Abstract
Purpose
Glioblastomas (GBM) comprise 17% of all primary brain tumors. These tumors are extremely aggressive due to their infiltrative capacity and chemoresistance, with glial-to-mesenchymal transition (GMT) proteins playing a prominent role in tumor invasion. One compound that has recently been used to reduce the expression of these proteins is shikonin (SHK), a naphthoquinone with anti-tumor properties. Temozolomide (TMZ), the most commonly used chemotherapeutic agent in GBM treatment, has so far not been studied in combination with SHK. Here, we investigated the combined effects of these two drugs on the proliferation and motility of GBM-derived cells.
Methods
The cytotoxic and proliferative effects of SHK and TMZ on human GBM-derived cells were tested using 3-(4,5-dimethylthiazol-2-yl)-2,5-diphenyl tetrazolium bromide (MTT), Ki67 staining and BrdU incorporation assays. The migration capacities of these cells were evaluated using a scratch wound assay. The expression levels of β3 integrin, metalloproteinases (MMPs) and GMT-associated proteins were determined by Western blotting and immunocytochemistry.
Results
We found that GBM-derived cells treated with a combination of SHK and TMZ showed decreases in their proliferation and migration capacities. These decreases were followed by the suppression of GMT through a reduction of β3 integrin, MMP-2, MMP-9, Slug and vimentin expression via inactivation of PI3K/AKT signaling.
Conclusion
From our results we conclude that dual treatment with SHK and TMZ may constitute a powerful new tool for GBM treatment by reducing therapy resistance and tumor recurrence.
Similar content being viewed by others
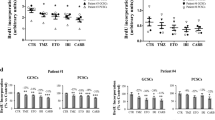
Avoid common mistakes on your manuscript.
1 Introduction
Glioblastomas (GBM) represent the most common malignant primary brain tumors [1, 2]. First-line treatment of GBM comprises surgical resection, followed by radiotherapy with concomitant and adjuvant chemotherapy using temozolomide (TMZ). However, even with treatment, patients survive for only 12 to 15 months after diagnosis [3]. Previous studies have indicated that the limited success of GBM therapy is related to the resistance of glioblastoma cells to TMZ, a DNA alkylating drug [4], as well as to genetic abnormalities and alterations in the signaling pathways responsible for cell proliferation, survival and motility [5, 6].
During invasion and migration, tumor cells alter their phenotype. These alterations involve changes in cell-cell adhesion and polarity in a process called epithelial-mesenchymal transition (EMT), wherein proteins typical of epithelial cells become down-regulated and mesenchymal marker proteins, for instance N-cadherin and vimentin, become up-regulated [7]. Degradation of the extracellular matrix (ECM) by metalloproteinases (MMPs) is essential for the invasiveness of tumor cells, and is also important for the EMT of tumor cells. One of the most important deregulated signaling pathways in GBM is the integrin pathway, which is also involved in cell adhesion, cytoskeletal organization, cell proliferation, survival and invasion [8]. Evidence indicates that integrins have a role in EMT [9]. Overexpression of integrins in tumor cells leads to a reduction in E-cadherin levels and to increases in the levels of vimentin and the transcription factor Slug [10]. Cooperation between integrins and MMPs is essential for the invasiveness of tumor cells [11]. Previous studies have reported that the overexpression of integrin αvβ3 plays an important role in GBM cell migration as well as in MMP-2 regulation, both of which are associated with the high invasiveness of these tumors [12]. Because current drug treatments for GBM are not effective, new molecular compounds have been tested, either alone, combined with flavonoids [13, 14], or as a dual treatment with TMZ [4, 15].
