Abstract
Grafted fresh market tomatoes are widely used in commercial production throughout Europe and Asia, and interest among commercial producers in the Americas has increased in recent years. Many field trials have found dramatic net economic return increases relative to non-grafted scion cultivars. However, optimal yields require growing conditions that satisfy the agronomic needs of both rootstock and scion cultivars. Most commercial rootstocks are resistant to multiple soilborne pathogens, allowing grafted plants to maintain high yields in pathogen-infested fields without the use of soil pesticides, including fumigants. Here we comprehensively and quantitatively review, for the first time, all available published trial data on fruit quality and yield of grafted tomatoes. Collectively, 159 publications included 202 different rootstocks, 126 geographic locations, and 1023 experimental treatments. Yield performance varies with the specific rootstock/scion combinations and with the conditions of a given production system. Among 949 heterograft treatments (rootstock/scion of different cultivars), grafted plant yields were not significantly higher in 65% of the cases, yet they averaged a 37% yield increase for all data. In addition, grafted/non-grafted yield ratios in 105 experimental treatments with rootstock ‘Maxifort’ varied dramatically by scion. However, European trials used completely different scions than US trials, so the roles of scion and geographical differences remain unclear. Concerns that grafting might contribute to inferior fruit quality (pH, titratable acidity, total soluble solids, lycopene, vitamin C, firmness, “taste”) seem unfounded in general, though isolated cases show dramatic differences. Grafted tomatoes show promise to reduce the usage of various soilborne pathogen treatments, with 33% of commercial tomato rootstocks either resistant or highly resistant to seven or more common soilborne pathogens. Our approach integrated trial data from around the world, though limitations in available data complicated our analysis of relationships between some experimental variables and fruit yields and quality.
Similar content being viewed by others
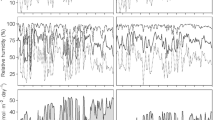
Avoid common mistakes on your manuscript.
1 Introduction
By splicing together plants of two different cultivars, grafting allows a grower to reap the benefits of both cultivars in a single plant (Fig. 1). While grafting has been widely used in agriculture for centuries, mainly in woody plants, its application to non-woody plants such as tomatoes is more recent (Kubota et al. 2008). In Europe and Asia, grafted tomatoes currently account for a substantial proportion of total tomato production. For example, Spain utilizes ~ 50 to 70 million grafted plants per year, accounting for about 40% of the country’s tomato production (Raymond 2013). In contrast, grafted plants have yet to constitute a major portion of the commercial field-based tomato market in the USA, and published field trials with grafted tomatoes in the USA have only appeared in the past decade or so (e.g., Kubota et al. 2008; Louws et al. 2010; Masterson et al. 2016).
Grafted tomatoes typically consist of a traditional cultivar of scion (the top part of the plant that produces the fruit) and a rootstock that may be resistant to one or more soilborne pathogens, and/or “vigorous”—meaning that it drives vegetative and fruit growth at a higher-than-normal rate (Oztekin et al. 2009a, b; Masterson et al. 2016). The vigorous and resistant qualities may both exist in a single rootstock, or a particular rootstock may have one characteristic or the other. The “vigorous” quality is often reported to show the greatest benefit under sub-optimal growing conditions (Albacete et al. 2015b). Tomato scions can also be successfully grafted to rootstocks of other species, including tobacco (Yasinok et al. 2009a), eggplant (Oda et al. 2005), potato (Peres et al. 2005), or various wild Solanum species (e.g., Cortez-Madrigal 2012; Lopes and Mendonça 2016). Many studies have demonstrated that some rootstocks offer other advantages, such as increased tolerance to abiotic stressors including drought (e.g., Cantero-Navarro et al. 2016a), salinity (e.g., Rao et al. 2013; Albacete et al. 2015a), or low temperatures (e.g., Venema et al. 2008a). Many studies have found dramatic, and often economically significant, increases in the marketable yield of tomatoes from grafted plants when compared to the non-grafted scion cultivars (e.g., Barrett et al. 2012a; Djidonou et al. 2013a; Rivard et al. 2010a; Rysin and Louws 2015). However, as with traditional crop cultivar testing, field trials are the best way to determine the performance of a particular scion/rootstock combination in a given production system and locale. This is especially true for grafted plants, because yields may be influenced by how suitable the growing conditions are for growth and tomato production for both the rootstock and scion cultivars.
A global effort to reduce soil fumigant use has been underway since the phase-out of methyl bromide began in the 1990s (Gao et al. 2016; Kyriacou et al. 2017). Tomatoes (processing and fresh market) are a major user of soil fumigants in the world’s major production areas: the USA, Europe, and Asia (Qiao et al. 2010; Deacon et al. 2016; Desaeger et al. 2017; Grieneisen et al. 2017). One of the major benefits of using grafted tomatoes lies in the multi-pathogen resistance of most of the commercially available rootstocks, and the potential for that resistance to reduce the cost and environmental impacts associated with fumigant use in tomatoes (e.g., Rivard and Louws 2008a; Louws et al. 2010; Gilardi et al. 2013; Kokalis-Burelle et al. 2016; Reddy 2016). In some cases, grafting onto pathogen-resistant rootstocks has produced yield increases comparable to fumigation treatments in pathogen-infested experimental systems (e.g., Rivard et al. 2010b; Kokalis-Burelle et al. 2016). Other studies which did not include a fumigation treatment have reported achieving high yields in pathogen-infested fields through the use of grafted tomatoes with rootstocks resistant to the pathogens involved (e.g., López-Pérez et al. 2006; McAvoy et al. 2012a; Rivard et al. 2012a). Since wild plants must grow without the benefit of soil fumigation, many of them possess resistance to soilborne pathogens, and most of the commercial rootstocks used today are hybrids between wild Solanum species (most commonly Solanum habrochaites) and the domesticated tomato, Solanum lycopersicum (Keatinge et al. 2014). Many published studies that did not include experiments with grafted plants have involved the screening of wild tomato species for resistance to common pathogens (e.g., Lopes and Mendonça 2016) and have suggested the use of the resistant species in hybrid rootstock development.
Successful results from some field trials have fueled a perception among many farmers that grafted tomatoes, and other grafted vegetables, tend to produce higher yields of high quality fruit than the non-grafted scion cultivars (e.g., Lee et al. 2010; Rouphael et al. 2010). However, individual trials have found that this is often not the case, but a comprehensive, quantitative review of published data on this issue has not been performed to date. In addition, the effects of grafting on fruit quality remain uncertain (Flores et al. 2010a; Kyriacou et al. 2017). This article reports the results of an extensive review of data from 159 publications which report yields and fruit quality from open-field and/or greenhouse trials of grafted tomatoes including data on 1023 different experimental treatments (where each treatment is a unique combination of scion and rootstock, geographic location, and growing season). We also consider the occurrence of soilborne pathogen resistances among the most popular commercial tomato rootstocks, in light of their potential to reduce the use of soil fumigation.
2 Literature search
A survey of previous grafted tomato field trials was conducted using a very broad query to search the standard bibliographic databases (CAB Abstracts, Biosis, and Web of Science):
Online sources that do not support Boolean queries (e.g., Google Scholar, the Directory of Open Access Journals (DOAJ), and the Chinese National Knowledge Infrastructure (CNKI)) were queried using combinations of these terms. Of the 1113 publications found, studies were rejected if they did not report data from both grafted tomatoes and either the non-grafted or self-grafted scion cultivars in the same experiment. From the 159 studies which did report such information (References for articles included in the meta-analysis), data were recorded on the experimental design, scion and rootstock cultivars, yields obtained and, when available, any of seven measures of fruit quality: pH, titratable acidity, total soluble solids, firmness, lycopene content, vitamin C content, and/or “taste test” data. When yields were reported in units other than kilogram/plant, they were converted to kilogram/plant whenever possible. For example, many articles reported yield data as kilogram/square meter and gave a plant density in units of plants/square meter. In this case, simply dividing the yield by plant density converts the yield data to kilogram/plant. Because methods of assessing pathogen resistance are extremely variable among published studies (e.g., pathogen population determined by various methods or difficult to compare disease symptom assessment schemes), we obtained overall categorization of resistance on a rootstock-by-rootstock basis, from website http://www.vegetablegrafting.org (accessed 26 June 2017).
3 Scale and geographic range of the published literature on grafted tomato trials
Among the 159 published articles which met the criteria of this review, field and greenhouse grafted tomato trials were identified from 126 different locations, mostly in Europe, the Middle East, and Asia, with lesser numbers in North America, South America, and Africa (Fig. 2). This geographic distribution is consistent with global tomato production figures. The FAOSTAT database (http://www.fao.org/faostat/) indicates that the top tomato producers in 2014 were as follows: China (52 million tonnes), India (19 million), the EU (15 million, with 5.6 million from Italy and 4.9 million from Spain), USA (14 million), and Turkey (12 million). With the exception of India, these are the regions from which most of the grafted tomato data have been published to date. The large numbers of studies in Europe and Asia reflect the large role that grafted tomatoes currently play in commercial tomato production on those continents. Many of the studies from the Middle East focused on drought- or salinity-tolerance of specific rootstocks (e.g., Oztekin et al. 2013a; Nilsen et al. 2014a; Albacete et al. 2015a). The study from Iceland was a greenhouse study (Stadler 2013). Many of the studies in Southeast Asia involved the eggplant rootstock EG203 developed by the World Vegetable Center (formerly AVRDC, Taiwan), which is flood-tolerant, an important trait to have in the monsoon regions (e.g., Palada and Wu 2007a). Regardless of the geographic localities, almost all trials evaluated fresh market tomato cultivars as scions.
