseases, such as endodontic infections, as well as the healthy oral cavity. To investigate the potential of oral E. faecalis to constitute a reservoir of antibiotic resistance, isolates from supragingival plaque/saliva and from endodontic infections were screened regarding their resistance to selected antibiotics in comparison to nosocomial and food isolates.
70 E. faecalis isolates were analyzed with PCR regarding their equipment with the resistance genes tetM, tetO, ermB, ermC, vanA, vanB and blaTEM. Additionally, they were tested for their phenotypic resistance to doxycycline, azithromycin, rifampicin, amoxicillin and streptomycin using the Etest.
High percentages of the plaque/saliva, nosocomial and food isolates were resistant to doxycycline and azithromycin, particularly plaque/saliva isolates (81%) and nosocomial isolates (73.3%) showed resistance to doxycycline, significantly more than among the food and endodontic isolates. Rifampicin resistance was widespread among isolates from plaque/saliva (52.4%), endodontic infections (50%) and nosocomial infections (40%); all isolates were susceptible to amoxicillin and all oral isolates to high-level streptomycin. TetM genes were detected in the majority of all isolates and ermB genes were present in many nosocomial and plaque/saliva isolates. Thirty percent of the endodontic isolates and 53% of the nosocomial isolates were equipped with blaTEM genes.
The results suggest that the oral cavity can harbor E. faecalis strains with multiple resistances against different antibiotics and thus be regarded as a potential source of resistance traits.
Access provided by CONRICYT-eBooks. Download chapter PDF
Similar content being viewed by others
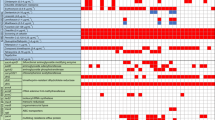
Keywords
1 Introduction
Enterococcus faecalis is of great importance as a leading opportunistic pathogen causing nosocomial infections (Arias and Murray 2012). Frequent infections include endocarditis, meningitis, urinary tract infections, wound infections and neonatal infections (Murray 1990). Furthermore, this bacterial species is of significance for the field of oral diseases. Even though E. faecalis is normally only found in low numbers in oral sites of healthy individuals (Portenier et al. 2003), its prevalence in the oral cavity substantially increases in many oral diseases, e.g. gingivitis, periodontitis, caries, endodontic infections and especially post-treatment apical periodontitis, where it is considered a main pathogen associated with endodontic failure (Dahlen et al. 2000; C. M. Sedgley et al. 2005b; C. Sedgley et al. 2006; Souto and Colombo 2008; Sun et al. 2009; Kouidhi et al. 2011; Anderson et al. 2013).
What contributes to its relevance as an opportunistic pathogen is the fact that E. faecalis is intrinsically resistant to several antibiotics and can harbor different acquired resistance traits (Van Tyne and Gilmore 2014). Despite its pathogenic potential, E. faecalis is typically found as a commensal in the gastrointestinal tract of humans and many animals (Arias and Murray 2012). Specific strains have been used as probiotics and some strains are found in food where they are responsible e.g. for the ripening of certain cheeses (Fisher and Phillips 2009; Franz et al. 2011; Hammerum 2012).
Its proficiency in efficiently acquiring and spreading genetic elements via horizontal gene transfer as well as its common ability to form biofilms have been well characterized for E. faecalis (Paulsen et al. 2003; Duggan and Sedgley 2007; Manson et al. 2010; Palmer et al. 2010; Paganelli et al. 2012) . Our group was able to demonstrate that E. faecalis originating from cheese is able to integrate into the oral biofilm in vivo (Al-Ahmad et al. 2010) and recently authors confirmed that E. faecalis can colonize a multi-species biofilm in a supragingival biofilm model (Thurnheer and Belibasakis 2015). These findings highlight the possibility of the oral cavity to constitute a reservoir for the antibiotic resistance genes of E. faecalis as well as other traits of clinical concern that could be spread within the oral biofilm. The information on antibiotic susceptibility characteristics of oral E. faecalis isolates is scarce apart from studies of endodontic isolates. In a previous study we investigated the virulence factors as well as the capacity for biofilm formation and susceptibility to some antibiotics of the E. faecalis isolates from different sources with a focus on the biofilm formation in association with virulence factors (Anderson et al. 2015). The aim of the present study was to take this analysis further focusing on selected antibiotic resistance genes as well as additional relevant phenotypic resistance to assess whether these strains can represent a reservoir for antibiotic resistance traits. For the determination of the antibiotic susceptibility, widely used phenotypic tests as well as PCR were applied (Amsler et al. 2010; Jorgensen and Ferraro 2009; Tenover et al. 1996), which give an insight into the bacterial strains’ equipment with resistance traits. This is advantageous in the attempt to determine if strains possessed genes that could be spread even without expressing the genes themselves.
