Abstract
The contamination of aquifers due to the leaching of organic and inorganic waste materials deposited in landfills and open dumpsites leads to serious degradation of the environment. To determine the extent of leaching and to assess the migration of the leachate into the surrounding aquifer, vertical electrical sounding (VES) and electrical resistivity tomography (ERT) surveys were carried out along a heavily contaminated waste disposal site in South-eastern Nigeria. The results obtained from the vertical electrical soundings show that the area comprises three subsurface layers, and that the aquifer lies between the second and third layer. Computation of longitudinal conductance values shows that the overall protective capacity of the aquifer is weak; hence, the capacity for leachate contamination within the aquifer is higher. Models obtained from the electrical resistivity tomography surveys indicate the direction of the leachate plumes and also show the extent of leachate percolation into the aquifer. On the electrical resistivity tomography models, the locations of anomalous low resistivity zones indicate the presence of leachate. The results show that the leachate is moving rapidly towards the aquifer and this will create serious environmental degradation to valuable water resources in the area, thereby affecting health of humans and livestock within this heavily populated zone. Further, the results from the resistivity surveys confirm that the resistivity technique is a powerful tool for delineating the presence of leachate plumes and for indicating to what extent the leachate has infiltrated into the surrounding aquifer.
Similar content being viewed by others
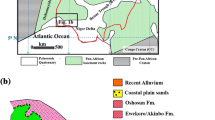
Explore related subjects
Discover the latest articles, news and stories from top researchers in related subjects.Avoid common mistakes on your manuscript.
Introduction
There has been a growing increase in the amount of waste disposed in landfills and waste disposal systems as a result of increased global population and higher level of industrial activities (Guy et al 2000; Olagunju et al. 2017). Waste products are typically disposed in landfills and waste-disposal systems where they pose significant negative influences on public health and cause degradation of the environment (Martinho and Almeida 2006). In consequence of these negative effects, landfills and dumpsites must be located far from residential areas (Dolk et al. 1998). Certain landfills have their waste enclosed within them so as to prevent infiltration of rainfall or snow, which leads to the production of leachate (Lohva et al. 2008; Genelle et al. 2012). Leachate is the liquid formed from the decomposed waste, and it typically has high electrical conductivity as result of the dissolved salts within it (Yoon et al. 2002; Martinho and Almeida 2006). Leachate plumes have higher electrical conductivity than the surrounding pore waters; hence, such plumes can be detected using geophysical surveying methods such as vertical electrical sounding (VES) and electrical resistivity tomography (ERT) (Kamura 2002; Moreira et al 2014; Udosen and George 2018). Vertical electrical sounding and electrical resistivity tomography are geophysical techniques that reconstruct subsurface resistivity from current injected into the ground (Gautam and Biswas 2016; George et al. 2017; Udosen and Potthast 2018).
Vertical electrical soundings (VES) and electrical resistivity tomography (ERT) are non-invasive and quick methods of delineating leachate plumes within the subsurface (Naudet et al. 2004; Gallas et al. 2011, Akinola et al. 2018). Resistivity methods can also be used to determine the depth between the waste material and the underlying ground cover (Chambers et al. 2006; Leroux et al. 2007) and to monitor the rate at which leachate has re-circulated (Grellier et al. 2008; Clement et al. 2011). These resistivity methods measure apparent electrical resistivity, and they enable a fast mapping of the resistivity distribution within an area (Devaraj et al. 2018). The electrical resistivity distribution within an area is influenced by soil porosity, salinity, temperature, cementation and mineral content of subsurface materials (White et al. 2015).
The South-eastern part of Nigeria has numerous non-enclosed waste-disposal systems and these pose numerous aesthetic and public health problems. The solid waste within these waste-disposal systems decompose slowly over several years, sending a large volume of leachate and other environmental pollutants into underground aquifers. These non-enclosed waste-disposal systems lead to contamination of the aquifer; hence this work uses electrical resistivity methods which have been found very useful in investigating leachate contamination at waste-disposal sites (Cardarelli and Bernabini 1997; Aristodemou and Thomas-Betts 2000; Bernstone et al. 2000; Ahmed and Sulaiman, 2001; Gawande et al. 2003; Abu-Zeid et al. 2004; Guerin et al. 2004; Batayneh and Barjous 2005; Depountis et al. 2005; Martinho and Almeida 2006; Zume et al. 2006; Olagunju et al. 2017; Fatoba et al. 2020).