4 Comparing the data across all published grafted tomato trials
4.1 Yield data expressed as kilogram fruit/plant
Of the 1023 experimental treatments in the literature survey, 735 expressed yields either as kilogram fruit/plant, or in units that could be converted to kilogram/plant using information provided in the article. Figure 3 shows this “kg fruit/plant” data as the yield from the non- or self-grafted scion cultivar versus the yield from the scion cultivar grafted onto a different rootstock. Points located above the 1:1 line are cases where the grafted plants gave a higher yield than the non- or self-grafted plants. Many of the experimental treatments which produced very low yields (< 2 kg/plant) involved intentionally stressed plants, such as those grown under low-water or high-saline conditions. About 80% of the data in Fig. 3 represents marketable yield, while the remaining 20% represents total yield data from studies that did not differentiate between marketable and cull fruit.
Yield performance of grafted versus non- or self-grafted tomato plants. Data from 735 experimental treatments where: (1) grafted plants with different rootstock and scion varieties were compared directly to either the non- or self-grafted scion variety in the same experiment; and (2) enough information was provided to express yields as kilogram fruit/plant. Published data for individual experimental treatments (blue), average of all data (yellow, at point 3.35, 4.01).
Overall, there were more instances of grafted tomatoes producing higher yields relative to the non- or self-grafted plants, than there were instances of grafted tomatoes producing lower yields. The average of all the data is the yellow point at (3.35, 4.01), which indicates an average yield increase for grafted plants across all of the studies of 0.66 kg/plant. However, it is notable that a large number of points are located below, on, or only slightly above the 1:1 line—indicating that the grafted plants did not provide substantial yield increases relative to the non- or self-grafted plants in a large number of cases. This point is further examined in the next section by a statistical analysis of yield data across the studies.
4.2 Grafting often does not produce higher yields
Overall, 684 data points represented grafted plant yield data which included a statistical analysis to separate significant differences between grafted and either non- or self-grafted plants (Table 1). Self-grafted plants differed from non-grafted plants in only 1 of the 53 cases with statistical data, suggesting that including self-grafted plants in experimental studies is probably not necessary. The heterografted plants (i.e., where rootstock and scion are of different cultivars) produced significantly higher yields in only about one third (35%) of the cases, with no significant difference in 58% of cases, and the non- or self-grafted plants gave higher yields in the remaining 6% of cases. As in the self-grafted data, the ratio range for the “Heterografted (all data),” varied widely among individual experimental treatments, from 0.09 to 5.70. This wide range probably reflects the broad spectrum of tomato cultivars used (see Table 2), growing conditions that may not have been equally suitable to the scion and rootstock, and plant stress levels (e.g., pathogen pressure or salinity) among the studies. However, it does underscore the fact that grafting is not a magic bullet, since the majority of rootstock/scion combinations do not produce significant yield increases in the production systems tested. We wondered whether the high heterograft ratios observed in some cases are linked to specific rootstock/scion combinations, and next consider the extensive data available for the rootstock cultivar ‘Maxifort.’
4.3 The yield performance of a given rootstock (e.g., cv. ‘Maxifort’) is highly variable depending on geography and/or scion variety
Our literature survey included data on 202 different rootstocks (Table 2). The cultivar ‘Maxifort’ was by far the most commonly tested rootstock, represented by 105 of the 1023 experimental treatments (more than 10%) in the survey. Most of the other rootstocks appeared in only a few trials. ‘Maxifort’ is reported to be resistant to seven different diseases: corky root rot, Fusarium wilt races 1 & 2 (but not race 3), Fusarium crown and root rot, Verticillium wilt, nematodes, and tomato mosaic virus (http://www.vegetablegrafting.org/tomato-rootstock-table/). Therefore, ‘Maxifort’ is a good candidate for use in pathogen-infested fields, or even portions of fields, that cannot be fumigated due to regulatory restrictions (Fennimore and Goodhue 2016; Porter 2017).
4.3.1 Grafted/non-grafted yield ratios for a given rootstock vary with scion variety
The survey found 43 different scion cultivars that were tested with ‘Maxifort’ as a rootstock (Fig. 4). Across all of these scions, the average ratio of the yield from ‘Maxifort’-grafted plants to the yield from non-grafted plants in the same experiment was 1.29 ± 0.44 (mean ± standard deviation). If the yield ratios are averaged for each scion in the survey, it appears that some scions performed much better than others with ‘Maxifort,’ in the production systems in which they were tested. For example, in 17 different trials with cv. ‘BHN 589’ on ’Maxifort,’ the average yield ratio was 1.51 ± 0.34, whereas cv. ‘Mountain Fresh’ averaged only 0.70 ± 0.30 in four trials. However, the economic viability of most of these grafted combinations appears questionable since relatively few of the tested scion cultivars have produced substantial average yield increases, i.e., ratios substantially above 1.00.
Performance of grafted tomato plants with rootstock ‘Maxifort’ in published field trials. Yield ratio is the ratio of the yield obtained with Maxifort rootstock to the yield obtained with the non- or self-grafted plants of the scion cultivar. The numbers of distinct treatments (rootstock/scion combination, location, and growing season) are indicated in parentheses in the Y-axis labels. Black bar, average of all 105 data points, the ratio is 1.29 ± 0.44 (mean ± standard deviation).
4.3.2 Grafted/non-grafted yield ratios for a given rootstock vary with geography and scion cultivar
Published grafted tomato trials often used locally important cultivars as the scions, in order to determine the performance of grafted combinations relative to cultivars with which local growers are familiar. Mapping the geographic locations of the studies including ‘Maxifort’ shows that most of the data (93 of 105 data points from 34 of 37 different localities) was generated in Europe or the USA, with data from single locations in Turkey, Japan, and Brazil (Fig. 5). Data on ‘Maxifort’ were lacking from the major tomato producing countries of China and India. Trials of ‘Maxifort’ in the USA had a higher yield ratio mean than those in Europe (1.380 vs. 1.182) and a larger proportion of yield ratios above 1.25 (29/57 vs. 6/36). However, the means were not completely separated with either the standard 95% Confidence Interval (CI) or the more generous 90% CI (Table 3). These geographic differences could have arisen from differences in either growing conditions (e.g., climate, soil) or production system decisions (e.g., irrigation practices, number of harvests, whether fruit are picked green or red, etc.). Assigning the ‘Maxifort’/scion combination data to the corresponding geographic localities revealed that no individual scion was used in trials in both Europe and the USA (Table 4). Therefore, it was not possible to separate the potential effects of scion cultivar from those of differences in growing conditions or production systems between trials conducted in Europe and those in the USA.
Locations of all trials that included Maxifort as rootstock. The average yield increases obtained in the USA were consistently higher than those obtained in the European trials; however, completely different sets of scion varieties were used in the USA and Europe. Therefore, it remains uncertain whether differences in biology (scion variety) or geography (climate, soil, production practices) were responsible for the differences in yield increases in these two regions. It is not possible to assess Maxifort’s potential performance outside of USA and Europe due to insufficient data
4.4 Yields obtained with self-grafted versus non-grafted plants rarely differ
Many published studies include self-grafted plants, in which the scion cultivar is cut and grafted onto roots of the same cultivar. The purpose of these treatments is to determine whether there is an effect of the grafting process itself, on yield or fruit quality, which is independent of any specific combinations of different scions and rootstocks. Of the 54 published cases in our survey that included both self-grafted and non-grafted scion plants, the average ratio of yields between the two was 0.999, in contrast to the ratio of 1.37 for all 949 cases of heterografted plants (Table 1). Among self-grafted trial results, significant differences occurred in only four of the 53 cases that included statistical data. While this may suggest that including self-grafted plants is not necessary, the range of yield ratios from individual studies spans roughly an order of magnitude, from 0.23 to 2.04. This range indicates that the differences in yields from self- and non-grafted plants of the same variety were occasionally quite dramatic.
4.5 Soilborne pathogen resistance of commercial tomato rootstocks for potential reduction of pesticide use
Numerous studies have examined various aspects of the pathogen-resistance of different tomato rootstocks (e.g., Rivard and Louws 2008a; Louws et al. 2010; Barrett et al. 2012a; McAvoy et al. 2012a; Rivard et al. 2012a; Gilardi et al. 2013; Keatinge et al. 2014; Reddy 2016). Published pathogen resistance studies vary in terms of rootstock species/cultivars, pathogens (or pathogen strains) involved, methods of determining “resistance” or “susceptibility,” and the degrees of resistance achieved under a wide variety of growing conditions. This variability makes comparisons across the studies very difficult. However, a table of commercially available tomato rootstocks lists their pathogen resistances as either HR (highly resistant), R (resistant) or IR (intermediate or partial resistance), based on information obtained from seed company catalogs and websites (http://www.vegetablegrafting.org/tomato-rootstock-table/, accessed 16 February 2017).