2 Materials and Methods
2.1 Bacterial Isolates
A total of 70 E. faecalis strains, 20 isolates from endodontic retreatment, 21 oral isolates (saliva and supragingival plaque), 14 food isolates (raw milk) and 15 isolates from nosocomial infections (9 urinary tract infections, 1 wound infection, 1 intraoperative swab, 1 drainage secretion, 1 intraabdominal aspirate, 1 blood culture and 1 central venous catheter) were analyzed for their antibiotic susceptibility. The oral and endodontic isolates were gathered from 2011–2014 in the Department of Operative Dentistry and Periodontology (Medical Center - University of Freiburg, Faculty of Medicine, University of Freiburg, Germany). The plaque and saliva samples were taken from healthy individuals with the following exclusion criteria: no serious illness, no use of antibiotics until 6 months prior to the study, no pregnancy or lactation, healthy oral status and absence of carious lesions. The food isolates from raw milk samples from different cows were received in 2014 from the Bavarian Health and Food Safety Authority (Oberschleißheim, Germany) and the isolates from nosocomial infections were obtained from patients in 2013 from the Department of Medical Microbiology and Hygiene (Medical Center - University of Freiburg, Faculty of Medicine, University of Freiburg, Germany). All endodontic and clinical isolates were obtained after approval by the Ethics Committee (no. 140/09, University of Freiburg) and the collection of the endodontic samples followed the protocol from Schirrmeister et al. (2007). Prior to the antibiotic susceptibility testing, the isolates were confirmed to be E. faecalis by amplification of a species-specific 16S rDNA fragment (Table 1). The following strains were used as reference strains: Klebsiella pneumoniae 1230 and Enterobacter cloacae 472 (containing the bla TEM gene), Enterococcus faecium 401, E. faecium 403 and E. faecium 643 (containing the ermB-gene), Staphylococcus aureus 2250, S. aureus 2223, S. aureus 4331 (containing the ermC-gene), E. faecium 633 and E. faecium 643 (containing the vanA-gene), E. faecalis V583, E. faecium BM4524, E. faecium 401 (containing the vanB-gene), E. faecium 633 (containing the tetM-gene) and a bacterial isolate from sewage MG (containing the tetO-gene). All reference strains were kindly provided by Prof. Daniel Jonas (Institute for Environmental Health Sciences and Hospital Infection Control, Medical Center - University of Freiburg, Faculty of Medicine, University of Freiburg, Germany).
2.2 Antibiotic Susceptibility Testing with the Etest
The Etest (Liofilchem, Roseto degli Abruzzi, Italy) was used to test the susceptibility of all the E. faecalis isolates to the following antibiotics: Doxycycline, azithromycin, amoxicillin, rifampicin and high-level streptomycin. The method was conducted according to the manufacturer’s protocol as described earlier (Al-Ahmad et al. 2014). Specifically, material from pure colonies was taken from an over-night culture, suspended in sterile NaCl-solution (0.9%) to reach an inoculum turbidity of McFarland 0.5. Each sample was streaked on Mueller-Hinton agar plates and one Etest strip was placed on each agar plate using sterile tweezers. The results were interpreted as indicating susceptible, intermediate or resistant categories according to the EUCAST (The European Committee on Antimicrobial Susceptibility Testing) breakpoints and, where EUCAST values were not available, to the CSLI (Clinical and Laboratory Standards Institute), both listed in Table 2 (EUCAST 2016; CLSI 2013). If these standards were not available, minimal inhibitory concentration (MIC) values were compared with values for similar strains in literature.
3 DNA Isolation
Material from pure cultures was used to extract total bacterial DNA with the DNeasy Blood and Tissue Kit (Qiagen, Hilden, Germany). The DNA extraction was performed according to the manufacturer’s protocol for Gram-positive bacteria. The DNA was eluted with 200 μl AE buffer (Qiagen) and stored at −20 °C.
3.1 PCR for the Detection of E. faecalis Antibiotic Resistance Genes
The isolated DNA was used as a template for the amplification of nine different antibiotic resistance genes from E. faecalis. The primers, annealing temperatures and corresponding references are listed in Table 1. To amplify the different fragments, initial denaturation was performed at 94 °C for 5 min, followed by 35 cycles with denaturation at 94 °C for 60 s, varying annealing times (Table S1), extension at 72 °C for 60s and a final extension at 72 °C for 10 min. The primer concentration, template amount and annealing temperature varied for the different PCR systems, therefore all respective information is listed in the supplementary material (Table S1). The amplification was performed in a total volume of 25 μl and all reaction mixtures contained 1x PCR buffer (Qiagen), 0.2 mM each of the four deoxyribonucleoside triphosphates (dNTPs; PEQLAB, Erlangen, Germany) and 2.5 U Taq-Polymerase (Qiagen) and the specific amount of forward and reverse primers as well as template DNA. A no-template control and a positive control were included in each set of PCR reactions. The amplified products were visualized by gel electrophoresis using a 1% agarose gel.
4 Statistical Analysis
The correlation of the antibiotic resistance genes and the phenotypic resistance characteristics with the respective origin of the E. faecalis isolates was analyzed using the Fisher’s exact test and pairwise comparisons were performed with the chi-square test with Bonferroni correction. The level of significance was 0.05.
5 Results
A total of 70 E. faecalis isolates from four different origins (endodontic, plaque/saliva, food and nosocomial isolates) were analyzed for the presence of nine antibiotic resistance genes and for their antibiotic susceptibility to five different antibiotics.
