Abstract
Water is a vital resource for life and the nucleus of everything on the globe. Nowadays, water is becoming a scarce resource, which is a menace to humankind and its future. Due to disparity in global water distribution, regions are suffering from acute water inability while others have a surplus. Water resources have to be prudently managed as they are beneficial for humanity. This study used the shuttle radar topography mission digital elevation model data to perform a detailed morphometric analysis of the Riyadh urban area. Generally, analyzing the morphometric parameters involves linear, areal, and relief aspects; therefore, the paper covered these parameters. The morphometric analysis results revealed that the Riyadh metropolitan area delineated into 40 watersheds and has eighth-order streams with a total area of 8500 km2; although the city area is 6570 km2 according to the administrative division. Consistent with statistical analysis, stream order is inversely related to the stream number with the coefficient of determination (R2) being 99%. Meanwhile, the cumulative stream length is directly proportional to the stream order with the same R2 value. Moreover, a high bifurcation ratio in most watersheds refers to less permeable rocks with a steeper slope. Consequently, the bifurcation ratio conforms to the high drainage density of the city (1.57 km/km2) and confirms the impermeability of subsurface material and mountainous relief. The Riyadh hypsometric integral is 38%, while the erosion integral value is 62%. Both values indicate that the basin is at a mature stage.
Similar content being viewed by others
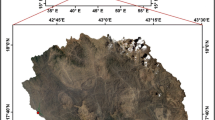
Avoid common mistakes on your manuscript.
Introduction
Water is a vital-scarce resource of life and its misuse threatens to humanity, both now and in the future. The disparity in water distribution creates an acute water deficit in many regions and a surplus in others. This surplus may occur during rapid and extreme rainfall events over a short period; thus, it has a twofold effect. This results in a massive amount of water leading to catastrophes and destruction from the inability of flood control systems to accommodate enormous volumes of water during the storms. This massive amount of water may impact different regions, including agricultural, industrial and urban areas. The surplus is beneficial when the harvested rainwater can be collected and used for different applications. Therefore, water resources need to be carefully managed due to their benefits for humankind. Sustainable development aims at conserving and developing natural resources. Developing the watershed is the backbone of natural resource management (land and water), that additionally mitigates the effects of natural disasters such as floods. Different factors influence the watershed development including the available water, soil type, physiography, geomorphology, land use/land cover and drainage density. To realize such benefits, several different approaches are used in watershed management. One of these is analyzing the watershed morphometric parameters. Furthermore, these morphometric parameters enable the decision-makers to gather useful information about the topographical, geological and hydrological characteristics of the watershed.
Morphometry represents the topographic relief of the earth’s surface in a mathematical way (Clarke 1966; Nongkynrih and Husain 2011; Bhunia et al. 2012; Hajam et al. 2013; Biswas et al. 2014; Withanage et al. 2015). The morphometric analysis is performed by measuring the linear, areal, relief and watershed gradients (Magesh et al. 2013; Ali and Ali 2014; Kaliraj et al. 2015; Ali et al. 2016). The widely recognized watershed morphometry concept is that the basin morphology impinges on the different dynamic geomorphologic and geologic mechanisms. Nonetheless, the impact of drainage morphometry is primary for understanding the terrain with the soil’s physical and erosional properties (Bagyaraj et al. 2011; Dutta and Roy 2012). Accordingly, the morphometric analysis is critically important to illustrate the basin’s hydrological characteristics. Consequently, the results of the watershed morphometric analysis are crucial in integrated water resource management.
Regardless of the existence of soil type maps, morphometric parameters (linear, areal and relief) can be utilized as a significant tool in micro-watersheds prioritization (Biswas et al. 1999). Drainage patterns refer to spatial relationships between streams or rivers, which may be influenced in their erosion by inequalities of the slope, soils, rock resistance, structure and geologic history of a region. Studying the morphological characteristics of streams has been instrumental in measuring different significant properties such as biological, physical and chemical. Apart from the identification of watershed morphometric parameters, it is crucial to study the morphometric aspects in several other environmental trends such as thermal stability, heat content, erosion, chemical mass, nutrient loading rates, growth and biological productivity. For instance, Hayes and Anthony (1964) found that there is a positive relationship between fish productivity and lake mean depth and surface area for 150 North American lakes. (Schindler 1971) interpreted that lake surface area, drainage area and volume have implications on alimentary differences in Ontario lakes. Besides, the lake morphology reflects its geological descent.
Watershed morphometric analysis along with the prevailing climate, geology and basin geomorphology assists in elucidating more information about the basin’s linear, areal and relief parameters (Pareta and Pareta 2011; Pareta and Pareta 2012; Rai et al. 2014; Pandey and Das 2016). Linear aspects include the stream order, stream number, stream length, mean stream length, stream length ratio, bifurcation ratio and length of overland flow. The areal aspects involve the basin length, perimeter, area and form factor, elongation ratio, circularity ratio, drainage density, stream frequency, drainage texture and infiltration rate. Meanwhile, the relief aspects encompass the total relief, relative relief, relief ratio, hypsometric analysis and gradient ratio.