The waste-disposal site under investigation is located in Uyo metropolis in Akwa Ibom State and was created in 2016 as the central waste-disposal site for Uyo, the capital of Akwa Ibom State. Uyo metropolis has a population of approximately 2 million. A previous waste-disposal site located a few miles away from this new site had generated lots of complaints from nearby residents. Complaints ranged from pollution of groundwater resources, environmental degradation, destruction of farm land, and air pollution. Consequently, in 2016, the government proposed a move to relocate the waste disposal site to a new location, where it felt the direct impact on public health and the environment would be minimal. This research investigates this new site, a few years after its creation, to evaluate its influence on public health and environmental degradation. This work uses vertical electrical sounding and electrical resistivity tomography to evaluate leachate contamination on the site. It aims at determining the extent and depth of leachate intrusion within the subsurface and alerting policy makers on the public health impacts and environmental degradation as a result of leachate percolation within the study area. As earlier mentioned, resistivity surveying methods are extremely useful tools for monitoring the extent of leachate percolation through the landmass.
Setting of study area
The study area, located in South-eastern Nigeria, lies between Latitudes 5.03° N and 5.05° N and Longitudes 7.93° E and 7.95° E. The geologic map of the study area is shown in Fig. 1 and the location map is shown in Fig. 2. The area is drained by Imo River, Kwa Iboe River and Cross River together with their tributaries. The elevation of the area ranges between 42 and 57 m above sea level. The study area comprises sandstone, gravels with intercalations of clay, and sandy clay in certain locations. The waste materials are dumped in the valley beside the site. The area experiences two seasons: wet season (April–October) and dry season (November–March), while the average annual rainfall is about 3000 mm characterized by humid tropical climate. Geologically, the study area is underlain by Recent to Tertiary sediments belonging to the Benin Formation (Tertiary to Quaternary coastal plain sands) of Niger Delta. The Benin Formation, underlain by the paralic Agbada Formation, has sediments which comprise fluvial loose sands, clays and lignite streaks. The Benin Formation is the major aquiferous unit in the area and it comprises of fine, medium and coarse sands alternating with lignite and thin clay at some areas.
Methods
Leachate percolation was modeled with vertical electrical soundings (VES) and electrical resistivity tomography (ERT) to determine the penetration depth of resistivity signals in the conductive waste and to investigate the extent of leachate accumulations within the waste-disposal site.
The VES and ERT data were collected using an ABEM resistivity meter, the aim being to obtain the depth to target, the thickness of each subsurface layer and the respective subsurface resistivity. Since dumpsites are usually heterogeneous, both VES and ERT profiles had electrodes lined up adjacent and across the site to enable the full mapping of leachate percolation within the site.
The VES data were acquired with the Schlumberger array configuration due to Schlumberger array’s high sensitivity to vertical structures and greater depth of subsurface penetration. The ERT data were acquired with the Wenner array configuration due to Wenner array’s high sensitivity to horizontal structures, low sensitivity to geological noise, and strong signal strength, which is useful in areas with high background noise.
Five VES profiles were acquired in the study area. All VES profiles had lengths of 400 m each. Four electrodes were used to generate data for each profile: two current electrodes were used to inject current into the subsurface and two potential electrodes were used to measure the corresponding potentials. The minimum electrode spacing was 1 m and the maximum electrode spacing was 200 m. At an initial potential electrode spacing of 0.5 m, the current electrode spacing used was 2 m, then 3 m, then 4 m, and finally 6 m. At a potential electrode spacing of 1 m, the current electrode spacing used was 8 m, then 10 m, then 12 m, then 14 m, then 16 m, then 18 m and finally 20 m. At a potential electrode spacing of 2 m, the current electrode spacing used was 10 m, then 30 m, then 40 m, and finally 60 m. At a potential electrode spacing of 5 m, the current electrode spacing used was 80 m, then 100 m, then 120 m, and finally 160 m. At a potential electrode spacing of 20 m, the current electrode spacing used was 200 m, then 300 m, and finally 400 m. The reason for the progressive increment in the potential and current electrode spacing was to increase the depth of current penetration. The VES field data were then input into WINRESIST software to generate logarithmic models of apparent resistivity against half the spacing between the current electrodes (AB/2). These models are shown in Figs. 3, 4, 5, 6, 7.