While over 80% of the rootstocks are listed as resistant to Fusarium wilt races 1 & 2, none are resistant to Southern blight (Table 5). Seventeen of the 51 (33%) individual rootstocks are resistant or highly resistant to seven or more common soilborne pathogens (Table 6). The results from Table 5 show that for many pathogens, dozens of different rootstocks show some degree of resistance to common pathogens, many of which are categorized as “highly resistant.” This flexibility allows researchers and growers the opportunity to find resistant rootstocks which work well with local scion cultivars and in a given local production system.
Resistance to soilborne pathogens is often suggested as a method for reducing the need to use pathogen-controlling pesticides. Soil fumigants pose significant environmental risks and are heavily used to control soilborne pathogens in the world’s major tomato production regions (e.g., China: Qiao et al. 2010; Europe: Deacon et al. 2016; and the USA: Desaeger et al. 2017). In the limited number of studies that directly compared grafted plants to fumigation treatments in pathogen-infested fields, the grafted plants often gave higher yields than the fumigation treatments (e.g., Rivard et al. 2010b; Kokalis-Burelle et al. 2016). The results from pathogen-infested field trials that did not include fumigant treatments (e.g., Rivard et al. 2010b; McAvoy et al. 2012a; Rivard et al. 2012a; Kokalis-Burelle et al. 2016) show that the resistances are often strong enough to achieve high yields in pathogen-infested fields, and may allow the reduction of soil fumigant use.
4.6 Effects of grafting on fruit quality is usually minor, but occasionally dramatic
While the results of selected individual studies on the effect of grafting on tomato fruit quality have been qualitatively reviewed (e.g., Kyriacou et al. 2017), a quantitative analysis of published data indicates that grafting rarely has a significant effect on several commonly reported measures of fruit quality (Table 7). The row labels are the same as in Table 1, though the word “grafted” is replaced by the letter “G” in some cases, due to the width of this table. Because pH is the only parameter that was always expressed in the same units, the difference between measurements from grafted and non-grafted plants could be considered across the studies; while for all other parameters, ratios are used to normalize the data across studies. For each parameter, the percentage of cases with a significant difference between grafted and non-grafted values was modest (14–33%), the average ratios were all close to 1 (0.94–1.05), and the average difference between pH measurements was close to zero (Table 7). However, the ranges of ratios and pH differences are rather broad, indicating that substantial differences in fruit quality values between tomatoes from grafted and non-grafted plants do occur in certain cases. Unfortunately, the data are too dispersed across various rootstocks and scion cultivars to determine whether certain combinations, which typically only have one or two observations, are consistently responsible for the occasional large differences in quality measures.
5 Conclusions
5.1 An integrated, quantitative analysis of available original grafted tomato trial data reveals general patterns relevant to the use of grafted plants in global tomato production
Traditional review articles typically present data from a few dozen articles in a qualitative, so-called vote-counting fashion, and base conclusions on the number of cases where a given treatment “worked” versus the number where it “did not work.” Previous reviews using such approaches have concluded that grafting does increase the yields of tomatoes and other fruiting vegetables, may or may not affect fruit quality, and have fueled confidence in the pathogen resistance of many rootstocks. Our approach quantitatively integrated the published data on yield and fruit quality from available field trials conducted around the world in order to validate the notions of increased yields and potential effects on fruit quality. In addition, we explore the potential for decreasing pesticide use by incorporating grafted tomatoes that include commercially available pathogen-resistant rootstocks into production systems.
5.2 Rootstock performance can vary greatly depending on the scion variety it is paired with, though scion choices vary geographically
The perception that rootstocks magically work to drive higher yields with any scion is an oversimplification. For 654 yield data points expressed as kilogram/plant, yields from grafted and non-grafted plants were significantly different in only 35% of the cases. Data from 105 different trial experimental treatments that used ‘Maxifort’ as a rootstock collectively paired it with 43 different scions; though completely different sets of scions were used in the US and European trials. Thus, it is not possible to separate the effects of scion cultivar and geography in this dataset. As a result, as with traditional crop cultivar testing, it seems that the only way to know how well a specific rootstock/scion combination will perform in a given production system is through field trials.
5.3 Self-grafted plants rarely have significantly different yields than non-grafted plants.
The data from 53 published trials argue against the need to include self-grafted plants in experimental trials, since there are rarely significant differences in yields between non-grafted and self-grafted tomatoes.
5.4 Grafted plants usually produce fruit of similar quality to fruit from non-grafted plants.
Fruit quality data based on human tasters were rare among the published studies. In the majority of cases where chemical analyses of quality parameters were presented, measures of fruit quality seldom varied significantly between grafted and non-grafted plants of the scion cultivar. However, for each measure there were individual cases where quality parameters were dramatically different. Due to limited sample sizes for specific rootstock/scion combinations, it was not possible to statistically analyze whether specific combinations were the cause of the occasionally substantial fruit quality differences.
5.5 Potential soil fumigant use reduction by virtue of rootstock pathogen resistance
Resistance to multiple soilborne pathogens is widespread among commercial tomato rootstocks available for grafting. One third of them are said to be “resistant” or “highly resistant” to seven or more common pathogens, and grafted tomato trials with selected rootstocks in pathogen-infested fields typically find that the grafted plants can maintain high yields in the presence of high pathogen pressure. Thus, they offer the potential for reduction of soil pesticide use. However, only a limited number of studies have compared grafted plants to soil fumigation treatments within the same experiment; and more grafted tomato trials should incorporate fumigation treatments as a variable in the experimental design.
References
Albacete A, Andujar C, Dodd I, Giuffrida F, Hichri I, Lutts S, Thompson A, Asins M (2015a) Rootstock-mediated variation in tomato vegetative growth under drought, salinity and soil impedance stress. Acta Hortic 1086:141–146. https://doi.org/10.17660/ActaHortic.2015.1086.17
Albacete A, Martinez-Andujar C, Martinez-Perez A, Thompson AJ, Dodd IC, Perez-Alfocea F (2015b) Unravelling rootstock X scion interactions to improve food security. J Exp Biol 66:2211–2226. https://doi.org/10.1093/jxb/erv027
Barrett CE, Zhao X, Hodges AW (2012a) Cost benefit analysis of using grafted transplants for root-knot nematode management in organic heirloom tomato production. HortTechnology 22:252–257
Cantero-Navarro E, Romero-Aranda R, Fernandez-Munoz R, Martinez-Andujar C, Perez-Alfocea F, Albacete A (2016a) Improving agronomic water use efficiency in tomato by rootstock-mediated hormonal regulation of leaf biomass. Plant Sci 251(Sp Iss):90–100. https://doi.org/10.1016/j.plantsci.2016.03.001
Cortez-Madrigal H (2012) Grafts of crops on wild relatives as base of an integrated pest management: The tomato Solanum lycopersicum as example. In: Larramendy ML, Soloneski S (eds) Integrated pest management and pest control: current and future tactics. InTech, Rijeka, pp 127–146. https://doi.org/10.5772/30181
Deacon S, Alix A, Knowles S, Wheeler J, Tescari E, Alvarez L, Nicolette J, Rockel M, Burston P, Quadri G (2016) Integrating ecosystem services into crop protection and pest management: Case study with the soil fumigant 1,3-dichloropropene and its use in tomato production in Italy. Integr Environ Assess Manag 12:801–810. https://doi.org/10.1002/ieam.1761
Desaeger J, Dickson DW, Lacascio SJ (2017) Methyl bromide alternatives for control of root-knot nematode (Meloidogyne spp.) in tomato production in Florida. J Nematol 49:140–149
Djidonou D, Gao Z, Zhao X (2013a) Economic analysis of grafted tomato production in sandy soils in northern Florida. HortTechnology 23:613–621
Fennimore SA, Goodhue RE (2016) Soil disinfestation with steam: a review of economics, engineering, and soil pest control in California strawberry. Int J Fruit Sci 16(S1):71–83. https://doi.org/10.1080/15538362.2016.1195312
Flores FB, Sanchez-Bel P, Estañ MT, Martinez-Rodriguez MM, Moyano E, Morales B, Campos JF, Garcia-Abellán JO, Egea MI, Fernández-Garcia N, Fomojaro F, Bolarín MC (2010a) The effectiveness of grafting to improve tomato fruit quality. Sci Hortic 125:211–217. https://doi.org/10.1016/j.scienta.2010.03.026
Gao SD, Sosnoskie LM, Cabrera JA, Qin RJ, Hanson BD, Gerik JS, Wang D, Browne GT, Thomas JE (2016) Fumigation efficacy and emission reduction using low-permeability film in orchard soil fumigation. Pest Manage Sci 72:306–314. https://doi.org/10.1002/ps.3993
Gilardi G, Gullino ML, Garibaldi A (2013) Critical aspects of grafting as a possible strategy to manage soil-borne pathogens. Sci Hortic 149:19–21. https://doi.org/10.1016/j.scienta.2012.07.014
Grieneisen ML, Zhan Y, Chen JH, Zhang MH (2017) Alternative management practices to reduce soil fumigant use in key California crops: a meta-analysis approach. Presentation: Department of Pesticide Regulation Integrated Pest Management Symposium, 21 Mar 2017, Sacramento, CA. http://www.cdpr.ca.gov/docs/pestmgt/ipm_symposium/mike_grieneisen.pdf. Accessed 26.06.2017.