Shikonin (SHK) is an active naphthoquinone isolated from Zicao, more specifically from the root of Lithospermum erythrorhizon, which is used in Chinese herbal medicine [16] and in the experimental treatment of cancer [17, 18], including GBM [19]. SHK is a relatively lipophilic compound, and its molecular structure allows it to cross the blood-brain barrier (BBB). Previous studies have reported that SHK may reduce the expression of β1 integrin in non-small-cell lung cancer cells and decrease the expression of MMP-2 and MMP-9 in prostate cancer cells [17, 18]. The combination of SHK and the topoisomerase I inhibitor topotecan (TPT) has been found to promote cell cycle arrest and apoptosis in GBM cells and in glioblastoma stem-like cells (GSCs) through activation of the caspase-9/3-dependent pathway [20]. Moreover, SHK has been found to reduce the migration and invasion of breast cancer cells by inhibiting EMT through a reduction in the expression of mesenchymal markers such as N-cadherin and Slug, and up-regulation of epithelial markers such as E-cadherin, suggesting that after SHK treatment, the tumor cells re-acquire an epithelial phenotype that is less resistant to therapy [21]. A more recent study has shown that malignant gliomas also express mesenchymal markers such as vimentin, fibronectin and Snail. These observations have resulted in a new concept, i.e., glial-mesenchymal transition (GMT), since the origin of glial tumor cells is different from that of epithelial tumors, such as breast tumors [22].
We hypothesized that a combination of SHK and TMZ may potentiate GBM treatment. To test this hypothesis, GBM cells were incubated with SHK and TMZ, in a dual treatment or individually, after which their proliferation and motility were evaluated. Proteins involved in EMT/GMT of tumor cells, which are associated with therapy resistance, were also analyzed. The results obtained may be instrumental for our understanding of the mode of the action of SHK on GBM cells and for the development of new tools to treat GBM.
2 Material and methods
2.1 Reagents
All culture reagents as well as the secondary antibodies, conjugated to Alexa Fluor 488 or 546, and the Alexa Fluor 568 phalloidin were obtained from Life Technologies (Carlsbad, CA, USA). Protease and phosphatase inhibitors, and the Bromodeoxyuridine kit (BrdU) were purchased from Roche (Indianapolis, IN, USA). Antibodies directed against integrin β3 and MMP-9 were purchased from Santa Cruz Biotechnology, Inc. (Dallas, TX, USA). Rabbit anti-MMP-2 and mouse anti-actin antibodies were obtained from Millipore (Billerica, MA, USA), and α-tubulin antibody from Sigma-Aldrich Corp. (St. Louis, MO, USA). Antibodies directed against AKT, phospho-AKT Ser473 and Slug were purchased from Cell Signaling Technology, Inc. (Danvers, MA, USA), and anti-vimentin, Ki67 and GFAP antibodies from Dako (Glostrup, Denmark). Anti-GAPDH antibody was purchased from Abcam (Cambridge, MA, USA). SHK was purchased from Calbiochem (Merck, Darmstadt, Germany). TMZ and other chemicals were purchased from Sigma-Aldrich Corp. SHK and TMZ were dissolved in dimethyl sulfoxide (DMSO) at stock concentrations of 34.68 mM and 100 mM, respectively. These stocks were aliquoted and diluted with culture medium according to the concentration used, and stored frozen at −80 °C.
2.2 Cell culture conditions
The human GBM cell line U118-MG was obtained from the American Type Culture Collection ATCC (USA), whereas GBM cell line GBM02 was established in the Brazilian laboratory as previously described [23]. Primary astrocyte cultures were prepared and cultured as described previously by our group [24]. The cells were cultured in Dulbecco’s modified Eagle’s medium (DMEM) supplemented with 3.5 mg/ml glucose, 0.1 mg/ml penicillin, 0.14 mg/ml streptomycin and 10% fetal bovine serum (FBS). The cultured cells were maintained at 37 °C in an atmosphere of 95% air and 5% CO2. The vehicle controls contained the same amount of DMSO in all treatments. In all conditions, we used concentrations below the IC50 of SHK (1.5 μM) and/or TMZ (200 μM), taking into account the daily doses of TMZ used in clinical practice (150 mg/m2 once per day for 5 days). The drug concentration necessary to inhibit viability was determined using GraphPad Prism 5 (version 5.00; GraphPad Software, Inc., San Diego, CA, USA).