5.1 Phenotypic Antimicrobial Susceptibility of E. faecalis Isolates
Table S2 (Supplementary material) shows the MIC values for the tested antibiotic agents for all isolates in detail and additionally includes the resistance phenotype analyzed by VITEK in a prior study (Anderson et al. 2015). All tested isolates were susceptible to amoxicillin. As shown in Fig. 1, a high percentage of the plaque/saliva isolates from healthy individuals (81.0%), of the food (78.6%) and the nosocomial isolates (73.33%) were resistant against doxycycline, as well as against azithromycin (81.0%; 85.6% and 86.7% resp.). Rifampicin resistance was detected in about half the plaque/saliva and endodontic isolates (52.4% and 50%), in 40% of the nosocomial isolates and in a lower percentage of the food isolates (14.3%). While none of the oral isolates showed resistance against high-level streptomycin, 46.7% of the nosocomial and 35.7% of the food isolates showed resistance.
5.2 Multidrug-Resistance among E. faecalis Isolates from Different Origins
According to the classification recommendations suggested by the European Center for Disease Prevention and Control (ECDC) only Enterococci resistant to ≥1 agent in ≥3 antimicrobial categories relevant for these species (Magiorakos et al. 2012) can be determined as multidrug-resistant (MDR). In our study this would correspond to a combined resistance against high-level gentamicin, high-level streptomycin, ciprofloxacin/levofloxacin, vancomycin, linezolid and doxycycline. According to these guidelines, several isolates of the nosocomial infections (sample nr. 110053, 109891, 512359, resistant to doxycycline, high-level streptomycin, high-level gentamicin and levofloxacin/ciprofloxacin as well as 109898 and 512176, resistant to high-level streptomycin, high-level gentamicin and levofloxacin/ciprofloxacin; this study and (Anderson et al. 2015)), can be classified as multidrug-resistant. Still, many of the other tested isolates showed resistance to more than one antibiotic. Several other nosocomial isolates showed resistance to doxycycline and high-level gentamicin or high-level gentamicin and levofloxacin/ciprofloxacin. Nine plaque/saliva isolates showed combined resistance against doxycycline and high-level gentamicin and one endodontic isolate showed resistance to doxycycline and linezolid.
5.3 Distribution of Antibiotic Resistance Genes in E. faecalis Isolates
Figure 2 shows the percentage of the detected resistance genes in the E. faecalis isolates. The tetM gene was present in isolates from all four origins in high percentages, i.e. 65.0%, 80.0%, 86.7% and 90.5% of the endodontic, nosocomial, food and plaque/saliva isolates respectively, while other tetracycline resistance genes (tetO) were not detected. The ermB gene was detected in many nosocomial isolates (60%) and in 47.6% and 26.6% of the plaque/saliva and food isolates respectively. BlaTEM genes were found primarily in nosocomial infection isolates (53.3%), but also 30.0% of the endodontic isolates and 13.3% of the food isolates harbored these genes. ErmC genes and genes for the resistance to vancomycin were not present in any of the isolates.
5.4 Statistical Results – Significant Associations of Detected Resistance and the Origin of the E. faecalis Isolates
The analysis of possible correlations between the antibiotic resistance results and the origin of the isolates revealed that the presence of the ermB gene correlated with the origin (p ≤ 0.001), 60% of the nosocomial isolates and 47% of the plaque/saliva isolates were found positive for this gene, whereas none of the endodontic and only 26.5% of the food isolates were positive for it. The bla TEM gene also correlated with the origin (p ≤ 0.001), 53.3% of the nosocomial isolates possessed this trait and 30% of the endodontic isolates, yet only 13.3% of the food and none of the plaque/saliva isolates. Phenotypic doxycycline resistance correlated with the origin (p ≤ 0.01), it was widespread in food, nosocomial and plaque/saliva isolates (78.6%, 73.3%, 81.0%), yet only few endodontic isolates (35%) showed resistance. Similarly, high-level streptomycin resistance correlated with the origin of the isolates (p ≤ 0.001), food and nosocomial isolates were frequently resistant (35.7% and 46.7% respectively), whereas all oral isolates were susceptible.
The pairwise comparison of selected traits showed significantly more doxycycline-resistant isolates from plaque/saliva and from food than from endodontic infections (p ≤ 0.01 and p = 0.036 resp.). Also, significantly more food isolates and nosocomial isolates were resistant to high-level streptomycin than endodontic and plaque/saliva isolates (p = 0.006 / p = 0.006 and p ≤ 0.001 / p ≤ 0.001 resp.) In addition, significantly more isolates from nosocomial infections harbored the bla TEM gene than isolates from endodontic infections (p = 0.04).
6 Discussion
Especially since the 1990s, Enterococcus faecalis has emerged as a leading nosocomial pathogen and has been shown to have the ability to acquire and spread resistance genes readily (Arias and Murray 2012). However, the role of the oral cavity as a potential reservoir for resistant E. faecalis has not been clarified yet. Therefore this study reports the antibiotic susceptibility and antibiotic resistance genes of isolates from supragingival plaque and saliva of healthy individuals and of endodontic infections in comparison to isolates from nosocomial infections and food. Notably the plaque/saliva isolates stand out regarding their equipment with tetracycline resistance genes (tetM) and erythromycin resistance genes (ermB) which are comparable to the nosocomial isolates (90.5% versus 80% and 47.6% versus 60.0% respectively). Phenotypic azithromycin resistance of the plaque/saliva isolates is similar to the nosocomial isolates and phenotypic resistance to doxycycline and rifampicin is shown in the same range in the plaque/saliva isolates as in the nosocomial isolates (81.0% versus 73.3% and 52.4% versus 40% respectively) which indicates a possible role of the oral cavity as a reservoir for these resistance traits. The doxycycline resistance in plaque/saliva was significantly higher than in the food and the endodontic isolates. Both, doxycycline and azithromycin as well as tetracycline are used for the treatment of periodontitis and other dental diseases (Poveda Roda et al. 2007; Preshaw et al. 2004; Roberts and Mullany 2010). Erythromycin, next to clindamycin, can be prescribed for patients allergic to penicillin e.g. in endodontic infections (Jacinto et al. 2003). Rifampicin is commonly used in the treatment of serious infections as well as for chemoprophylaxis in bacterial meningitis (Gaetti-Jardim et al. 2010).