Johnson SJ, Miles CA (2011) Effect of healing chamber design on the survival of grafted eggplant, tomato, and watermelon. HortTechnology 21:752–758
Keatinge JDH, Lin LJ, Ebert AW, Chen WY, Hughes JA, Luther GC, Wang JF, Ravishankar M (2014) Overcoming biotic and abiotic stress in the Solanaceae through grafting: current status and future perspectives. Biol Agric Hortic 30:272–287. https://doi.org/10.1080/01448765.2014.964317
Kokalis-Burelle N, Butler DM, Hong JC, Bausher MG, McCollum G, Rosskopf EN (2016) Grafting and Paladin Pic-21 for nematode and weed management in vegetable production. J Nematol 48:231–240
Kubota C, McClure MA, Kokalis-Burelle N, Bausher MG, Rosskopf EN (2008) Vegetable grafting: history, use, and current technology status in North America. HortScience 43:1664–1669
Kyriacou MC, Rouphael Y, Colla G, Zrenner R, Schwarz D (2017) Vegetable grafting: the implications of growing agronomic imperative for vegetable fruit quality and nutritive value. Front Plant Sci 8:741. https://doi.org/10.3389/fpls.2017.00741
Lee JM, Kubota C, Tsao SJ, Bie Z, Hoyos Echevarria P, Morra L, Oda M (2010) Current status of vegetable grafting: diffusion, grafting techniques, automation. Sci Hortic 127:93–105. https://doi.org/10.1016/j.scienta.2010.08.003
Leonardi C, Giuffrida F (2006) Variation of plant growth and macronutrient uptake in grafted tomatoes and eggplants on three different rootstocks. Eur J Hortic Sci 71:97–101
Lopes CA, Mendonça JL (2016) Reação de acessos de jurubeba à murcha bacteriana para uso como porta-enxerto em tomateiro—Reaction of accessions of two species of “jurubeba” as rootstocks to protect tomato plants against bacterial wilt. Hortic Bras 34:356–360. https://doi.org/10.1590/S0102-05362016003008
López-Pérez JA, Le Strange M, Kaloshian I, Ploeg AT (2006) Differential response of Mi gene-resistant tomato rootstocks to root-knot nematodes (Meloidogyne incognita). Crop Prot 25:382–388. https://doi.org/10.1016/j.cropro.2005.07.001
Louws FJ, Rivard CL, Kubota C (2010) Grafting fruiting vegetables to manage soilborne pathogens, foliar pathogens, arthropods and weeds. Sci Hortic 127:127–146. https://doi.org/10.1016/j.scienta.2010.09.023
Masterson SA, Kennelly MM, Janke RR, Rivard CL (2016) Scion shoot removal and rootstock cultivar affect vigor and early yield of grafted tomatoes grown in high tunnels in the central United States. HortTechnology 26:399–408
McAvoy T, Freeman JH, Rideout SL, Olson SM, Paret ML (2012a) Evaluation of grafting using hybrid rootstocks for management of bacterial wilt in field tomato production. HortScience 47:621–625
Nilsen ET, Freeman J, Grene R, Tokuhisa J (2014a) A rootstock provides water conservation for a grafted commercial tomato (Solanum lycopersicum L.) line in response to mild-drought conditions: a focus on vegetative growth and photosynthetic parameters. PLoS One 9:e115380. https://doi.org/10.1371/journal.pone.0115380
Oda M, Maruyama M, Mori G (2005) Water transfer at graft union of tomato plants grafted onto Solanum rootstocks. J Jpn Soc Hortic Sci 74:458–463. https://doi.org/10.2503/jjshs.74.458
Oztekin GB, Giuffrida F, Tuzel Y, Leonardi C (2009a) Is the vigour of grafted tomato plants related to root characteristics? J Food Agric Environ 7:364–368
Oztekin GB, Tuzel Y, Tuzel IH (2013a) Does mycorrhiza improve salinity tolerance in grafted plants? Sci Hortic 149:55–60. https://doi.org/10.1016/j.scienta.2012.02.033
Palada MC, Wu DL (2007a) Increasing off-season tomato production using grafting technology for peri-urban agriculture in Southeast Asia. Acta Hortic 742:125–131. https://doi.org/10.17660/ActaHortic.2007.742.17
Peres LEP, Carvalho RF, Zsogon A, Bermudez-Zambrano OD, Robles WGR, Tavares S (2005) Grafting of tomato mutants onto potato rootstocks: an approach to study leaf-derived signaling on tuberization. Plant Sci 169:680–688. https://doi.org/10.1016/j.plantsci.2005.05.017
Porter IJ (2017) What is driving industry tipping points from open field to hydroponics. Acta Hortic 1176:129–136. https://doi.org/10.17660/ActaHortic.2017.1176.17
Qiao K, Jiang L, Wang H, Ji X, Wang K (2010) Evaluation of 1,3-dichloropropene as a methyl bromide alternative in tomato crops in China. J Agric Food Chem 58:11395–11399. https://doi.org/10.1021/jf102830y
Rao ES, Kadirvel P, Symonds RC, Ebert AW (2013) Relationship between survival and yield related traits in Solanum pimpinellifolium under salt stress. Euphytica 190:215–228. https://doi.org/10.1007/s10681-012-0801-2
Raymond G (2013) Grafting market developments. Rijk Zwaan USA, Salinas, CA, 26 pp http://www.vegetablegrafting.org/wp/wp-content/uploads/2013/11/session-4-raymond-scri-vege-grftg-symp-nov13.pdf. Accessed 26.06.2017
Reddy PP (2016) Grafted vegetables for management of solborne pathogens. In: Reddy PP (ed) Sustainable crop protection under protected cultivation. Springer, Berlin, pp 83–97. https://doi.org/10.1007/978-981-287-952-3_7
Rivard CL, Louws FJ (2008a) Grafting to manage soilborne diseases in heirloom tomato production. HortScience 43:2104–2111
Rivard CL, Sydorovych O, O’Connell S, Peet MM, Louws FJ (2010a) An economic analysis of two grafted tomato transplant production systems in the United States. HortTechnology 20:794–803
Rivard CL, O’Connell S, Peet MM, Welker RM, Louws FJ (2012a) Grafting tomato to manage bacterial wilt caused by Ralstonia solanacearum in the southeastern United States. Plant Dis 96:973–978. https://doi.org/10.1094/PDIS-12-10-0877
Rouphael Y, Schwarz D, Krumbein A, Colla G (2010) Impact of grafting on product quality of fruit vegetables. Sci Hortic 127:172–179. https://doi.org/10.1016/j.scienta.2010.09.001
Rysin O, Louws FJ (2015) Decision tool for growers to evaluate economic impact of grafting technology adoption: an application to open-field conventional tomato production. HortTechnology 25:132–138
Stadler C (2013) Final report of the research project „Áhrif ljósstyrks, ágræðslu og umhverfis á vöxt, uppskeru og gæði gróðurhúsatómata“ [Reykir, Iceland]. Rit LbhÍ nr. 45, v, 58 pp. http://www.lbhi.is/sites/default/files/gogn/vidhengi/finalreporttomato.pdf. Accessed 27 Jun 2017
Tian S, Ashraf MA, Kondo N, Shiigi T, Momin MA (2013) Optimization of machine vision for tomato grafting robot. Sensor Lett 11:1190–1194. https://doi.org/10.1166/sl.2013.2899
Venema JH, Dijk BE, Bax JM, van Hasselt PR, Elzenga JTM (2008a) Grafting tomato (Solanum lycopersicum) onto the rootstock of a high-altitude accession of Solanum habrochaites improves suboptimal-temperature tolerance. Environ Exp Bot 63:359–367. https://doi.org/10.1016/j.envexpbot.2007.12.015
Yasinok AE, Sahin FI, Eyidogan F, Kuru M, Haberal M (2009a) Grafting tomato plant on tobacco plant and its effect on tomato plant yield and nicotine content. J Sci Food Agric 89:1122–1128. https://doi.org/10.1002/jsfa.3555
References for articles included in the meta-analysis
Abdelmageed AHA, Gruda N (2009) Influence of grafting on growth, development and some physiological parameters of tomatoes under controlled heat stress conditions. Eur J Hortic Sci 74:16–20
Alvarez-Hernandez JC (2012) Comportamiento agronomico e incidencia de enfermedades en plantas de tomate (Solanum lycopersicum L.) injertadas = Agronomic performance and incidence of diseases in tomato grafted plants (Solanum lycopersicum L.). Acta Agron. Univ Nac Colombia 61:117–125
Arwiyanto T, Nurcahyanti SD, Indradewa D, Widada J (2015) Grafting local commercial tomato cultivars with H-7996 and Eg-203 to suppress bacterial wilt (Ralstonia solanacearum) in Indonesia. Acta Hortic 1069:173–178
Baez-Valdez EP, Carrillo-Fasio JA, Baez-Sanudo MA, Garcia-Estrada RS, Valdez-Torres JB, Contreras-Martinez R (2010) Resistant rootstocks utilization for fusarium control (Fusarium oxysporum f. sp. lycopersici Snyder & Hansen race 3) in tomato (Lycopersicon esculentum Mill) under shade conditions. Rev Mex Fitopatol 28:111–123
Bai X (2009) The effect of graft cultivation on yield and economic benefit of tomato. Guizhou Agric Sci 37:131–132