There are two approaches (traditional and modern) to analyze the morphometric parameters. The conventional approach is based on topographic maps and manual calculations. Horton (1945) proposed empirical relations between the landform characteristics and the controlling variable of climate, hydrology, vegetation and soil properties. These relationships were the cornerstone of the quantitative description process of the drainage basin network and form, as well as their interrelationships. However, the modern approach depends on the complementarity of remote sensing techniques (RST) and geographic information systems (GIS) (Kant et al. 2015). This can improve the analysis accuracy and save time. RST and GIS are the latest sophisticated tools for achieving the sustainable development of watercourses and water resources via management, watershed development and micro-watershed prioritization. There are different useful remote sensing data sources, such as the shuttle radar topographic mission (SRTM) and the advanced space-borne thermal emission and reflection radiometer (ASTER). These missions provide accurate, rapid and economic data for analyzing the hydrological systems compared to traditional methods. Watershed characteristics were produced using the complimentary SRTM digital elevation model (DEM). Numerous morphometric studies have been conducted in several countries (Rao et al. 2010; Nongkynrih and Husain 2011; Kumar 2013; Waikar and Nilawar 2014; Gabale and Pawar 2015; Kalam and Ramesh 2015; Asode et al. 2016).
This study aims at managing the urban watershed by identifying the morphometric parameters of the Riyadh metropolitan area based on the DEM data via the GIS platform, along with the delineation of studied watersheds. The analysis will help decision-makers to understand the hydrological, geological and topographical characteristics of the study area to constitute an intense initiation for all future studies on the urban watershed.
Site descriptions
Riyadh city (latitude 24°13′51″ N to 25°10′30′′ N and longitude 45°59′12′′ E to 47°20′29′′ E) is the capital of Saudi Arabia (Fig. 1). The Riyadh metropolitan area is 6570 km2 of which 1800 km2 urbanized area, while the watershed area is 8500 km2. Riyadh was selected to analyze the morphometric parameters, as it is an urban area with tremendous infrastructure with agricultural and industrial inter-sectoral collaboration. Moreover, it is among the fastest-growing cities in the world since it has vertical and horizontal expansion with a high population of 6.5 million people (Aina et al. 2008; Ashwan et al. 2012). Furthermore, the soil texture of Riyadh is varied, including sand, loam and gravel (Radwan et al. 2018). The current city climate features a hot-dry summer and a cool-moist winter. The average temperature reaches its highest (22–43 °C) from June to August and the lowest (8–22 °C) from December to February (Qhtani and Al Fassam 2011). The area is part of a semiarid zone that experiences a tropical climate (Radwan et al. 2019). Despite a deficit in annum average precipitation of about 62 mm, the city periodically experiences flash flooding (Rahman et al. 2016). However, in the recent past, the rainfall pattern has changed, resulting in downpours for hours to days causing a high volume of surface runoff. The flooding results in significant damage, including socioeconomic loss, soil erosion, destroyed infrastructure, land degradation, vegetation loss, the inundation of cities and the loss of lives (Bajabaa et al. 2014; Špitalar et al. 2014).
Materials and methods
Morphometry is a mathematical description of the earth’s surface and reflects the nature of its configuration. The watersheds in the Riyadh metropolitan area were delineated based on DEM data obtained from the US Geological Survey web site. The 1-ARC SRTM DEM with a 30 m accuracy was employed in the city watershed delineation (Fig. 2). The DEM publication date was September 23, 2014 and was updated on August 6, 2015. A watershed is a basin-like landform. There are two approaches for extracting the watershed network; the traditional approach is to extract the watershed network and manually identify the stream order, which takes considerable time and effort. Whereas, the modern approach is to obtain the watershed automatically. The watersheds were delineated automatically using the DEM digital processing by the hydrology tool incorporated in ArcGIS 10.4. The processing includes DEM filling, identifying flow direction and flow accumulation, setting conditional, stream order, and also converting the stream order from raster into vector data (Fig. 3). To ensure consistency, the watershed borders were verified by overlaying it on Google Earth Pro software. Additionally, the DEM was used to create the slope map and contour map in Figs. 4 and 5, respectively.