Up to now, only the results of one other study analyzing plaque isolates from healthy individuals are available (Poeta et al. 2009), but the authors only detected 3 E. faecalis isolates among other enterococci, which were all resistant to streptomycin, erythromycin and tetracycline, possessing ermB and tetM/tetL genes. In contrast to our results, Gaetti-Jardim et al., who analyzed mixed samples of saliva and plaque from healthy individuals and those with different dental diseases found much lower resistance to doxycycline (12.9) and no resistance to rifampicin, yet 19.4% resistance to amoxicillin compared to 0% in our isolates (Gaetti-Jardim et al. 2010). This could be explained by geographical differences considering antibiotic use, since these isolates stemmed from Brazilian patients. Another very recent Brazilian study analyzed a large number of E. faecalis isolates from oral rinses of healthy individuals reporting a similar amoxicillin resistance (12.3%) corresponding with Gaetti-Jardims results (Komiyama et al. 2016). Fifty-three percent of the tested isolates in their study were resistant to tetracycline compared to over 90% of plaque isolates in the present study harboring the tetM gene, and 85.7% being phenotypically resistant in an earlier study of our group (Anderson et al. 2015).
As far as studying isolates from healthy individuals, most other authors have analyzed fecal isolates, e.g. Kuch et al. (Kuch et al. 2012), finding resistance to rifampicin (37%), to tetracycline (55.6%) and to high-level gentamicin (8.6%) mostly in the same range as our results, although slightly less than we found in plaque/saliva from healthy individuals (52.4%, 85.7% and 47.5% respectively). Lietzau et al. analyzed feces samples from healthy individuals in Germany and found only 29.8% of the E. faecalis isolates resistant to doxycycline compared to 81% of our plaque/saliva isolates (Lietzau et al. 2006). Another study that investigated a large number of nosocomial and a few commensal strains from various geographical regions detected tetracycline resistance (conferred through tetM and tetL), erythromycin resistance (conferred through ermB) and high level gentamicin as well as vancomycin resistance in the nosocomial isolates (McBride et al. 2007). Yet the commensal strains only harbored the tetM gene in contrast to our plaque/ saliva isolates of which nearly 50% possessed the ermB gene.
Endodontic isolates have been extensively analyzed for their virulence and antibiotic resistance traits, since E. faecalis is thought to contribute to persistent root canal infections (Anderson et al. 2013). Increasing resistances, e.g. against tetracycline, rifampicin, ciprofloxacin and erythromycin have been reported (Al-Ahmad et al. 2014). Pinheiro et al. detected 85.8% azithromycin 71.5% erythromycin and 14.3% tetracycline as well as doxycycline resistance in endodontic E. faecalis (Pinheiro et al. 2004). In comparison, the present study detected 55% azithromycin, 35% doxycycline and 65% tetracycline resistant isolates. A recent study by Barbosa-Ribeiro et al. detected isolates showing intermediate and full resistance to amoxicillin, azithromycin, rifampicin and doxycycline from endodontic retreatment cases, concurring with our results, although with lower percentages for the latter three antibiotics (Barbosa-Ribeiro et al. 2016). Regarding their equipment with resistance genes, our endodontic samples showed a fairly high percentage of isolates carrying the blaTEM gene (30%) compared with our nosocomial isolates (53.3%). Although enterococci possess intrinsic resistance against beta-lactam antibiotics, this resistance varies and e.g. ampicillin still has a high effectiveness against E. faecalis (Kristich et al. 2014). Other authors (Rocas and Siqueira 2013; Jungermann et al. 2011) have found bla TEM in DNA extracts from endodontic infections. The equipment with resistance genes as well as phenotypic resistance against other tested antibiotics was lower for the endodontic isolates in the present study than for isolates of other sources.
For the last two decades most studies of nosocomial E. faecalis isolates regarding their antibiotic resistance have focused specifically on vancomycin resistance, while data on other antibiotics is less frequent (Ruiz-Garbajosa et al. 2006). In our study, we did not detect any vancomycin resistance in the nosocomial isolates, nor the isolates from other origins, which for isolates from the oral cavity is a consistent and favorable finding, considering the function of vancomycin as reserve antibiotic (Barbosa-Ribeiro et al. 2016; Komiyama et al. 2016; Pinheiro et al. 2004; Rams et al. 2013; C. M. Sedgley et al. 2005a; Dahlen et al. 2012).
On the other hand, high percentages of the nosocomial isolates of the present study exhibited doxycycline and azithromycin resistance as well as the possession of blaTEM, tetM and ermB. Azithromycin resistance has also been found in a high percentage of nosocomial isolates from German patients by Wenzler et al. (72% versus 86.6% percent in our study) (Wenzler et al. 2004) and frequent rifampicin resistance has been reported by Kuch et al. (36.7% versus 40% in our study) (Kuch et al. 2012). Kuch also reported high-level streptomycin resistance in 26.7% of the German nosocomial isolates (Kuch et al. 2012) which is somewhat higher in our study, with 46.7% of our nosocomial isolates showing resistance.