Barrett CE, Zhao X, McSorley R (2012b) Grafting for root-knot nematode control and yield improvement in organic heirloom tomato production. HortScience 47:614–620
Barrett CE, Zhao X, Sims CA, Brecht JK, Dreyer EQ, Gao ZF (2012c) Fruit composition and sensory attributes of organic heirloom tomatoes as affected by grafting. HortTechnology 22:804–809
Bhatt RM, Upreti KK, Divya MH, Bhat S, Pavithra CB, Sadashiva AT (2015) Interspecific grafting to enhance physiological resilience to flooding stress in tomato (Solanum lycopersicum L.). Sci Hortic 182:8–17
Bogescu M, Doltu M, Sora D, Iordache B (2012) Sustainable alternatives to methyl bromide in Romanian horticulture. Bull Univ Agric Sci Vet Med Cluj Napoca Horticulture 69:89–95
Bogoescu M, Doltu M, Iordache B, Vintila M, Sora D, Mohora A (2011) The grafting tomatoes crop—an alternative for vegetable growers. Bull Univ Agric Sci Vet Med Cluj Napoca Horticulture 68:215–221
Bogoescu M, Gullino ML, Minuto A, Amadio A (2005) Alternatives to methyl bromide in Romanian protected crops. Acta Hortic 698:315–320
Bonomo G, Maltese V, Bellafiore M (2008) A Marsala si prova pomodoro datterino = Datterino tomato is also tested in Marsala. Colture Protette 37(8):54–58
Branco RBF, Goto R, Carneiro Junior AG, Guimaraes VF, Rodrigues JD, Trivelin PCO (2007a) Transporte do 15N e produtividade do tomateiro enxertado irrigado com agua carbonatada = Transport of 15N and yield of the grafted tomato irrigated with carbonated water. Hortic Bras 25:77–81
Branco RBF, Goto R, Carneiro Junior AG, Guimaraes VF, Rodrigues JD, Trivelin PCO, Silveira LVDA (2007b) Enxertia e água de irrigação carbonatada no transporte de 15N e na produção do tomateiro = Grafting and carbonated irrigation water in transport of 15N and in the tomato production. Reva Bras Engenhar Agricola e Ambient 11:374–379
Burleigh JR, Black LL, Mateo LG, Cacho D, Aganon CP, Boncato T, Arida IA, Ulrichs C, Ledesma DR (2005) Performance of grafted tomato in Central Luzon, Philippines: a case study on the introduction of a new technology among resource-limited farmers. Crop Manage 2005(Jul):1–9
Cantero-Navarro E, Romero-Aranda R, Fernandez-Munoz R, Martinez-Andujar C, Perez-Alfocea F, Albacete A (2016b) Improving agronomic water use efficiency in tomato by rootstock-mediated hormonal regulation of leaf biomass. Plant Sci 251:90–100
Cantu RR, Junglaus RW, Goto R (2009) Produtividade e crescimento do tomateiro ‘Paron’ enxertado em diferentes porta-enxertos = Production and growth of tomato Paron cultivar grafted in different rootstocks. Agropec Catarin 22:69–73
Cardoso SC, Soares ACF, Brito ADS, Carvalho LAD, Ledo CADS (2006a) Viabilidade de uso do hibrido Hawaii 7996 como porta-enxerto de cultivares comerciais de tomate = Potential of Hawaii 7996 hybrid as rootstock for tomato cultivars. Bragantia 65:89–96
Cardoso SC, Soares ACF, Brito ADS, Carvalho LAD, Peixoto CC, Pereira MEC, Goes E (2006b) Qualidade de frutos de tomateiro com e sem enxertia = Quality characteristics of tomato fruits from grafted plants. Bragantia 65:269–274
Chalanska A, Slusarski C, Ulinski Z, Meszka B, Sobiczewski P, Malusa E, Ciesielska J (2014) The effectiveness of grafting and soil fumigation on the performance of greenhouse tomatoes. Acta Hortic 1044:263–268
Chaudhari S (2015) Critical period for weed control in grafted tomato (Solanum lycopersicum) and herbicide tolerance of grafted tomato and eggplant (Solanum melongena). Dissertation, North Carolina State University
Chaudhari S, Jennings K, Monks D, Jordan D, Gunter C, Louws F (2015) Response of grafted tomato (Solanum lycopersicum) to herbicides. Weed Technol 29:800–809
Chen Z, Wang P, Zhou Y, Ji Y, Liang P, Wan Z, Hao J (2012) Effects of different resistant rootstocks on yield and quality of grafted tomato and control effects of Meloidogyne incognita. China Veg 2012(20):83–87
Deng L, Zhao L, Liu L (2007) Effects of different rootstocks with resistance to root-knot nematode on growth, quality and yield of tomato in greenhouse. China Veg 2007:13–16
Djidonou D (2012) Improving fruit yield and nutrient management in tomato production by using grafting. Dissertation, University of Florida
Djidonou D, Gao ZF, Xhao X (2013b) Economic analysis of grafted tomato production in sandy soils in northern Florida. HortTechnology 23:613–621
Dong D, Cao Z, Wang X, Hu J, Gullino ML (2007) Effect of nematode resistant rootstocks on growth characteristics and yields of tomato. Acta Hortic Sin 34:1305–1308
Dong LD, Shi L, Guo JH (2010) Growth and heat-resistance of grafted tomato under high temperature stress. J Agric Univ Hebei 33:27–29, 49
Ekpong B, Somkul C (2007) Influence of different rootstocks for grafting on yield and quality of currant tomato (Lycopersicon pimpinellifolium Jusl). In: Proceedings of the 45th Kasetsart University Annual Conference, Bangkok, Thailand, 30 January-2 February 2007, pp 586-592
Espinoza Arellano JJ, Chew Madinaveitia YI, Mascorro AG (2012) Evaluación económica del uso de injerto en tomate (Lycopersicon esculentum) bajo condiciones de invernadero. Produc Agrícola Agrofaz 12:57–62
Estevez-Caparros JM, Diaz-Perez M, Camacho-Ferre F (2011) Influence of several rootstocks on yield of cultivars of pear cherry tomato cultivated under mesh greenhouse. J Food Agric Environ 9:364–368
Farias EADP, Ferreirza RLF, Araujo Neto SED, Costa FC, Nascimento DS (2013) Organic production of tomatoes in the Amazon region by plants grafted on wild Solanum rootstocks. Ciênc Agrotecnol 37:323–329
Flomo ST (2010) Investigation of yield and quality of grafted heirloom and hybrid tomatoes. Dissertation, Western Kentucky University
Flores FB, Sanchez-Bel P, Estan MT, Martinez-Rodriguez MM, Moyano E, Morales B, Campos JF, Garcia-Abellan JO, Egea MI, Fernandez-Garcia N, Romojaro F, Bolarin MC (2010b) The effectiveness of grafting to improve tomato fruit quality. Sci Hortic 125:211–217
Freeman J, McAvoy T, Rideout S, Paret M, Olson S (2011) Utilization of grafted tomato seedlings for bacterial wilt resistance in open field production. Acta Hortic 914:337–339
Gajc-Wolska J, Kowalczyk K, Marcinkowska M, Radzanowska J, Bujalski D (2014) Influence of growth conditions and grafting on the yield, chemical composition and sensory quality of tomato fruit in greenhouse cultivation. J Elementol 20:73–81
Gajc-Wolska J, Lyszkowska M, Zielony T (2010) The influence of grafting and biostimulators on the yield and fruit quality of greenhouse tomato cv. (Lycopersicon esculentum Mill.) grown in the field. Veg Crops Res Bull 72:63–70
Gebologlu N, Ylmaz E, Cakmak P, Aydn M, Kasap Y (2011) Determining of the yield, quality and nutrient content of tomatoes grafted on different rootstocks in soilless culture. Sci Res Essays 6:2147–2153
Ghosheh H, Al-Kawamleh M, Makhadmeh I (2010) Weed competitiveness and herbicidal sensitivity of grafted tomatoes (Solanum lycopersicon Mill.). J Plant Prot Res 50:307–313
Gioia FD, Serio F, Buttaro D, Ayala O, Santamaria P (2010) Influence of rootstock on vegetative growth, fruit yield and quality in ‘Cuore di Bue’, an heirloom tomato. J Hortic Sci Biotechnol 85:477–482
Giotis C, Theodoropoulou A, Cooper J, Hodgson R, Shotton P, Shiel R, Eyre M, Wilcockson S, Markellou E, Liopa-Tsakalidis A, Volakakis N, Leifert C (2012) Effect of variety choice, resistant rootstocks and chitin soil amendments on soil-borne diseases in soil-based, protected tomato production systems. Eur J Plant Pathol 134:605–617
Godoy Hernandez H, Castellanos Ramos JZ, Alcantar Gonzalez G, Sandoval Villa M, Munoz Ramos JDJ (2009) Efecto del injerto y nutricion de tomate sobre rendimiento, materia seca y extraccion de nutrimentos = Greenhouse tomato yield, dry matter and nutrient accumulation, as affected by grafting and nutrient supply. Terra Latinoam 27:1–11
Gomes RF, Cruz FJR, Nunes RC, Castoldi R, Santos DMM, Braz LT (2016) Respostas enzimaticas na enxertia de tomateiro. Hortic Bras 34:491–497
Gomes RF (2013) Enxertia, atividade enzimática e orientaçãdo tomateiro com quatro hastes. Dissertation, Universidad Estadual Paulista, Campus de Jaboticabal