This work was carried out through georeferenced satellite data to analyze the morphometric parameters. Accordingly, the morphometric parameters were identified for every watershed based on mathematical formulas and GIS platform measurements using the SRTM DEM. The morphometric parameters were divided into three categories: (1) linear aspects, (2) areal aspects and (3) relief aspects. The linear aspects involve the x-axis calculations of the stream properties. These aspects include the stream order, stream number, stream length, mean stream length, stream length ratio, bifurcation ratio and length of overland flow. The parameters of stream properties are calculated using the standard methods and formulae presented in Table 1. The areal morphometric analysis includes the two-dimensional parameters involved in the x- and y-axes calculations of the drainage basin. Some of these aspects are determined by the DEM processing in the GIS environment, such as the basin length, basin perimeter and basin area. Whereas, the remaining areal variables such as the basin form factor, elongation ratio, circularity ratio, drainage density, stream frequency, drainage texture and infiltration rate are computed using the formula in Table 1. The relief morphometric analysis considers the three-dimensional parameters concerned with the x-,y- and z-axes calculations. These variables, such as the total relief, relative relief, relief ratio, hypsometric analysis and gradient ratio, are found in Table 1.
Moreover, the relationship between the watershed land area and its altitude has been represented by the hypsometric curve. It is calculated by dividing the watershed area by the number of altitude classes in that watershed area. Furthermore, all maps were projected onto the World Geodetic System 1984 Universal Transverse Mercator (WGS 84 UTM) Zone 38 Coordinate System.
Results and discussion
The Riyadh metropolitan area was delineated automatically into 40 watersheds as shown in Fig. 6 via the GIS platform based on the high-resolution quality of the DEM. The detailed morphometric parameters results are mentioned below:
Linear morphometric parameters
The linear aspects of a watershed are mainly related to the drainage network pattern that is influenced by the topographic characteristics (Radwan et al. 2017). The following is the detailed information for each linear aspect. The determination of the stream order (u) constitutes the first step in the drainage watershed analysis. The literature considers several ordering methods for the classification (Gravelius 1914; Horton 1945; Strahler 1957; Scheidegger 1965; Shreve 1967). The straightforward method proposed by Strahler (1964) is adopted in this study. The Strahler method assigns the smallest stream segment as first order; where two such streams join, a stream segment of the second order is generated. When two streams of the second-order join, this generates a stream segment of the third order and so on. When streams of two different orders join, the higher order is assigned. Figure 6 depicts the stream order classification of the Riyadh metropolitan area watersheds.
It is noted that the lowest stream order has the maximum number of streams and vice versa. The Riyadh metropolitan area is delineated into 40 watersheds as shown in Figs. 7, 8, 9 and 10. These watersheds vary in stream order, size and all morphometric parameters. Overall, the city is classified as an eighth-order drainage basin. The stream order controls the watershed size and hierarchy. The stream order of the city delineated watersheds ranging from the third to seventh order as presented in Table 2. Watersheds 12 and 17 are of seventh order, while the minimum order (third order) is located in watersheds 13, 16 and 32 to 40 except 34.
The stream count of the same order (stream number, Nu) is based on the method proposed by Horton (1945). Figure 11 shows an inverse relationship between the stream order and stream number with a coefficient of determination (R2) of 99% for the basin area. Therefore, the total number of streams gradually decreases with the increase in stream order. This figure shows a geometric series agreeing with and obtained by Horton’s law. The series begins with a single stream for the highest order and increases according to a constant bifurcation ratio. The total number of streams ranges from 1 to 25,322 for the entire city for the eighth and first order, respectively. The detailed stream numbers for every order in Riyadh city are presented in Table 3. According to Table 2, to summarize the watershed stream numbers, the watersheds with more than 1000 streams are 9, 12, 14, 15, and 17, while watersheds 4, 6 to 8, 10, and 18 to 25 except 21, have 100 to 500 streams. The rest of the watersheds have less than 100 streams. The first and second stream orders represent about 95% of the streams in the studied watersheds. Whereas, the higher stream orders constitute the remaining 5% of the streams number. These results are important in investigating the watershed characteristics like drainage pattern (Waikar and Nilawar 2014; Kant et al. 2015) that is a good indicator of the impermeability and infiltration capacity (Pandey and Das 2016).
Stream length (Lu) is a very significant hydrological feature of the watershed and can be considered as an indicator of surface runoff characteristics. The stream length is based on Horton’s law proposed by Horton (1945). Consequently, the stream length was measured from the basin mouth to drainage divide (Kant et al. 2015; Pandey and Das 2016). Generally, the longer stream lengths are an indicator of a flatter gradient (Pandey and Das 2016). The maximum stream length is concentrated in the first-order streams, which decreases with increasing stream order (Strahler 1964). The length of the first and second stream orders cover about 94% of the total stream length, whereas the length of the remaining stream orders covers less than 6%. The main basin stream length ranges from 0.209 to 11,305.5 km for the eighth- and first-order streams, respectively. Table 2 presents the delineated watersheds’ total stream length, which ranges from 2.96 to 7548.31 km for watersheds 37 and 17, respectively.