Regarding the food isolates tested in the present study, we found a high resistance to doxycycline and azithromycin, comparable to the plaque/ saliva and nosocomial isolates, as well as frequent possession of tetM, while they were susceptible to most other tested antibiotics. In contrast to the oral isolates those from food showed a measure of high-level streptomycin resistance (35.7%). E. faecalis is frequently detected not only in raw milk but also raw milk cheeses (Jamet et al. 2012) and isolates have been found to be resistant to various antibiotics, e.g. tetracycline, erythromycin, rifampicin and streptomycin in concordance with our results (Jamet et al. 2012; Schlegelova et al. 2002; Templer and Baumgartner 2007). Jamet et al. found tetM and ermB genes widespread in multiple resistant cheese isolates, and their multilocus sequence typing (MLST) analysis revealed several isolates from clonal complexes that have been associated with periodontitis (Jamet et al. 2012). This finding is significant against the backdrop of the study of Al-Ahmad et al. reporting that E. faecalis isolates from cheese were able to integrate into the oral biofilm in vivo (Al-Ahmad et al. 2010). Thus, also food isolates, e.g. raw milk and products thereof could serve as a resistance reservoir and facilitate the spread of resistance through transfer of genes to oral E. faecalis. The possibility of a transfer of resistance genes from these cheese isolates to the oral isolates or among different oral E. faecalis isolates through conjugative transposons in vivo has not been studied yet. Nevertheless, oral E. faecalis has been proven to be a recipient of resistance genes from other species (Roberts et al. 2001) in vitro and transfer of plasmid coded erythromycin resistance has been shown in root canals ex vivo (C. M. Sedgley et al. 2008). The presence of the Tn916-like element, carrying e.g. tetM, and other transposons has been detected in E. faecalis from food as well as oral enterococci (Jamet et al. 2012; Roberts and Mullany 2011; Kristich et al. 2014).
In summary we can conclude that both the oral cavity, in particular dental plaque and saliva, as well as foods can present a reservoir of E. faecalis strains with multiple antibiotic resistances including the potential of resistance transfer to other strains or even other species. Consequently, continued monitoring of E. faecalis for antibiotic resistance should be performed not only for nosocomial, but also for oral strains.
Funding Information
This study was partly supported by the German Research foundation (DFG, AL-1179/2–1) and by the Ministry of Science, Research and the Arts in Baden-Württemberg (VR-MED_FAK).
References
Al-Ahmad A, Maier J, Follo M, Spitzmuller B, Wittmer A, Hellwig E et al (2010) Food-borne enterococci integrate into oral biofilm: an in vivo study. J Endod 36(11):1812–1819. doi:10.1016/j.joen.2010.08.011
Al-Ahmad A, Ameen H, Pelz K, Karygianni L, Wittmer A, Anderson AC et al (2014) Antibiotic resistance and capacity for biofilm formation of different bacteria isolated from endodontic infections associated with root-filled teeth. J Endod 40(2):223–230. doi:10.1016/j.joen.2013.07.023
Amsler K, Santoro C, Foleno B, Bush K, Flamm R (2010) Comparison of broth microdilution, agar dilution, and Etest for susceptibility testing of doripenem against gram-negative and gram-positive pathogens. J Clin Microbiol 48(9):3353–3357. doi:10.1128/jcm.00494-10
Anderson AC, Al-Ahmad A, Elamin F, Jonas D, Mirghani Y, Schilhabel M et al (2013) Comparison of the bacterial composition and structure in symptomatic and asymptomatic endodontic infections associated with root-filled teeth using pyrosequencing. PLoS One 8(12):e84960. doi:10.1371/journal.pone.0084960
Anderson AC, Jonas D, Huber I, Karygianni L, Wolber J, Hellwig E et al (2015) Enterococcus faecalis from food, clinical specimens, and oral sites: prevalence of virulence factors in association with biofilm formation. Front Microbiol 6:1534. doi:10.3389/fmicb.2015.01534
Arias CA, Murray BE (2012) The rise of the Enterococcus: beyond vancomycin resistance. Nat Rev Microbiol 10(4):266–278. doi:10.1038/nrmicro2761
Barbosa-Ribeiro M, De-Jesus-Soares A, Zaia AA, Ferraz CC, Almeida JF, Gomes BP (2016) Antimicrobial susceptibility and characterization of virulence genes of Enterococcus faecalis isolates from teeth with failure of the endodontic treatment. J Endod. doi:10.1016/j.joen.2016.03.015
Call DR, Bakko MK, Krug MJ, Roberts MC (2003) Identifying antimicrobial resistance genes with DNA microarrays. Antimicrob Agents Chemother 47(10):3290–3295