Goto R, Miguel AD, Marsal JI, Gorbe E, Calatayud A (2013) Effect of different rootstocks on growth, chlorophyll a fluorescence and mineral composition of two grafted scions of tomato. J Plant Nutr 36:825–835
Goto R, Sirtori LF, Rodrigues JD, Lopes MC (2010) Produção de tomateiro, híbrido momotaro, em função do estádio das mudas e da enxertia = Production of tomato, momotaro hibrio according to seedling stage and grafting. Ciênc Agrotec 34:961–966
Granges A, Gillioz JM, Augsburger J, Nicollier F (2008) Varietes de tomate a grappes cultivees hors sol a basse temperature: valeur agronomique, analytique et gustative = Soilless truss tomato varieties cultivated under low temperature conditions: agronomic, analytical and sensory values. Rev Suisse Viticu, Arboric Hortic 40:223–229
Guo J (2015) Effects of different grafting rootstocks on the growth, quality and yield of tomato. J Hebei Agric Sci 19:22–25, 64
Gur A, Semel Y, Osorio S, Friedmann M, Seekh S, Ghareeb B, Mohammad A, Pleban T, Gera G, Fernie AR, Zamir D (2011) Yield quantitative trait loci from wild tomato are predominately expressed by the shoot. Theor Appl Genet 122:405–420
Haberal M, Korpe DA, Iseri OD, Sahin FI (2016) Grafting tomato onto tobacco rootstocks is a practical and feasible application for higher growth and leafing in different tobacco-tomato unions. Biol Agric Hortic 32:248–257
Hibar K, Daami-Remadi M, Jabnoun-Khiareddine H, El Mahjoub M (2006) Control of Fusarium crown and root rot of tomato caused by Fusarium oxysporum f.sp. radicis-lycopersici, by grafting onto resistant rootstocks. Plant Pathol J 5:161–165
Higashide T, Nakano A, Yasuba K (2014) Yield and dry matter production of a Japanese tomato ‘Momotaro York’ are improved by grafting onto a Dutch rootstock ‘Maxifort. J Jpn Soc Hortic Sci 83:235–243
Hoyos Echevarria P, Rollon Martinez G, Galvez Rodriguez B (2012) Influence of grafting on the yield and quality of tomato cultivars grown in greenhouse in Central Spain. Acta Hortic 927:449–454
Hu B (2016) Improved tomato grafting techniques. Dissertation, Ohio State University
Ibrahim M, Munira MK, Kabir MS, Islam AKMS, Miah MMU (2001) Seed germination and graft compatibility of wild Solanum as rootstock of tomato. Online J Biol Sci 1:701–703
Ilankoon J, Zoysa IJD, Wijesekara A (2001) Tomato grafting as a remedy for bacterial wilt in a protected agriculture system. Ann Sri Lanka Dept Agric 3:53–60
Iott MC (2013) Utility of grafting and evaluation of rootstocks for the management of Verticillium wilt in tomato production in western North Carolina. Dissertation, North Carolina State University
Izal ER (2014) Valoración agronómica de la variedad de tomato Caramba (Lycopersicon esculentum) en invernadero: Ensayo de distintos patrones. Dissertation, Universidad Pública de Navarra
Johnson SJ (2012) Grafting eggplant, tomato, and watermelon to manage Verticillium wilt caused by Verticillium dahliae. Dissertation, Washington State University
Kaskavalc G, Tuzel Y, Dura O, Oztekin GB (2009) Effects of alternative control methods against Meloidogyne incognita in organic tomato production. Ekoloji 18(72):23–31
Kaskavalci G, Akkurt HD (2012) Organik domates tarmnda Kok-ur nematodlar (Meloidogyne spp.)'na kars savasta baz yontemlerin birlikte kullanm etkinlikleri = Efficacy of the combined usage of several control methods against root-knot nematodes (Meloidogyne spp.) in organic tomato agriculture. Turk Entomol Derg 36:413–422
Kawaguchi M, Taji A, Backhouse D, Oda M (2008) Anatomy and physiology of graft incompatibility in solanaceous plants. J Hortic Sci Biotechnol 83:581–588
Khah EM, Kakava E, Mavromatis A, Chachalis D, Goulas C (2006) Effect of grafting on growth and yield of tomato (Lycopersicon esculentum Mill.) in greenhouse and open-field. J Appl Hortic 8:3–7
Korenak P (2010) Primerjava različnih podlag za cepljenje paradižnika (Lycopersicon esculentum Mill.) = Comparison of different rootstock for grafting tomatoes (Lycopersicon esculentum Mill.). Dissertation, Univerze v Mariboru, Slovenia
Kosec M (2011) Hidroponsko pridelovanje cepljenega paradižnika (Lycopersicum esculentum L. = Hidroponic growing of grafted tomato (Lycopersicum esculentum L.). Dissertation, Univerza v Ljubljani, Slovenia
Kowalczyk K, Gajc-Wolska J (2011) Effect of the kind of growing medium and transplant grafting on the cherry tomato yielding. Acta Sci Polon Hort Cult 10:61–70
Krumbein A, Schwarz D (2013) Grafting: a possibility to enhance health-promoting and flavour compounds in tomato fruits of shaded plants? Sci Hortic 149:97–107
Kumar P, Edelstein M, Cardarelli M, Ferri E, Colla G (2015a) Grafting affects growth, yield, nutrient uptake, and partitioning under cadmium stress in tomato. HortScience 50:1654–1661
Kumar P, Lucini L, Rouyphael Y, Cardarelli M, Kalunke RM, Colla G (2015b) Insight into the role of grafting and arbuscular mycorrhiza on cadmium stress tolerance in tomato. Front Plant Sci 6:477
Kumar P, Rouphael Y, Cardarelli M, Colla G (2015c) Effect of nickel and grafting combination on yield, fruit quality, antioxidative enzyme activities, lipid peroxidation, and mineral composition of tomato. J Plant Nutr Soil Sci 178:848–860
Kunwar S, Paret ML, Olson SM, Ritchie L, Rich JR, Freeman JH, McAvoy T (2015) Grafting using rootstocks with resistance to Ralstonia solanacearum against Meloidogyne incognita in tomato production. Plant Dis 99:119–124
Latifah E, Korlina E, Boga K, Maryono J (2013) Grafting technology for sustainable improvement of tomato production: a field study in Kediri, East Java. In: Proceedings International Conference, 2013, 4th Green Technology, pp 49-53
Lee MH, Kim JK, Lee HK, Kim KJ, Yu SH, Kim YS, Lee YS (2013) Reduction of bacterial wilt diseases with eggplant rootstock EG203-grafted tomatoes in the field trials. Res Plant Dis 19:108–113
Li GL, Zhang SP, Yang HL, Liang XA (2009) Study on grafting methods with Xianchongjue No. 3 as rootstock. J Changjiang Veg 2009(10):47–49
Liang XA, Liang FF, Zhang SP, Jian H, Yang HL, Xie KY (2014) Studies on disease resistance and grafting effects of Xianchong-jue tomato rootstock series. J Henan Agric Sci 43:85–88
Loos RA, Caliman FRB, Silva DJHD (2009) Enxertia, produção e qualidade de tomateiros cultivados em ambiente protegido = Grafting, production and quality of tomato growth in protected environment. Ciênc Rural 39:232–235
Lopez-Perez JA, Strange ML, Kaloshian I, Ploeg AT (2006) Differential response of Mi gene-resistant tomato rootstocks to root-knot nematodes (Meloidogyne incognita). Crop Prot 25:382–388
Lykas C, Kittas C, Zambeka A (2008) Water and fertilizers use efficiency in grafted and non grafted tomato plants on soilless culture. Acta Hortic 801:1551–1556
Ma HF, Wang XL, Chen LD (2004) Comparison of main properties of grafted and common seedling in Israel tomato. J Changjiang Veg 2004(11):48–49
Mahmoud AMA (2014) Grafting as a tool to improve TYLCV-tolerance in tomato. J Hortic Sci Ornam Plant 6:109–115
Marsic NK, Osvald J (2004) The influence of grafting on yield of two tomato (Lycopersicon esculentum Mill.) cultivars grown in a plastic house. Acta Agric Slovenica 83:243–249
Martinez S, Garbi M, Grimaldi MC, Somoza J, Morelli G, Cerisola C (2014) Evaluacion de la respuesta agronomica de plantas de tomate injertadas en cultivo bajo invernadero = Evaluation of agronomic performance of grafted tomato plants in greenhouse cultivation. Rev Fac Agron La Plata 113:218–223
Masterson SA (2013) Propagation and utilization of grafted tomatoes in the Great Plains. Dissertation, Kansas State University
Masterson SA, Rivard CL, Janke RR, Kennelly MM (2015) Effect of seedling shoot removal on the yield of grafted tomatoes in high tunnels in the central United States. Acta Hortic 1107:173–179
Mazuela A, Cepeda P, Cubillos V (2012) Efecto del injeto y del bioestimulante Fartum(R) sobre la produccion y calidad en tomate cherry = Effect of grafting and the biostimulant Fartum on production and quality in cherry tomatoes. Idesia 30:77–81