2.3 MTT assay
Cell viability was assessed using a 3-(4,5-dimethylthiazol-2-yl)-2,5-diphenyl tetrazolium bromide (MTT) reduction colorimetric assay. Briefly, cells were seeded into 96-well plates at 103 cells/well and incubated for 24 h with TMZ and/or SHK at different concentrations prepared from stocks. Then, MTT (5 mg/ml) was added to each well at a final concentration of 10% and left for 2 h. The blue formazan crystals produced were dissolved by adding 100 μl DMSO after which the absorbance of the cell culture medium was measured in a microplate reader at 570 nm. The drug concentration required to inhibit growth by 50% (IC50) was estimated using GraphPad Prism 6.
2.4 Ki67 staining and BrdU incorporation assay
GBM cells were treated for 24 h with 1.5 μM SHK alone or in combination with 200 μM TMZ. The proliferation capacity of the GBM cells was determined by two methods: evaluation of Ki67 expression by immunofluorescence using an anti-Ki67 antibody (Dako) and quantification of BrdU incorporation into the DNA of replicating cells using a Cell Proliferation ELISA kit according to the manufacturer’s instructions (Cell Proliferation ELISA for BrdU, Roche).
For the Ki67 expression experiments, GBM cells incubated with 1.5 μM SHK and/or 200 μM TMZ were fixed with 4% PBS/paraformaldehyde, incubated with 3% PBS/BSA for 1 h at room temperature, and then incubated overnight with a rabbit anti-Ki67 antibody in 1% PBS/BSA. The resulting immune complexes were revealed using an anti-rabbit Alexa Fluor 488 antibody. As a negative control absence of anti-Ki67 was used.
For the BrdU incorporation experiments, cells treated for 24 h with 1.5 μM SHK and/or 200 μM TMZ were incubated with BrdU labeling solution (0.1 μl/ml) for 120 min at 37 °C in a humidified atmosphere (5% CO2). Next, the cells were incubated with a FixDenat solution and with anti-BrdU POD (anti-BrdU-FLUOS) according to the manufacturer’s instructions (Roche). Colorimetric analyses were performed using a VICTOR X3 multi-label plate reader, and the absorbances were determined at 450 nm (Perkin-Elmer, Waltham, MA, USA).
2.5 Scratch wound assay
Cell migration was studied according to a previously described method [25]. Briefly, U118-MG and GBM02 cells were seeded into 24-well plates until they reached confluence. Cell monolayers were then scraped in straight lines with a p10 pipet tip, and debris was removed by washing the monolayers with new culture medium. Next, the plates were placed in a CO2/temperature chamber attached to a phase-contrast microscope, and images were captured before treatment with 1.5 μM SHK and/or 200 μM TMZ (0 h), and then after 24 h of treatment. ImageJ software (v1.46; National Institutes of Health, Bethesda, MD, USA) was used to analyze the images, and the scratched areas were determined using the “Polygon Selection Tool” for each time-point and treatment. The results were normalized to the scratched areas at 0 h.
2.6 Cytoskeleton organization assay
GBM cells were seeded onto round coverslips in 24-well plates and incubated with 1.5 μM SHK alone or in combination with 200 μM TMZ for 24 h. In order to visualize actin filaments (F-actin), the cells were fixed with 4% PBS/paraformaldehyde at room temperature for 20 min, permeabilized with 0.1% Triton X-100/PBS for 3 min, and then incubated with an Alexa Fluor 568 phalloidin staining solution (5 μg/ml) for 1 h. To visualize the microtubules, the cells were fixed with methanol at −20 °C for 5 min, permeabilized with 0.1% Triton X-100/PBS for 3 min, and then incubated for 45 min with an anti-α-tubulin antibody (Sigma) in PBS containing 1% BSA. Anti-rabbit Alexa Fluor 488 was used as a secondary antibody. The nuclei were stained with 4′,6-diamidino-2-phenylindole (DAPI). Cells were examined using a DMi8 advanced fluorescence microscope (Leica Microsystems, Germany). The plug-in Fibril Tool for ImageJ (NIH, USA) was used to quantify the anisotropy of F-actin and the microtubules [26]. The anisotropy parameter reaches its maximum when all fibers in the array point in the same direction. Its value is defined as zero when all fibers are randomly oriented. Cells stained only with the secondary antibody were used as a negative control.