CLSI (2013) CLSI. Performance Standards for Antimicrobial Susceptibility Testing; Twenty-Third Informational Supplement. CLSI document M100-S23. Clinical and Laboratory Standards Institute, Wayne, PA
Dahlen G, Samuelsson W, Molander A, Reit C (2000) Identification and antimicrobial susceptibility of enterococci isolated from the root canal. Oral Microbiol Immunol 15(5):309–312
Dahlen G, Blomqvist S, Almstahl A, Carlen A (2012) Virulence factors and antibiotic susceptibility in enterococci isolated from oral mucosal and deep infections. J Oral Microbiol:4. doi:10.3402/jom.v4i0.10855
Depardieu F, Kolbert M, Pruul H, Bell J, Courvalin P (2004) VanD-type vancomycin-resistant Enterococcus faecium and Enterococcus faecalis. Antimicrob Agents Chemother 48(10):3892–3904. doi:10.1128/aac.48.10.3892-3904.2004
Duggan JM, Sedgley CM (2007) Biofilm formation of oral and endodontic Enterococcus faecalis. J Endod 33(7):815–818. doi:10.1016/j.joen.2007.02.016
Dutka-Malen S, Evers S, Courvalin P (1995) Detection of glycopeptide resistance genotypes and identification to the species level of clinically relevant enterococci by PCR. J Clin Microbiol 33(1):24–27
EUCAST (2016) The European Committee on Antimicrobial Susceptibility Testing. Breakpoint tables for interpretation of MICs and zone diameters. Version 6.0, 2016 http://www.eucast.org. http://www.eucast.org/clinical_breakpoints/
Fass RJ (1993) Erythromycin, clarithromycin, and azithromycin: use of frequency distribution curves, scattergrams, and regression analyses to compare in vitro activities and describe cross-resistance. Antimicrob Agents Chemother 37(10):2080–2086
Fisher K, Phillips C (2009) The ecology, epidemiology and virulence of Enterococcus. Microbiology 155(Pt 6):1749–1757. doi:10.1099/mic.0.026385-0
Franz CM, Huch M, Abriouel H, Holzapfel W, Galvez A (2011) Enterococci as probiotics and their implications in food safety. Int J Food Microbiol 151(2):125–140. doi:10.1016/j.ijfoodmicro.2011.08.014
Gaetti-Jardim EC, Marqueti AC, Faverani LP, Gaetti-Jardim E Jr (2010) Antimicrobial resistance of aerobes and facultative anaerobes isolated from the oral cavity. J Appl Oral Sci 18(6):551–559
Hammerum AM (2012) Enterococci of animal origin and their significance for public health. Clin Microbiol Infect 18(7):619–625. doi:10.1111/j.1469-0691.2012.03829.x
Jacinto RC, Gomes BP, Ferraz CC, Zaia AA, Filho FJ (2003) Microbiological analysis of infected root canals from symptomatic and asymptomatic teeth with periapical periodontitis and the antimicrobial susceptibility of some isolated anaerobic bacteria. Oral Microbiol Immunol 18(5):285–292
Jamet E, Akary E, Poisson MA, Chamba JF, Bertrand X, Serror P (2012) Prevalence and characterization of antibiotic resistant Enterococcus faecalis in French cheeses. Food Microbiol 31(2):191–198. doi:10.1016/j.fm.2012.03.009
Jorgensen JH, Ferraro MJ (2009) Antimicrobial susceptibility testing: a review of general principles and contemporary practices. Clin Infect Dis 49(11):1749–1755. doi:10.1086/647952
Jungermann GB, Burns K, Nandakumar R, Tolba M, Venezia RA, Fouad AF (2011) Antibiotic resistance in primary and persistent endodontic infections. J Endod 37(10):1337–1344. doi:10.1016/j.joen.2011.06.028
Komiyama EY, Lepesqueur LS, Yassuda CG, Samaranayake LP, Parahitiyawa NB, Balducci I et al (2016) Enterococcus species in the oral cavity: prevalence, virulence factors and antimicrobial susceptibility. PLoS One 11(9):e0163001. doi:10.1371/journal.pone.0163001
Kouidhi B, Zmantar T, Mahdouani K, Hentati H, Bakhrouf A (2011) Antibiotic resistance and adhesion properties of oral Enterococci associated to dental caries. BMC Microbiol 11:155. doi:10.1186/1471-2180-11-155
Kristich CJ, Rice LB, Arias CA (2014) Enterococcal infection-treatment and antibiotic resistance. In: Gilmore MS, Clewell DB, Ike Y, Shankar N (eds) Enterococci: from commensals to leading causes of drug resistant infection. Massachusetts Eye and Ear Infirmary, Boston
Kuch A, Willems RJ, Werner G, Coque TM, Hammerum AM, Sundsfjord A et al (2012) Insight into antimicrobial susceptibility and population structure of contemporary human Enterococcus faecalis isolates from Europe. J Antimicrob Chemother 67(3):551–558. doi:10.1093/jac/dkr544
Lietzau S, Hoewner M, von Baum H, Marre R, Brenner H (2006) Antibiotic resistant fecal isolates of Enterococci among unselected patients outside the clinical sector: an epidemiological study from Southern Germany. Pharmacoepidemiol Drug Saf 15(4):275–277. doi:10.1002/pds.1167