McAvoy T, Freeman JH, Rideout SL, Olson SM, Paret ML (2012b) Evaluation of grafting using hybrid rootstocks for management of bacterial wilt in field tomato production. HortScience 47:621–625
Meyer L (2016) Grafting to increase high tunnel tomato productivity in the central United States. Dissertation, Kansas State University
Miguel A, Marsal JI, Goto R, San Bautista A, Lopez-Galarza S, Pascual B, Maroto JV (2011) Improving the affinity of tomato grafted on Solanum torvum using an intermediate rootstock. Acta Hortic 898:291–295
Mišković A, Vujasinović V, Vukosavlijević V, Ilin Ž (2005) Efekti primene kalemljenja na kvalitet I prinos ploda paradajza = Effects of grafting on quality and yield of tomato fruits. Letopis Naucnih Radova 29:204–209
Mohammed SMT, Humidan M, Boras M, Abdalla OA (2009) Effect of grafting tomato on different rootstocks on growth and productivity under glasshouse conditions. Asian J Agric Res 3:47–54
Mohsenian Y, Roosta HR, Karimi HR, Esmaeilizade M (2012) Investigation of the ameliorating effects of eggplant, datura, orange nightshade, local Iranian tobacco, and field tomato as rootstocks on alkali stress in tomato plants. Photosynthetica 50:411–421
Montesano FF, Parente A, Grassi F, Santamaria P (2014) Posidonia-based compost as a growing medium for the soilless cultivation of tomato. Acta Hortic 1034:277–282
Neocleous D (2010) Yield, nutrients, and antioxidants of tomato in response to grafting and substrate. Int J Veg Sci 16:212–221
Neu K, Nair A (2017) Evaluation of grafted and non-grafted hybrid and heirloom tomatoes in a high tunnel. Farm Prog Rep 2016:7–13
Nicoletto C, Tosini F, Sambo P (2013a) Effect of grafting and ripening conditions on some qualitative traits of ‘Cuore di bue’ tomato fruits. J Sci Food Agric 93:1397–1403
Nicoletto C, Tosini F, Sambo P (2013b) Effect of grafting on biochemical and nutritional traits of ‘Cuore di Bue’ tomatoes harvested at different ripening stages. Acta Agric Scand B Soil Plant Sci 63:114–122
Nilsen ET, Freeman J, Grene R, Tokuhisa J (2014b) A rootstock provides water conservation for a grafted commercial tomato (Solanum lycopersicum L.) line in response to mild-drought conditions: a focus on vegetative growth and photosynthetic parameters. PLoS One 9:e115380
Nitzsche PJ, Kline W, Rabin J (2013) The influence of grafting on high tunnel tomato production. Proc N J Agric Convention Trade Show New Jersey Agric Convention Trade Show 58:12–14
Ntatsi G, Savvas D, Ntatsi G, Klaring HP, Schwarz D (2014) Growth, yield, and metabolic responses of temperature-stressed tomato to grafting onto rootstocks differing in cold tolerance. J Am Soc Hortic Sci 139:230–243
O’Connell S (2008) Grafted tomato performance in organic production systems: nutrient uptake, plant growth, and fruit yield. Dissertation, North Carolina State University
Oda M, Okada K, Sasaki H (2000) Effects of transplant container and Solanum rootstocks on the incidences of overgrowth and unmarketable fruits in tomato plants planted with plug seedlings. Environ Control Biol 38:273–280
Onduso JN (2014) Management of bacterial wilt of tomato by use of resistant rootstock. Dissertation, University of Nairobi, Kenya
Owusu SB, Kwoseh CK, Starr JL, Davies FT (2016) Grafting for management of root-knot nematodes, Meloidogyne incognita, in tomato (Solanum lycopersicum L.). Nematropica 46:14–21
Oztekin GB, Giuffrida F, Tuzel Y, Leonardi C (2009b) Is the vigour of grafted tomato plants related to root characteristics? J Food Agric Environ 7:364–368
Oztekin GB, Tuzel Y, Tuzel IH (2013b) Does mycorrhiza improve salinity tolerance in grafted plants? Sci Hortic 149:55–60
Oztekin GB, Tuzel Y (2011) Salinity response of some tomato rootstocks at seedling stage. Afr J Agric Res 6:4726–4735
Palada MC, Ali M (2007) Evaluation of technologies for improving year-round production of safe vegetables in peri-urban agriculture of Southeast Asia. Acta Hortic 762:271–281
Palada MC, Wu DL (2007b) Increasing off-season tomato production using grafting technology for peri-urban agriculture in Southeast Asia. Acta Hortic 742:125–131
Palkovics L, Petroczy M, Hevesi M, Salamon P (2007) A globalizacio kockazata: uj novenyi korokozok megjelenese hazankban = The risk of globalization: appearance of new plant pathogens in Hungary. In: 12. Tiszantuli Novenyvedelmi Forum, 17-18 October 2007, Debrecen, Hungary, pp 33-35
Paret ML, Freeman J, McAvoy T, Rideout S, Olson SM (2012) Grafting for management of bacterial wilt and root-knot nematodes in tomato production. Fla Tomato Inst Proc 2012:9–11
Patakioutas G, Dimou D, Kostoula O, Yfanti P, Paraskevopoulos A, Ntatsi G, Savvas D (2015) Inoculation of tomato roots with beneficial micro-organisms as a means to control Fusarium oxysporum f. sp. lycopersici and improve nutrient uptake and yield. Acta Hortic 1107:141–148
Pedo T, Aumonde TZ, Oliveira LDC, Nora L, Mauch CR (2013) Produtividade e caracteristicas qualitativas do tomateiro submetidas a enxertia = Yield and qualitative characteristics of grafted tomato. Rev Cienc Agrar / Amazon J Agric Environ Sci 56:179–183
Peil RMN, Galvez JL (2004) Redimiento de plantas de tomate injertadas y efecto de la densidad de tallos en el sistema hidroponico = Yield of tomato crop as a result of grafting and shoot density in hydroponic system. Hortic Bras 22:265–270
Pogonyi A, Pek Z, Helyes L, Lugasi A (2005) Effect of grafting on the tomato’s yield, quality and main fruit components in spring forcing. Acta Aliment 34:453–462
Qaryouti MM, Qawasmi W, Hamdan H, Edwan M (2007) Tomato fruit yield and quality as affected by grafting and growing system. Acta Hortic 741:199–206
Qin D, Li YH, Li YY, Mo HK, Liu CC (2013) Field screening for disease-resistant rootstocks of tomato. J Changjiang Veg 2013:20–22
Rahmatian A, Delshad M, Salehi R (2014) Effect of grafting on growth, yield and fruit quality of single and double stemmed tomato plants grown hydroponically. Hortic Environ Biotechnol 55:115–119
Riga P (2015) Effect of rootstock on growth, fruit production and quality of tomato plants grown under low temperature and light conditions. Hortic Environ Biotechnol 56:626–638
Riga P, Benedicto L, Garcia-Flores L, Villano D, Medina S, Gil-Izquierdo A (2016) Rootstock effect on serotonin and nutritional quality of tomatoes produced under low temperature and light conditions. J Food Compos Anal 46:50–59
Rivard CL, Louws FJ (2008b) Grafting to manage soilborne diseases in heirloom tomato production. HortScience 43:2104–2111
Rivard CL, O'Connell S, Peet MM, Louws FJ (2010) Grafting tomato with interspecific rootstock to manage diseases caused by Sclerotium rolfsii and southern root-knot nematode. Plant Dis 94:1015–1021
Rivard CL, O’Connell S, Peet MM, Welker RM, Louws FJ (2012b) Grafting tomato to manage bacterial wilt caused by Ralstonia solanacearum in the southeastern United States. Plant Dis 96:973–978
Romano D, Paratore A (2001) Effects of grafting on tomato and eggplant. Acta Hortic 559:149–153
Semiz GD, Suarez DL (2015) Tomato salt tolerance: impact of grafting and water composition on yield and ion relations. Turk J Agric For 39:876–886
Shou W, Dong W, Chen J, Xu Z, Zhou S, Dai D, Lei J (2004) Effects of rootstock varieties and grafting methods on growth and photosynthesis of tomato. Acta Agric Zhejiang 16:136–138
da Silva EG (2015) Resposta enzimátálógica e produtiva do tomateiro e desempenho de porta enxertos resistentes à murcha bacteriana. Dissertation, Universidade Estadual Paulista Júlio de Mesquita Filho, Campus de Botucatu
da Silva ES (2013) Enxertia no controle da murcha bacteriana, na atividade de enzimas e produçã em tomateiro. Dissertation, Universidade Estadual Paulista Júlio de Mesquita Filho, Campus de Botucatu
Silverman EJ (2015) Inoculation methods and screening of selected tomato accessions for bacterial wilt incidence and managing bacterial wilt by grafting with disease resistant rootstocks in North Carolina. Dissertation, North Carolina State University
Singh S, Singh DR, Kumar K, Birah A (2014) Eco-friendly management modules for bacterial wilt (Ralstonia solanacearum) of tomato for protected cultivation in a tropical island ecosystem. Biol Agric Hortic 30:219–227