Two ERT profiles were acquired in the study area. All profiles had lengths of 100 m each. Four electrodes were used: two current electrodes to inject current into the subsurface and two potential electrodes to measure the corresponding potential. The minimum electrode spacing was 5 m and the maximum electrode spacing was 35 m. Data acquired from the field survey were modeled with RES2DINV software from Geotomo to generate 2D models of the resistivity distribution within the subsurface. These models are shown in Figs. 8 and 9.
Results and discussion
Five VES profiles were acquired in the study area and with the use of WINRESIST software. One-dimensional models of resistivity were obtained for the VES profiles. The VES models generated from the WINRESIST software are indicated in Figs. 3, 4, 5, 6 and 7. Each VES model indicates the resistivity and thickness of each subsurface layer (see Table 1 for the summary of this data). The VES models indicate the presence of three geo-electric layers (overlying motley topsoil, coarse sand and fine sand) with the aquifer lying between the second and third layer.
Two ERT profiles were acquired in the study area and with the use of RES2DINV software, two-dimensional models of resistivity were obtained for the ERT profiles. The ERT models generated from the RES2DINV software are indicated in Figs. 8 and 9. Each ERT model indicates the 2D image of the resistivity and thickness of the subsurface layers. Assessments of the ERT models show that the first subsurface layer (motley topsoil) has resistivity values ranging between 2.4 and 480 Ωm. The second layer (coarse sand) has resistivity values ranging between 120 and 3375 Ωm, and the third layer (fine sand) has resistivity values ranging between 19 and 4393 Ωm. The regions with anomalous low resistivity values are the leachate plumes (Yoon et al. 2002; Martinho and Almeida 2006). As earlier mentioned, leachate plumes have a higher electrical conductivity than the surrounding pore water due to the presence of dissolved salts within it. Hence, areas with anomalous values of high conductivity indicate the presence of leachate, and on the ERT models in Figs. 8 and 9, leachate is identified by high conductivity values (i.e., low resistivity values ranging from 0.5 to 6 Ωm) at about a depth range of 9 m. The ERT models show that the leachate is moving rapidly towards the aquifer. This is a worrisome situation as the sole potable water source in the area is ground water. The extent of leachate contamination is aggravated by the difference in topography between the waste disposal site and the groundwater body as the disposal site is located on a hill and hence, leachate will more easily drain into the groundwater system downstream. The leachate on this site results from the disposal of organic and inorganic matter on the site, which is characteristic of domestic and industrial waste deposited at this waste disposal site. The leachate plumes are indicated by anomalous zones of high conductivity. This has been confirmed by past work (e.g., Grellier 2004, 2007). In these past studies, temporal changes in resistivity were observed after injection of leachate into the subsurface. Zones with increased conductivity appeared at locations at which leachate was injected.
Given the known thicknesses and resistivity values of the study area’s lithology as obtained from the VES models generated in Figs. 3, 4, 5, 6, 7, it became possible to calculate the Dar-Zarrouk parameters, which are the longitudinal conductance S and the transverse resistance R. The longitudinal conductance \(S = \sum\limits_{n}^{i = 1} {\frac{{h_{i} }}{{\rho_{i} }}}\), where h is the thickness of the layers overlying the aquifer and ρa is the apparent resistivity of the layers overlying the aquifer layer. The longitudinal conductance defines areas with ground water potential. The transverse resistance \(T = \sum\limits_{n}^{i = 1} {h_{i} } \cdot \rho_{i}\) is also another parameter for defining areas with ground water potential.