The mean stream length (Lsm) was calculated by dividing the total stream length for any order by the number of streams for the same order. This is closely related to the drainage network and associated surfaces (Strahler 1964). The mean stream length ranges from 0.021 to 0.627 km for the fifth- and third-order streams in the main basin, respectively. The cumulative stream length for the study area is 2.286 km; it is noted that the mean stream length increases with increasing stream order. Additionally, the cumulative stream length of the watersheds is augmented by increasing the stream order (with a coefficient of determination (R2) of 94%) (Fig. 12). The coefficient of determination confirms the precision of the results in delineating watersheds and extracting the drainage basin network. The closer R2 value is to one, the better is the quality of the extraction. For consistency, these results are matched to Horton’s law. The stream length ratio of the study area for different stream orders ranges from 0.1 to 8 for the sixth and fifth order, respectively. Meanwhile, the main stream length for delineated watersheds ranges from 0.86 to 111.17 km for watersheds 35 and 12, respectively, as presented in Table 2. However, the presence of moderately resistant rocks, high slopes and topography is observed in the terrain, furthermore these conclusions in accordance with the important indications of the results obtained by Bindu et al. (2012); Pandey and Das (2016).
The ratio between the number of streams of a particular order and the number of streams of the next higher order (bifurcation ratio, Rb) is estimated based on Schumm (1956). The bifurcation ratio (Rb) ranges from 2 to 5.636 for the seventh and fifth order, respectively; the entire city has a mean Rb of 4.45. The results of different studies indicate that the mean bifurcation ratio ranges from 3 to 5 for watersheds without differential geologic control (Coates 1958; Kant et al. 2015; Pandey and Das 2016). In accordance with Table 2, the bifurcation ratio of all delineated watersheds is within the normal range excluding the following watersheds 15, 30, 32, 33, 35, 37, and 38. A higher mean bifurcation ratio value suggests the presence of less permeable rocks with a high slope, while a lower bifurcation ratio value suggests porous rocks with less structural control (Pareta and Pareta 2011; Pareta and Pareta 2012; Pandey and Das 2016).
The length of overland flow in Riyadh city is 0.32 km, while the length of the overland flow for studied watersheds ranges from 0.175 to 2 km for watersheds 13 and 4, respectively, as presented in Table 2. These values are considered in the hydrologic and physiographic development of watershed areas (Waikar and Nilawar 2014). This independent variable can substantially affect the quantity of the water required to exceed a certain threshold of erosion. Generally, a smaller length of overland flow indicates that the basin may have a higher flood hazard potential (Shi 2014).
Areal morphometric parameters
The areal morphometric parameters detect essential features for the watershed drainage, including the geological structure, climatic conditions and watershed denudation history. The aspects considered in this study are the basin length, basin perimeter, basin area, basin form factor, elongation ratio, circularity ratio, drainage density, stream frequency, drainage texture and infiltration rate. The detailed areal results for every watershed in the Riyadh city are presented in Table 4.
The studied watershed area ranges from 1.29 and 2944.6 km2 for watersheds 37 and 17, respectively. 7.5% of the total watersheds have areas greater than 1000 km2. The area ranges from 100 to 500 km2 located in 10% of the total watershed area, while 37.5% of the whole watersheds have areas ranging from 20 to 100 km2. About 20% of the entire watershed area covers less than 10 km2. The remaining watersheds range from 10 to 20 km2 in area. Generally, the increase in the basin area raises the share of precipitation received and, consequently, the amount of surface water. The length of the watersheds ranges from 1.82 to 116.9 km for watersheds 37 and 12, respectively. The perimeter of the watersheds ranges from 5.9 to 545.7 km for watersheds 37 and 12, respectively. It can be inferred from Table 4 that the watersheds are an elongated area, which reflects their hydrologic characteristics. Elongated watersheds have higher peak flows with longer lag times. Watershed shape is defined by the elongation and circularity ratios (Kant et al. 2015).