Sirtoli LF, Cerqueira RC, de Souza Fernandes LM, Rodrigues JD, Goto R, do Amaral JL (2008) Avaliação de diferentes porta-enxertos de tomateiro cultivados em ambiente protegido = Use of rootstocks in plants of tomato cultivated under protecting culture. Biodiversidade 7:24–28
Sirtoli LF, Cerqueira RC, Rodrigues JD, Goto R, Braga CL (2011) Enxertia no desenvolvimento e qualidade de frutos de tomateiro sob diferentes porta-enxertos em cultivo protegido = Grafting in the development and fruit quality of tomato under different rootstocks in protected cultivation. Sci Agrar Parana 10:15–22
Suchoff D, Gunter C, Schultheis J, Louws FJ (2015) On-farm grafted tomato trial to manage bacterial wilt. Acta Hortic 1086:119–128
Theodoropoulou A, Giotis C, Hunt J, Gilroy J, Toufexi E, Liopa-Tsakalidis A, Markellou A, Lueck L, Seal C, Leifert C (2007) Effect of variety choice and use of resistant rootstock on crop yield and quality parameters of tomato plants grown in organic, low input and conventional production systems/growth media. In: Niggli U, Liefert C, Alfoldi T, Luck L, Willer H (eds) Improving sustainability in organic and low input food production systems. Proceedings of the 3rd International Congress of the European Integrated Project Quality Low Input Food (QLIF), University of Hohenheim, Germany, 20-23 March, 2007. Research Institute of Organic Agriculture, Frick, Switzerland, pp 177-180
Tian Y, Cao Z, Zhang X, Guo W, Mei X, Gao L (2009a) Changes of soil enzyme activities under different agricultural treatments in greenhouse and its correlation analysis. Plant Nutr Fertil Sci 15:857–864
Tian Y, Dong LD, Jiao YG, Guo JH, Shi LQ (2010) Study on yield-increasing mechanism of grafted tomato. J Hebei Agric Sci 14(10):15–17
Tian Y, Zhang X, Liu J, Chen Q, Gao L (2009b) Microbial properties of rhizosphere soils as affected by rotation, grafting, and soil sterilization in intensive vegetable production systems. Sci Hortic 123:139–147
Truffault V, Fifel F, Longuenesse JJ, Vercambre G, le Quillec S, Gautier H (2015) Impact of temperature integration under greenhouse on energy use efficiency, plant growth and development and tomato fruit quality depending on cultivar rootstock combination. Acta Hortic 1099:95–100
Tuhtar S (2011) Gojenje treh sort cepljenega paradižnika (Lycopersicon esculentum Mill.) na dveh podlagah = Three cultivars of grafted tomatoes (Lycopersicon esculentum Mill.) growing on two rootstocks. Dissertation, Univerza v Ljubljani, Slovenia
Turhan A, Ozmen N, Serbec MS, Senz V (2011) Effects of grafting on different rootstocks on tomato fruit yield and quality. Hortic Sci 38:142–149
Turkmen O, Seymen M, Dursun A (2010) Effects of different rootstocks and cultivars on yield and some yield components of grafted tomato. Bull Univ Agric Sci Vet Med Cluj Napoca Hortic 67:284–291
Tuzel Y, Duyar H, Oztekin GB, Gul A (2009) Domates anaclarnn farkl dikim tarihlerinde bitki gelisimi, scaklk toplam istegi, verim ve kaliteye etkileri = Effects of tomato rootstocks on plant growth, temperature sum requirements, yield and quality in different planting dates. Ege Univ Ziraat Fak Derg 46:79–92
Velasco-Alvarado MJ (2013) Anatomia y manejo agronomico de plantas injertadas en jitomate (Solanum lycopersicum L.). Dissertation, Universidad Autonoma Chapingo, Mexico
Velasco-Alvarado MJ, Castro-Brindis R, Castillo-Gonzalez AM, Avitia-Garcia E, Sahagun-Castellanos J, Lobato-Ortiz R (2016) Composicion mineral, biomasa y rendimiento en tomate (Solanum lycopersicum L.) injertado/Mineral composition, biomass and fruit yield in grafted tomato (Solanum lycopersicum L.). Interciencia 41:703–708
Venema JH, Dijk BE, Bax JM, Hasselt PRV, Elzenga JTM (2008b) Grafting tomato (Solanum lycopersicum) onto the rootstock of a high-altitude accession of Solanum habrochaites improves suboptimal-temperature tolerance. Environ Exp Bot 63:359–367
Verdejo-Lucas S, Cortada L, Sorribas FJ, Ornat C (2009) Selection of virulent populations of Meloidogyne javanica by repeated cultivation of Mi resistance gene tomato rootstocks under field conditions. Plant Pathol 58:990–998
Verdejo-Lucas S, Javier Sorribas F (2008) Resistance response of the tomato rootstock SC 6301 to Meloidogyne javanica in a plastic house. Eur J Plant Pathol 121:103–107
Voutsela S, Yarsi G, Petropoulos SA, Khan EM (2012) The effect of grafting of five different rootstocks on plant growth and yield of tomato plants cultivated outdoors and indoors under salinity stress. Afr J Agric Res 7:5553–5557
Vrcek IV, Samobor V, Bojic M, Medic-Saric M, Vukobratovic M, Erhatic R, Horvat D, Matotan Z (2011) The effect of grafting on the antioxidant properties of tomato (Solanum lycopersicum L.). Sp J Agric Res 9:844–851
Wang H, Ru S, Wang L, Fang L, Ren H, Feng Z (2009) Control of tomato bacterial wilt with grafting. Acta Agric Zhejiang 21:283–287
Wang M, Zhang G, Wang X, Wen S, Cui S, Shi Y (2011) A new tomato rootstock variety highly resistant to root-knot nematodes—‘Kezhen No. 1’. China Veg 2011(14):80–83
Wang MY (2010) The influence of tomato rootstocks of immune root-knot nematode on scion. J Anhui Agric Sci 38:2991–2993
Wang S, Kong Y, Yang R, Cheng J, Li H (2012) Effect of rootstocks on fruit quality of tomato growing in solar greenhouse. Acta Hortic 944:147–151
Wimer AF (2009) The spatial and temporal distribution and management of tomato bacterial wilt on Virginia’s Eastern Shore. Dissertation, Virginia Polytechnic Institute and State University
Yan GY (2011) Preliminary report on the grafting cultivation of cherry tomato Fusarium wilt resistance. Hortic Seed 2011(1):27–28
Yarsi G (2011) Effects of grafted seedling use on yield, growth and quality parameters of tomato growing in greenhouse. Acta Hortic 923:311–314
Yasinok AE, Sahin FI, Eyidogan F, Kuru M, Haberal M (2009b) Grafting tomato plant on tobacco plant and its effect on tomato plant yield and nicotine content. J Sci Food Agric 89:1122–1128
Zhang CK, Kang YH, Zhang LS (2009a) Selection of grafted rootstocks of cherry tomato. J Changjiang Veg 2009(14):42–44
Zhang CK, Luo YH, Zhang LS (2008) Effects of grafting cultivation on bacterial wilt resistance and yield of cherry tomato. Acta Agric Jiangxi 20(4):68–69
Zhang S, Liang X, Yang H, Jian H, Wang XE (2009b) Selection and breeding of tomato rootstock variety with resistance to root-knot nematodes. China Veg 2009(16):74–77
Zhang ZH, Xiao JW, Liu Y, Chen SX, Yang JG, Liu WB (2010) Grafting high resistance rootstock King Qing Yi Hao to prevent tomato bacterial wilt. Chin Agric Sci Bull 26(11):276–278
Zheng C, Cao Z, Chen G (2004) Effect of rootstock on tomato growth and yield. China Veg 2004(4):37–38
Zheng CY, Cao ZP, Chen GK, Chen YF, Yang H (2005) Study on the grafted tomatoes controlling root-nematode in greenhouse. Chin J Eco Agric 13:164–166
Zhou C, Zhang X, Yin X (2001) Comparison trial of grafted and self-rooted seedlings of tomato. China Veg 2001(4):32–33
Funding
This study was funded in part by the Specialty Crop Block Grant Program of the U.S. Department of Agriculture (USDA) through Grant 14-SCBGP-CA-0006. Its contents are solely the responsibility of the authors and do not necessarily represent the official views of the USDA. The California Department of Pesticide Regulation also provided partial funding for this project through Pest Management Research Grant 16–PML–R004, but does not necessarily agree with any opinion expressed, nor endorse any commercial product or trade name mentioned.
Author information
Authors and Affiliations
Corresponding author
Ethics declarations
Conflict of interest
The authors declare that they have no conflict of interest.
About this article
Cite this article
Grieneisen, M.L., Aegerter, B.J., Scott Stoddard, C. et al. Yield and fruit quality of grafted tomatoes, and their potential for soil fumigant use reduction. A meta-analysis. Agron. Sustain. Dev. 38, 29 (2018). https://doi.org/10.1007/s13593-018-0507-5
Accepted:
Published:
DOI: https://doi.org/10.1007/s13593-018-0507-5