Table 2 shows the results obtained from computation of the Dar-Zarrouk parameters and how these results have been used to indicate the protective capacity of the aquifer. Longitudinal conductance values ranging from 0.1 to 0.19 indicate weak protective capacity, values ranging from 0.2 to 0.69 indicate moderate protective capacity, and values ranging between 0.7 and 4.9 indicate good protective capacity while values less than 0.1 correspond to poor protective capacity (Oladapo and Akintorinwa 2007). Based on the longitudinal conductance values obtained from this site, the protective capacity of the aquifer ranges from good to poor.
The electrical resistivity reflection coefficient \(k = \frac{{\rho_{2} - \rho_{1} }}{{\rho_{2} + \rho_{1} }}\), which indicates how soil resistivity varies between the geoelectric layers was also computed (Table 2). This computation showed how resistivity varied between the first and second layers. The positive values of electrical resistivity reflection coefficient k obtained indicate that the resistivity values of the first layer are less than the resistivity values of the second layer. Negative values would have indicated that the resistivity of the first layer was greater than that of the second layer.
This work investigated leachate contamination resulting from deposition of organic and inorganic waste at waste-disposal site in South-eastern Nigeria. Waste disposal sites cause serious health hazards and environmental degradation, especially when such sites are unconfined. The interest in this work has been the aquifer, which is the sole water resource for people living around the area. The essence of the VES surveys (1D resistivity imaging) was to delineate and model the location of the aquifer and map out the litho-stratigraphic units within the area while the essence of the ERT surveys was to model the location and direction of the leachate plumes. Both methods, as combined as in this study, have successfully shown the extent and direction of leachate contamination on the site. Computations of the Dar-Zarrouk parameters have shown that the protective capacity of the aquifer is generally low, indicating that the study area has a very high rate of aquifer contamination.
Conclusions
This work set out to investigate migration of leachate within a contaminated waste-disposal site in South-east Nigeria with the use of vertical electrical sounding (VES) and electrical resistivity tomography (ERT). VES data were used to generate 1D models of the subsurface, and it indicated the presence of three subsurface layers, (motley sand, coarse sand and fine sand). The VES models show that the aquifer lies between the second and third layers. The ERT data was used to generate 2D models of the subsurface, and it indicated that leachate is very rapidly moving into the surrounding aquifer which lies between the second and third subsurface layer. This is alarming, being that the sole potable water source for residents in the region is groundwater. The ERT models indicate the presence of leachate plumes and its flow direction. It also shows that the leachate is migrating downward and will soon reach the water table to mix with the groundwater system. Given the generally poor protective capacity of the aquifer as computed from the Dar-Zarrouk parameters, this situation warrants urgent attention.
This work also shows that that integrated use of 1D and 2D surveys provided useful information about the electrical characteristics of the subsurface. Both VES and ERT surveys were able to describe both the depth and lateral boundary of the contaminant plumes, thereby providing useful information for the management and monitoring of the extent of contamination on the site. This confirms previous work (e.g., Yoon et al. 2002; Frid et al. 2008; Rosqvist et al. 2011; Olagunju et al. 2017; Fatoba et al. 2020). The resistivity surveys at our waste disposal site were conducted on a grid to determine the locations and boundaries of leachate contaminant plumes that arise from the presence of accumulated waste and to assess the characteristics and thickness of the underlying subsurface formation. Resistivity modeling is therefore, useful for mapping the subsurface resistivity structure as well as lateral changes in stratigraphy. ID and 2D surveys when used together, as it is done in this work, provide complementary information on how the subsurface resistivity is distributed. They also reveal the distribution of contaminant plumes and delineate the geo-stratigraphy of the study area.