Magiorakos AP, Srinivasan A, Carey RB, Carmeli Y, Falagas ME, Giske CG et al (2012) Multidrug-resistant, extensively drug-resistant and pandrug-resistant bacteria: an international expert proposal for interim standard definitions for acquired resistance. Clin Microbiol Infect 18(3):268–281. doi:10.1111/j.1469-0691.2011.03570.x
Manson JM, Hancock LE, Gilmore MS (2010) Mechanism of chromosomal transfer of Enterococcus faecalis pathogenicity island, capsule, antimicrobial resistance, and other traits. Proc Natl Acad Sci U S A 107(27):12269–12274. doi:10.1073/pnas.1000139107
McBride SM, Fischetti VA, Leblanc DJ, Moellering RC Jr, Gilmore MS (2007) Genetic diversity among Enterococcus faecalis. PLoS One 2(7):e582. doi:10.1371/journal.pone.0000582
Murray BE (1990) The life and times of the Enterococcus. Clin Microbiol Rev 3(1):46–65
Paganelli FL, Willems RJ, Leavis HL (2012) Optimizing future treatment of enterococcal infections: attacking the biofilm? Trends Microbiol 20(1):40–49. doi:10.1016/j.tim.2011.11.001
Palmer KL, Kos VN, Gilmore MS (2010) Horizontal gene transfer and the genomics of enterococcal antibiotic resistance. Curr Opin Microbiol 13(5):632–639. doi:10.1016/j.mib.2010.08.004
Paulsen IT, Banerjei L, Myers GS, Nelson KE, Seshadri R, Read TD et al (2003) Role of mobile DNA in the evolution of vancomycin-resistant Enterococcus faecalis. Science 299(5615):2071–2074. doi:10.1126/science.1080613
Perez-Trallero E, Montes M, Orden B, Tamayo E, Garcia-Arenzana JM, Marimon JM (2007) Phenotypic and genotypic characterization of Streptococcus pyogenes isolates displaying the MLSB phenotype of macrolide resistance in Spain, 1999 to 2005. Antimicrob Agents Chemother 51(4):1228–1233. doi:10.1128/aac.01054-06
Perreten V, Vorlet-Fawer L, Slickers P, Ehricht R, Kuhnert P, Frey J (2005) Microarray-based detection of 90 antibiotic resistance genes of gram-positive bacteria. J Clin Microbiol 43(5):2291–2302. doi:10.1128/jcm.43.5.2291-2302.2005
Pinheiro ET, Gomes BP, Drucker DB, Zaia AA, Ferraz CC, Souza-Filho FJ (2004) Antimicrobial susceptibility of Enterococcus faecalis isolated from canals of root filled teeth with periapical lesions. Int Endod J 37(11):756–763. doi:10.1111/j.1365-2591.2004.00865.x
Poeta P, Igrejas G, Goncalves A, Martins E, Araujo C, Carvalho C et al (2009) Influence of oral hygiene in patients with fixed appliances in the oral carriage of antimicrobial-resistant Escherichia coli and Enterococcus isolates. Oral Surg Oral Med Oral Pathol Oral Radiol Endod 108(4):557–564. doi:10.1016/j.tripleo.2009.06.002
Portenier I, Waltimo TMT, Haapasalo M (2003) Enterococcus faecalis– the root canal survivor and ‘star’ in post-treatment disease. Endod Top 6(1):135–159. doi:10.1111/j.1601-1546.2003.00040.x
Poveda Roda R, Bagan JV, Sanchis Bielsa JM, Carbonell Pastor E (2007) Antibiotic use in dental practice: a review. Med Oral Patol Oral Cir Bucal 12(3):E186–E192
Preshaw PM, Hefti AF, Jepsen S, Etienne D, Walker C, Bradshaw MH (2004) Subantimicrobial dose doxycycline as adjunctive treatment for periodontitis: a review. J Clin Periodontol 31(9):697–707. doi:10.1111/j.1600-051X.2004.00558.x
Rams TE, Feik D, Mortensen JE, Degener JE, van Winkelhoff AJ (2013) Antibiotic susceptibility of periodontal Enterococcus faecalis. J Periodontol 84(7):1026–1033. doi:10.1902/jop.2012.120050
Reinert RR, Filimonova OY, Al-Lahham A, Grudinina SA, Ilina EN, Weigel LM et al (2008) Mechanisms of macrolide resistance among Streptococcus pneumoniae isolates from Russia. Antimicrob Agents Chemother 52(6):2260–2262. doi:10.1128/aac.01270-07
Roberts AP, Mullany P (2010) Oral biofilms: a reservoir of transferable, bacterial, antimicrobial resistance. Expert Rev Anti-Infect Ther 8(12):1441–1450. doi:10.1586/eri.10.106
Roberts AP, Mullany P (2011) Tn916-like genetic elements: a diverse group of modular mobile elements conferring antibiotic resistance. FEMS Microbiol Rev 35(5):856–871. doi:10.1111/j.1574-6976.2011.00283.x
Roberts AP, Cheah G, Ready D, Pratten J, Wilson M, Mullany P (2001) Transfer of TN916-like elements in microcosm dental plaques. Antimicrob Agents Chemother 45(10):2943–2946. doi:10.1128/aac.45.10.2943-2946.2001
Rocas IN, Siqueira JF Jr (2013) Detection of antibiotic resistance genes in samples from acute and chronic endodontic infections and after treatment. Arch Oral Biol 58(9):1123–1128. doi:10.1016/j.archoralbio.2013.03.010
Ruiz-Garbajosa P, Canton R, Pintado V, Coque TM, Willems R, Baquero F et al (2006) Genetic and phenotypic differences among Enterococcus faecalis clones from intestinal colonisation and invasive disease. Clin Microbiol Infect 12(12):1193–1198. doi:10.1111/j.1469-0691.2006.01533.x