There are three essential ratios considered in the study that affect the hydrologic characteristics of the watershed: the form factor (Rf), elongation ratio (Re) and circularity ratio (Rc). They are used to describe the shape of the drainage basin and are calculated according to formulae by Horton (1932), Schumm (1956) and Miller (1953), respectively. A lower form factor value indicates that the watershed will be more elongated. According to Horton (1932), the form factor values would never exceed 0.75. The Riyadh form factor is 0.62, with the watersheds’ values ranging from 0.13 to 0.62 for watersheds 32 and 7, respectively. Watersheds 7 and 31 have form factors ranging from 0.4 to 0.6, respectively, and the remaining watersheds have values less than 0.4. Hence, in accordance with form factor values, the delineated watersheds are within the Horton range. Basins that have low form factors have a longer flow direction (Kant et al. 2015; Pandey and Das 2016). Consequently, the aforementioned results confirm that the watersheds are elongated, which are consistent with the area and perimeter inferences. Furthermore, the watershed is more prone to massive peak flows with a longer duration. Floods are easier to control in elongated basins than in circular basins (Pareta and Pareta 2011; Pareta and Pareta 2012; Kumar 2013; Waikar and Nilawar 2014; Pandey and Das 2016). The main basin elongation ratio is 0.889. Thus, the study area shape is oval and this outcome is quite straightforward in Fig. 1. The elongation ratio ranges from 0.399 to 0.891 for watersheds 32 and 7, respectively. Watershed 7 is oval (0.8 to 0.9). The less elongated watersheds are 1, 2, 31, 35 and 37 (0.7 to 0.8), meanwhile watersheds 12, 15, 18, 27 to 29 and 32 are more elongated (< 0.5). The shape of the remaining watersheds is elongated (0.5 to 0.7). The circularity ratio of the entire city is 0.2; however, the watersheds’ values range from 0.1 to 0.57 for watersheds 12 and 31, respectively. Table 4 presents detailed values of form factor, elongation ratio and circularity ratio. Generally, these values confirm that the delineated watersheds are elongated. An elongated basin tends to have fewer flood hazards compared to circular basins because of longer flow durations and smaller peak discharges (Shi 2014).
Generally, the drainage density and drainage texture are influenced by the terrain, land use/land cover and soil in the basin area. Figure 13 depicts the study area’s drainage density. Drainage density reflects the basin response to runoff processes and is generally affected by surface geological formations and vegetation. The Riyadh drainage density is 1.57 km/km2, with the watersheds’ values ranging from 2.3 to 2.9 km/km2 for watersheds 37 and 13, respectively. These values are very high. A high drainage density indicates greater and faster runoff from rainfall events, while a low drainage density suggests that precipitation infiltrates the ground, and only a few stream channels are needed to transport the runoff. The stream frequency ranges from 2.38 to 6.2 for watersheds 33 and 37, respectively. This range indicates that the watersheds are characterized by low vegetation and infiltration capacity with high relief and runoff. The values of drainage density, drainage texture and stream frequency indicate that the main basin has high impermeability and runoff with marked lithology. The infiltration number computed by the Zavoiance (1985) formula for the study area is 6. The infiltration number ranges from 6.8 to 14.1 for watersheds 33 and 37, respectively. Thus, these values confirm the results for runoff and infiltration capacity.
Relief morphometric parameters
Relief morphometry is critical in studying the watershed erosional characteristics (Sreedevi et al. 2009; Magesh et al. 2011; Rai et al. 2014; Vieceli et al. 2015; Al-Saady et al. 2016). In this study, the three morphometric relief parameters used are the watershed relative relief (R), relief ratio (Rh) and gradient ratio (Rg). They are inherently an indication of the progressive changes in the landforms. For transparency reasons, the detailed relief results for every watershed in the Riyadh city have been presented in Table 5. Relative relief (R) values have been estimated by the Melton (Melton 1957) equation, as mentioned under the relief section in Table 1. The maximum relief of the entire city is 985 m, and the watersheds range between 535 and 985 m for watersheds 1 and 17, respectively. In Riyadh, the minimum relief is 431 m, and the watersheds range between 431 to 648 m for watersheds 14 and 19, respectively. The mean relief value of the main basin is 742 m, and the watershed values range 491 to 742 m for watersheds 2 and 17, respectively. The mean relief has been calculated according to the Strahler (1952) formula. While the relative relief of the main basin is 0.07, the values of the watersheds range from 0.035 to 1.72 for watersheds 12 and 35, respectively. The low relative relief value indicates the presence of less resistant rocks in the drainage basin (Magesh et al. 2011; Pandey and Das 2016).
The relief ratio (Rh) of the city is 0.005, and the values of the watersheds range from 0.002 to 0.052 for watersheds 12 and 37, respectively. This indicates an inverse relationship between the watershed area and the watershed size. The low value suggests the existence of a gentle slope in the terrain. The gradient ratio (Rg) ranges from 0.002 to 0.155 for watersheds 12 and 35, respectively, according to the Sreedevi et al. (2005) formula. The results indicate that the watersheds have a homogeneous lithology and a lack of structural control.
Hypsometric analysis
The increasing importance of the hypsometric integral (HI) is demonstrated in regions that differ in the developmental stage and geologic structure. Resultantly, the hypsometric integral value for the youth stage is significant and decreases gradually toward the old age stage. The hypsometric characteristics of Riyadh were calculated by dividing the land area for every watershed into many classes based on the contour map with the aid of the triangulated irregular network (TIN) map. According to the hypsometric curve shown in Fig. 14, it is estimated that 65% of the area falls within an elevation of 630 m, and 20% of the surface of the watershed area is above an elevation of 750 m.