References
Abu-Zeid N, Bianchini G, Santarato G, Vaccaro C (2004) Geochemical characterization and geophysical mapping of landfill leachates: the Marozzo canal case study (NE Italy). Environ Geol 45:439–447
Ahmed AM, Sulaiman WN (2001) Evaluation of groundwater and soil pollution in a landfill area using electrical resistivity imaging survey. Environ Manag 28:655–663
Akinola BS, Awoyemi MO, Matthew OJ, Adebayo AS (2018) Geophysical and hydro-chemical investigation of contamination plume in a basement complex formation around Sunmoye dumpsite in Ikire, Southwestern Nigeria. Model Earth Syst Environ 4:753–764
Aristodemou E, Thomas-Betts A (2000) DC resistivity and induced polarization investigations at a waste disposal site and its environments. J Appl Geophys 44:275–302
Batayneh AT, Barjous MO (2005) Resistivity surveys near a waste disposal site in the Qasr Tuba area of central Jordan. Bull Eng Geol Environ 64:285–291
Bernstone C, Dahlin T, Ohlsson T, Hogland H (2000) DC-resistivity mapping of internal landfill structures: two pre-excavation surveys. Environ Geol 39:360–371
Cardarelli E, Bernabini M (1997) Two case studies of the determination of parameters of urban waste dumps. J Appl Geophys 36:167–174
Chambers JE, Kuras O, Meldrum PI, Ogilvy RD, Hollands J (2006) Electrical resistivity tomography applied to geologic, hydrogeologic, and engineering investigations at a former waste disposal site. Geophysics 71:B231
Clement R, Oxarango L, Descloitres M (2011) Contribution of 3-D time-lapse ERT to the study of leachate recirculation in a landfill. Waste Manag 31:457–467
Depountis N, Harris C, Davies MCR, Koukis G, Sabatakakis N (2005) Application of electrical imaging to leachate plume evolution studies under in-situ and model conditions. Environ Geol 47:907–914
Devaraj N, Chidambaram S, Panda B, Thivya C, Thilagavathi R, Ganesh N (2018) Geo-electrical approach to determine the lithological contact and groundwater quality along the KT boundary of Tamilnadu, India. Model Earth Syst Environ 4:269–279
Dolk H, Vrijheid M, Armstrong B, Abramsky L, Bianchi F, Garne E, Nelen V, Robert E, Scott JES, Stone D, Tenconi R (1998) Risk of congenital anomalies near hazardous-waste landfill sites in Europe: the Eurohazcon study. Lancet 352:423–427
Fatoba JO, Eluwole AB, Sanuade OA, Hammed OS, Igboama WN, Amosun JO (2020) Geophysical and geochemical assessments of the environmental impact of Abule-Egba landfill, southwestern Nigeria. Model Earth Syst Environ. https://doi.org/10.1007/s40808-020-00991-8
Frid V, Liskevich G, Doudkinski D, Korostishevsky N (2008) Evaluation of landfill disposal boundary by means of electrical resistivity imaging. Environ Geol 53:1503–1508
Gallas JDF, Taioli F, Filho WM (2011) Induced polarization, resistivity, and self-potential: a case history of a contamination evaluation due to landfill leakage. Environ Earth Sci 63:251–261
Gautam PK, Biswas A (2016) 2D geo-electrical imaging for shallow depth investigation in Doon Valley Sub-Himalaya, Uttarakhand, India. Model Earth Syst Environ 2:1–9
Gawande NA, Reinhart DR, Thomas PA, McCreanor PT, Townsend TG (2003) Municipal solid waste in situ moisture content measurement using an electrical resistance sensor. Waste Manag 23:667–674
Genelle F, Sirieix C, Riss J, Renié S, Bégassat P (2012) Electrical resistivity monitoring on an experimental landfill clayey cover. In: Proceedings of the Near Surface Geoscience 2012—18th European meeting of environmental and engineering geophysics, Paris, France
George NJ, Ekanem AM, Ibanga JI, Udosen NI (2017) Hydrodynamic implication of Aquifer Quality Index (AQI) and flow zone (F21) in ground water abstraction: a case study of coastal hydro-lithofacies in South-eastern Nigeria. J Coast Conserv 21:759–776
Grellier S, Guerin R, Aran C, Robain H, Bellier G (2004) Geophysics applied to a bioreactor during leachate recirculation and to leachate samples. In: Conference proceedings of the 17th EEGS symposium on the application of geophysics to engineering and environmental problems, Feb 2004, cp-186-00062