Schirrmeister JF, Liebenow AL, Braun G, Wittmer A, Hellwig E, Al-Ahmad A (2007) Detection and eradication of microorganisms in root-filled teeth associated with periradicular lesions: an in vivo study. J Endod 33(5):536–540. doi:10.1016/j.joen.2007.01.012
Schlegelova J, Babak V, Klimova E, Lukasova J, Navratilova P, Sustackova A et al (2002) Prevalence of and resistance to anti-microbial drugs in selected microbial species isolated from bulk milk samples. J Vet Med B Infect Dis Vet Public Health 49(5):216–225
Sedgley CM, Molander A, Flannagan SE, Nagel AC, Appelbe OK, Clewell DB et al (2005a) Virulence, phenotype and genotype characteristics of endodontic Enterococcus spp. Oral Microbiol Immunol 20(1):10–19. doi:10.1111/j.1399-302X.2004.00180.x
Sedgley CM, Nagel AC, Shelburne CE, Clewell DB, Appelbe O, Molander A (2005b) Quantitative real-time PCR detection of oral Enterococcus faecalis in humans. Arch Oral Biol 50(6):575–583. doi:10.1016/j.archoralbio.2004.10.017
Sedgley C, Buck G, Appelbe O (2006) Prevalence of Enterococcus faecalis at multiple oral sites in endodontic patients using culture and PCR. J Endod 32(2):104–109. doi:10.1016/j.joen.2005.10.022
Sedgley CM, Lee EH, Martin MJ, Flannagan SE (2008) Antibiotic resistance gene transfer between Streptococcus gordonii and Enterococcus faecalis in root canals of teeth ex vivo. J Endod 34(5):570–574. doi:10.1016/j.joen.2008.02.014
Siqueira JF Jr, Rocas IN (2004) Polymerase chain reaction-based analysis of microorganisms associated with failed endodontic treatment. Oral Surg Oral Med Oral Pathol Oral Radiol Endod 97(1):85–94. doi:10.1016/s1079210403003536
Souto R, Colombo AP (2008) Prevalence of Enterococcus faecalis in subgingival biofilm and saliva of subjects with chronic periodontal infection. Arch Oral Biol 53(2):155–160. doi:10.1016/j.archoralbio.2007.08.004
Sun J, Song X, Kristiansen BE, Kjaereng A, Willems RJ, Eriksen HM et al (2009) Occurrence, population structure, and antimicrobial resistance of enterococci in marginal and apical periodontitis. J Clin Microbiol 47(7):2218–2225. doi:10.1128/jcm.00388-09
Templer SP, Baumgartner A (2007) Enterococci from Appenzeller and Schabziger raw milk cheese: antibiotic resistance, virulence factors, and persistence of particular strains in the products. J Food Prot 70(2):450–455
Tenover FC, Baker CN, Swenson JM (1996) Evaluation of commercial methods for determining antimicrobial susceptibility of Streptococcus Pneumoniae. J Clin Microbiol 34(1):10–14
Thurnheer T, Belibasakis GN (2015) Integration of non-oral bacteria into in vitro oral biofilms. Virulence 6(3):258–264. doi:10.4161/21505594.2014.967608
Van Tyne D, Gilmore MS (2014) Friend turned foe: evolution of enterococcal virulence and antibiotic resistance. Annu Rev Microbiol 68:337–356. doi:10.1146/annurev-micro-091213-113003
Wenzler S, Schmidt-Eisenlohr E, Daschner F (2004) Comparative in vitro activities of three new quinolones and azithromycin against aerobic pathogens causing respiratory tract and abdominal wound infections. Chemotherapy 50(1):40–42. doi:10.1159/000077284
Acknowledgements
The authors thank Bettina Spitzmüller, Kristina Kollmar and Annette Wittmer for excellent technical assistance and Nicole Arweiler, Daniel Jonas and Ingrid Huber for providing part of the isolates, as well as Grant Anderson for English language correction.
Conflicts of Interest
The authors deny any conflicts of interest related to this study.
Ethical Statement
All endodontic and clinical isolates were obtained after approval by the Ethics Committee (no. 140/09, University of Freiburg).
Author information
Authors and Affiliations
Corresponding author
Editor information
Editors and Affiliations
Supplementary Material
Supplementary Material
Rights and permissions
Copyright information
© 2017 Springer International Publishing AG
About this chapter
Cite this chapter
Anderson, A.C., Andisha, H., Hellwig, E., Jonas, D., Vach, K., Al-Ahmad, A. (2017). Antibiotic Resistance Genes and Antibiotic Susceptibility of Oral Enterococcus faecalis Isolates Compared to Isolates from Hospitalized Patients and Food. In: Donelli, G. (eds) Advances in Microbiology, Infectious Diseases and Public Health. Advances in Experimental Medicine and Biology(), vol 1057. Springer, Cham. https://doi.org/10.1007/5584_2017_53
Download citation
DOI: https://doi.org/10.1007/5584_2017_53
Published:
Publisher Name: Springer, Cham
Print ISBN: 978-3-319-79016-9
Online ISBN: 978-3-319-79017-6
eBook Packages: Biomedical and Life SciencesBiomedical and Life Sciences (R0)