The hypsometric curve has been used to obtain the HI by relating the mean elevation and relief range of the basin. It is inversely correlated with total relief, slope steepness, drainage density and channel gradients. The HI value has been validated by the Pike and Wilson (1971) formula. The integral value for the study area is estimated at 38%, and the erosion integral (EI) is 62%. This reflects the distribution of a major volume of the landmass of 3727 km2 at relatively low elevations (< 670 m). The HI value indicates that the basin is at a mature stage, which means that the basin is in the erosion stage. The following hypothetical standards have been recognized for determining the geologic stages of the basin (Table 6) according to Pareta and Pareta (2012); Radwan et al. (2017).
Slope and watershed profile
Figure 4 shows the slope map of Riyadh that varies from 0 to 52° with an average of 4°. The map depicts that the slope is categorized into five classes: very low (< 2.5°), low (2.5°–6.5°), medium (> 6.5°–12.5°), high (> 12.5°–20.5°) and very high (> 20.5°). This confirms the nature of the runoff and infiltration mentioned above in the linear and areal morphometric parameters for watersheds. The city slopes from west to southeast and to south direction, which appears in the slope and contour maps (Figs. 4 and 5). Longitudinal profile value expresses the altitude for a specific point at the mainstream watershed from sea level in meters. Moreover, the importance of the longitudinal profile is to validate the mainstream selection quality. The maximum mainstream altitude should be at the watershed source, while the minimum mainstream altitude should be at the watershed mouth (outlet). The study area’s longitudinal profile ranges from 441 to 823 m, as shown in Fig. 15. According to Table 7, the maximum longitudinal profile of the delineated watersheds ranges from 529 to 823 m for watersheds 1 and 12, respectively, with a mean of 755 m. The minimum value ranges from 431 to 648 m for watersheds 14 and 19, respectively, with a mean of 540 m. This is considered to be the best way to geometrically represent the watershed forms (Pareta and Pareta 2011).
Conclusions
Many water resources such as watersheds and wadis must be managed wisely. Accordingly, proper watershed management requires an extensive morphometric study of the basin. This morphometric analysis helps decision-makers understand many basin characteristics, such as the underlying lithology, infiltration capacity, runoff, basin shape and size. A combination of RST and GIS based on the DEM was used to provide maximum accuracy for the analysis. The morphometric parameters, including the linear, areal and relief aspects were analyzed for urban watersheds of the Riyadh metropolitan area. Based on this analysis, the stream order of the city was found to be of the eighth order. The study revealed that the lower order is the most dominant. This points to the adequacy of the watersheds to provide sufficient superficial draining networks containing a high number of low-order streams. The linear aspects indicate the presence of impermeable rocks with a steep slope. The areal aspects indicate that most of the watershed shapes are elongated with high runoff, low infiltration capacity, high impermeability of the underlying lithology, high relief, and lack of vegetation. Due to these characteristics, the watersheds tend to have more massive floods with a longer duration of flow compared to circular watersheds. The relief aspects indicate the presence of less resistant rocks in the drainage basin. In the end, it can be emphasized the effectiveness of using the integration of geographic information systems and remote sensing in determining the physical characteristics of urban watersheds. And thereby overcome the absence of ground data for these catchments due to its spread over large areas. This means that this integration provides a simple method with reasonable accuracy and low costs.
References
Aina YA, Merwe J, Alshuwaikhat HM (2008) Urban spatial growth and land use change in Riyadh: Comparing spectral angle mapping and band ratioing techniques. Paper presented at the Proceedings of the Academic Track of the 2008 Free and Open Source Software for Geospatial (FOSS4G) Conference, incorporating the GISSA 2008 Conference, Cape Town, South Africa,
Ali U, Ali SA (2014) Analysis of drainage morphometry and watershed prioritization of Romushi-Sasar catchment. Kashmir valley, India using remote sensing and GIS technology. J Adv Res 2:5–23
Ali SA, Alhamed M, Ali U (2016) Morphometric analysis of Abdan Basin, Almahfid Basement Rock, Yemen: using remote sensing and GIS. Int J Adv Remote Sens GIS, pp 1605–1617