Grellier S, Reddy KR, Gangathulasi J, Adib R, Peters CC (2007) Correlation between electrical resistivity and moisture content of municipal solid waste in bioreactor landfill. Geotechnical special publication, vol 163. ASCE Press, Reston, pp 1–14
Grellier S, Guérin R, Robain H, Bobachev A, Vermeersch F, Tabbagh A (2008) Monitoring of leachate recirculation in a bioreactor landfill by 2D electrical resistivity imaging. J Environ Eng Geophys 13:351–359
Guerin R, Munoz ML, Christophe A, Laperrelle C, Hidra M, Drouart E, Grellier S (2004) Leachate recirculation: moisture content assessment by means of a geophysical technique. Waste Manag 24:785–794
Guy G, Best M, Spence G, Dosso S, Telmer K. (2000) EM and GPR mapping of bedrock fractures as conduits for contaminated groundwater flow in a landfill. In: Symposium on the application of geophysics to engineering and environmental problems 2000
Kamura K (2002) Relationships between electrochemical properties of leachate and resistivity of strata in the landfill site consisting mainly of combustion residuals. Environ Geol 41:537–546
Leroux V, Dahlin T, Svensson M (2007) Dense resistivity and induced polarization profiling for a landfill restoration project at Härlöv, Southern Sweden. Waste Manag Res 25:49–60
Lohva J, Jokinen T, Suppala I (2008) Airborne geophysical studies around landfill areas—case histories from Finland. In: Conference Proceedings of the Near Surface 2008—14th EAGE European meeting of environmental and engineering geophysics, Sep 2008, cp-64-00038
Martinho E, Almeida F (2006) 3D behaviour of contamination in landfill sites using 2D resistivity/IP imaging: case studies in Portugal. Environ Geol 49:1071–1078
Moreira C, Castro M, Gonsalez A, Cavallari F, Munhoz T, Pereira A (2014) Comparative analysis between biogas flow in landfill and electrical resistivity tomography in Rio Claro City, Brazil. J Geol Res. https://doi.org/10.1155/2014/845906
Naudet V, Revil A, Rizzo E, Bottero JY, Bégassat P (2004) Groundwater redox conditions and conductivity in a contaminant plume from geoelectrical investigations. Hydrol Earth Syst Sci 8:8–22
Oladapo MI, Akintorinwa OJ (2007) Hydrogeophysical study of Ogbese Southwestern Nigeria. Global J Pure Appl Sci 13:55–61
Olagunju E, Ariyibi E, Awoyemi M, Adebayo A, Dasho O, Adenika C (2017) Application of geochemical and geophysical approach to environmental impact assessment: a case study of Emirin active open dumpsite, Ado-Ekiti Southwestern Nigeria. Model Earth Syst Environ 3:1543–1555
Rosqvist H, Leroux V, Dahlin T, Svensson M, Lindsjo M, Mansson C, Johansson S (2011) Mapping landfill gas migration using resistivity monitoring. Waste Resour Manag 164:3–15
Udosen NI, George NJ (2018) A finite integration forward solver and a domain search inverse solver for electrical resistivity tomography. Model Earth Syst Environ 4:1–12
Udosen NI, Potthast RWE (2018) Automated optimization of electrode locations for electrical resistivity tomography. Model Earth Syst Environ 4:1059–1083
White E, Day-Lewis F, Johnson CD, Lane J (2016) Application of frequency and time-domain electromagnetic surveys to characterize hydrostratigraphy and landfill construction at the Amargosa Desert Research Site, Beatty, Nevada. In: Symposium on the application of geophysics to engineering and environmental problems 2015
Yoon GL, Oh H, Park J (2002) Laboratory study of landfill leachate effect on resistivity in unsaturated soil using cone penetrometer. Environ Geol 43:18–28
Zume JT, Tarhule A, Christenson SC (2006) Subsurface imaging of an abandoned solid waste landfill site in Norman, Oklahoma. Ground Water Monit Rem 26:62–69
Author information
Authors and Affiliations
Corresponding author
Additional information
Publisher's Note
Springer Nature remains neutral with regard to jurisdictional claims in published maps and institutional affiliations.
Rights and permissions
About this article
Cite this article
Udosen, N.I. Geo-electrical modeling of leachate contamination at a major waste disposal site in south-eastern Nigeria. Model. Earth Syst. Environ. 8, 847–856 (2022). https://doi.org/10.1007/s40808-021-01120-9
Received:
Accepted:
Published:
Issue Date:
DOI: https://doi.org/10.1007/s40808-021-01120-9