Al-Saady YI, Al-Suhail QA, Al-Tawash BS, Othman AA (2016) Drainage network extraction and morphometric analysis using remote sensing and GIS mapping techniques (Lesser Zab River Basin, Iraq and Iran). Environ Earth Sci 75:1243
Ashwan MSA, Salam AA, Mouselhy MA (2012) Population growth, structure and distribution in Saudi Arabia. Humanit Soc Sci Rev 1:33-46
Asode AN, Sreenivasa A, Lakkundi T (2016) Quantitative morphometric analysis in the hard rock Hirehalla sub-basin. Bellary and Davanagere districts, Karnataka, India using RS and GIS. Arab J Geosci 9:1–14
Bagyaraj M, Gurugnanam B, Nagar A (2011) Significance of morphometry studies, soil characteristics, erosion phenomena and landform processes using remote Sensing and GIS for Kodaikanal Hills, a global biodiversity hotpot in Western Ghats. Dindigul District, Tamil Nadu, South India. Res J Environ Earth Sci 3:221–233
Bajabaa S, Masoud M, Al-Amri N (2014) Flash flood hazard mapping based on quantitative hydrology, geomorphology and GIS techniques (case study of Wadi Al Lith, Saudi Arabia). Arab J Geosci 7:2469–2481
Bhunia GS, Samanta S, Pal B (2012) Quantitative analysis of relief characteristics using space technology. Int J Phys Soc Sci 2:350–365
Bindu G, Neelakantan R, Reghunath R (2012) Remote sensing cum GIS based assessment of morphometric characteristics of Chittar basin in district Thiruvananthapuram. Kerala, South India. Nat Environ Poll Technol 11:729–736
Biswas S, Sudhakar S, Desai V (1999) Prioritization of sub-watersheds based on morphometric analysis of drainage basin, district Midnapore West Bengal. J Indian Soc Remote Sens 27:155–166
Biswas A, Das Majumdar D, Banerjee S (2014) Morphometry governs the dynamics of a drainage basin: analysis and implications. Geogr J 2014:1–14
Clarke JI (1966) Morphometry from Maps. Essays in geomorphology. Elsevier Publ. Co., New York
Coates DR (1958) Quantitative geomorphology of small drainage basins of southern Indiana. Technical report (columbia university department of geology); no 10
Dutta S, Roy S (2012) Determination of erosion surfaces and stages of evolution of Sangra drainage basin in Giridih district. Jharkhand, India. Int J Geomat Geosci 3:63–73
Gabale SM, Pawar NR (2015) Quantitative morphometric analysis of AmbilOdha (Rivulet) in Pune, Maharashtra, India IOSR. J Environ Sci Toxicol Food Technol 9:41–48
Gravelius H (1914) Flusskunde, Goschen’sche Verlagshandlung. Berlin. vol 1. GJ göschen,
Hajam RA, Hamid A, Bhat S (2013) Application of morphometric analysis for geo-hydrological studies using geo-spatial technology: a case study of Vishav drainage basin. Hydro Curr Res
Hayes F, Anthony E (1964) Productive capacity of North American lakes as related to the quantity and the trophic level of fish, the lake dimensions, and the water chemistry. Trans Am Fish Soc 93:53-57
Horton RE (1932) Drainage‐basin characteristics Eos. Trans Am Geophysical Union 13:350–361
Horton RE (1945) Erosional development of streams and their drainage basins; hydrophysical approach to quantitative morphology. Geol Soc Am Bull 56:275–370
Kalam M, Ramesh M (2015) Morphometric analysis of Milli watershed area in Zaheerabad. Int J 3:97–101
Kaliraj S, Chandrasekar N, Magesh N (2015) Morphometric analysis of the River Thamirabarani sub-basin in Kanyakumari district. South west coast of Tamil Nadu, India, using remote sensing and GIS. Environ Earth Sci 73:7375–7401
Kant S, Meshram S, Dohare R, Singh S (2015) Morphometric analysis of Sonar sub-basin using SRTM data and geographical information system (GIS) Afr J Agric Res 10:1401-1406
Kumar N (2013) Morphometric analysis of river catchments using remote sensing and GIS (a case study of the Sukri River, Rajasthan). Int J Sci Res Publ 3:1–6
Magesh N, Chandrasekar N, Soundranayagam JP (2011) Morphometric evaluation of Papanasam and Manimuthar watersheds, parts of Western Ghats. Tirunelveli district, Tamil Nadu, India: a GIS approach. Environ Earth Sci 64:373–381
Magesh N, Jitheshlal K, Chandrasekar N, Jini K (2013) Geographical information system-based morphometric analysis of Bharathapuzha river basin. Kerala, India. Appl Water Sci 3:467–477
Melton MA (1957) An analysis of the relations among elements of climate, surface properties, and geomorphology. Columbia Univiersity, New York
Miller VC (1953) A quantitative geomorphic study of drainage basin characteristics in the clinch mountain area virginia and tennessee. Columbia Univiersity New York
Nongkynrih JM, Husain Z (2011) Morphometric analysis of the Manas river basin using earth observation data and geographical information system. Int J Geomat Geosci 2:647–654
Pandey PK, Das S (2016) Morphometric analysis of Usri River basin. Chhotanagpur Plateau, India, using remote sensing and GIS Arabian journal of geosciences 9:1–13
Pareta K, Pareta U (2011) Quantitative morphometric analysis of a watershed of Yamuna basin, India using ASTER (DEM) data and GIS. Int J Geomat Geosci 2:248
Pareta K, Pareta U (2012) Quantitative geomorphological analysis of a watershed of Ravi River basin. HP India Int J Remote Sens GIS 1:47–62
Pike RJ, Wilson SE (1971) Elevation-relief ratio, hypsometric integral, and geomorphic area-altitude analysis. Geol Soc Am Bull 82:1079-1084
Qhtani AM, Al Fassam AN (2011) Ar Riyadh Geospatial urban information system and metropolitan development strategy for Ar Riyadh. In: Paper presented at the ESRI international user conference
Radwan F, Alazba A, Mossad A (2017) Watershed morphometric analysis of Wadi Baish Dam catchment area using integrated GIS-based approach. Arab J Geosci 10:256
Radwan F, Alazba AA, Mossad A (2018) Estimating potential direct runoff for ungauged urban watersheds based on RST and GIS. Arab J Geosci 11:748. https://doi.org/10.1007/s12517-018-4067-4
Radwan F, Alazba AA, Mossad A (2019) Flood risk assessment and mapping using AHP in arid and semiarid regions. Acta Geophys 67:215–229. https://doi.org/10.1007/s11600-018-0233-z
Rahman MT, Aldosary AS, Nahiduzzaman KM, Reza I (2016) Vulnerability of flash flooding in Riyadh, Saudi Arabia. Nat Hazards 84:1807–1830
Rai PK, Mohan K, Mishra S, Ahmad A, Mishra VN (2014) A GIS-based approach in drainage morphometric analysis of Kanhar River Basin, India. App Water Sci 1-16
Rao NK, Latha SP, Kumar AP, Krishna HM (2010) Morphometric analysis of Gostani river basin in Andhra Pradesh state. India using spatial information technology. Int J Geomat Geosci 1:179
Scheidegger AE (1965) The algebra of stream-order numbers United states geological survey professional paper, pp 187–189
Schindler D (1971) A hypothesis to explain differences and similarities among lakes in the Experimental Lakes Area, northwestern Ontario. J Fish Board Can 28:295–301
Schumm SA (1956) Evolution of drainage systems and slopes in badlands at perth amboy. New Jersey Geol Soc Am Bull 67:597–646
Shi Q (2014) Flood hazard assessment along the Western Regions of Saudi Arabia using GIS-based morphometry and remote sensing techniques, vol 10, pp 1–103. Master thesis. King Abdullah University of Science and Technology (KAUST)
Shreve RL (1967) Infinite topologically random channel networks. J Geol 178–186
Špitalar M, Gourley JJ, Lutoff C, Kirstetter P-E, Brilly M (2012) Carr N (2014) Analysis of flash flood parameters and human impacts in the US from 2006 to. J Hydrol 519:863–870
Sreedevi P, Subrahmanyam K, Ahmed S (2005) The significance of morphometric analysis for obtaining groundwater potential zones in a structurally controlled terrain. Environ Geol 47:412–420
Sreedevi P, Owais S, Khan H, Ahmed S (2009) Morphometric analysis of a watershed of South India using SRTM data and GIS. J Geol Soc india 73:543–552
Strahler AN (1952) Dynamic basis of geomorphology. Geol Soc Am Bull 63:923–938
Strahler AN (1957) Quantitative analysis of watershed geomorphology Eos. Trans Am Geophys Union 38:913–920
Strahler AN (1964) Quantitative geomorphology of drainage basin and channel networks. Handbook of applied hydrology
Vieceli N, Bortolin TA, Mendes LA, Bacarim G, Cemin G, Schneider VE (2015) Morphometric evaluation of watersheds in Caxias do Sul City, Brazil, using SRTM (DEM) data and GIS. Environ Earth Sci 73:5677–5685
Waikar M, Nilawar AP (2014) Morphometric analysis of a drainage basin using geographical information system: a case study. Int J Multidiscip Curr Res 2:179–184
Withanage N, Dayawansa N, De Silva R (2015) Morphometric analysis of the Gal Oya River basin using spatial data derived from GIS. Trop Agric Res 26:175–188
Zavoiance I (1985) Morphometry of drainage basins (Developments in water science). Elsevier, London 20:104–105
Acknowledgements
The project was financially supported by King Saud University, Vice Deanship of Research Chairs.
Author information
Authors and Affiliations
Corresponding author
Ethics declarations
Conflict of interest
On behalf of all authors, the corresponding author states that there is no conflict of interest.
Additional information
Publisher's Note
Springer Nature remains neutral with regard to jurisdictional claims in published maps and institutional affiliations.
Rights and permissions
About this article
Cite this article
Radwan, F., Alazba, A.A. & Mossad, A. Analyzing the geomorphometric characteristics of semiarid urban watersheds based on an integrated GIS-based approach. Model. Earth Syst. Environ. 6, 1913–1932 (2020). https://doi.org/10.1007/s40808-020-00802-0
Received:
Accepted:
Published:
Issue Date:
DOI: https://doi.org/10.1007/s40808-020-00802-0