2.7 Immunocytochemistry
GBM cells were seeded onto round coverslips in 24-well plates and then incubated with either 1.5 μM SHK or 200 μM TMZ, or both for 24 h. To detect intermediate filament, metalloproteinase and integrin expression, the cells were fixed and then incubated with rabbit anti-GFAP (1:250, Dako), mouse anti-vimentin (1:100, Dako), mouse anti-actin (1:500, Millipore), mouse anti-MMP9 (1:400, Santa Cruz Biotechnology), rabbit anti-MMP2 (1:500, Millipore) and goat anti-integrin (1:200, Santa Cruz Biotechnology) primary antibodies at 4 °C overnight. Next, the cells were washed with PBS and incubated with specific Alexa secondary antibodies at 37 °C for 1 h. The nuclei were stained with DAPI. The slides were examined using a DMI8 advanced fluorescence microscope (Leica Microsystems). The fluorescence intensity of individual cells was measured and analyzed using ImageJ software (NIH).
2.8 Western blotting
GBM cells were cultured in 6-well plates and incubated for 24 h in the presence of either 1.5 μM SHK or 200 μM TMZ, or both for 24 h. Next, the cells were washed with PBS and scraped off using a rubber policeman, after which lysis buffer was added (10 mM Tris base, 0.25 M saccharose and1 mM EDTA, in the presence of protease inhibitors). The lysates were sonicated and then centrifuged at 4 °C, 10,000 g for 10 min. The supernatants were analyzed for protein content using a bicinchoninic acid assay (BCA) kit (Thermo Fisher Scientific, Inc.). Western blotting was performed as described by Towbin et al. [27] with minor modifications introduced by us.
For the immunodetection of proteins, 30 μg total cell protein was separated by electrophoresis on 10% SDS-polyacrylamide gels and transferred to polyvinylidene difluoride (PVDF) membranes. Next, the membranes were blocked with 5% non-fat milk in Tris-buffered saline with 0.1% Tween-20 (TBS-T) for 1 h, incubated with specific primary antibodies overnight at 4 °C, washed with TBS-T, and incubated with peroxidase-conjugated antibodies. The signals of GFAP (1:1000, Dako), MMP-2 (1:200, Millipore), Slug (1:1000, Cell SignalingTechnology), vimentin (1:500, Dako), Phospho-AKT Ser473 (1:2000, Cell SignalingTechnology), AKT (1:1000, Cell SignalingTechnology), GAPDH (1:2500, Abcam) and actin (1:1000, Millipore) were detected using ECL and chemiluminescence in conjunction with a ChemiDoc MP imaging system (BioRad, Benicia, CA, USA). The densitometric analyses were performed using ImageJ software, and the values obtained represent the ratio between the immunodetected protein and the loading control (GAPDH or actin).
2.9 O6-methylguanine-DNA-methyltransferase (MGMT) expression analysis
U118-MG and GBM02 cells were cultured under standard conditions as described under 2.2 and total RNA was extracted using a PureLink RNA Mini Kit (Thermo Fisher Scientific) following the manufacturer’s instructions. Five micrograms of total RNA and a High-Capacity cDNA Reverse Transcription Kit (Applied Biosystems) were used to perform cDNA synthesis from astrocytes (positive control) and each GBM cell line. Quantitative polymerase chain reactions (qPCR) were carried out in quadruplicate using 50 ng cDNA, MGMT Taqman® Probe (Hs04419844_s1) and GAPDH as the endogenous control (RT2 profiler PCR array, Qiagen). To calculate relative fold variations in mRNA expression, the 2-ΔΔCT method was used and the data were analyzed using Student’s t-test. Thermal cycling was always carried out using the conditions recommended by the manufacturer in a CFX96 Touch Real-Time PCR Detector (BioRad).
2.10 Statistical analysis
All values were expressed as mean ± SD. Groups were compared by means of One-way ANOVA test, Dunnet’s test and Bonferroni probabilities (except for qPCR, as mentioned above), with a significance threshold of p ≤ 0.05. All statistical analyses were performed using GraphPad Prism 6 (GraphPad Software Inc., San Diego, CA, USA).
3 Results
3.1 Dual treatment of SHK and TMZ reduces glioblastoma cell viability
In order to evaluate the effects of SHK on the viability of glioblastoma cells, U118-MG and GBM02 cells were incubated with different concentrations of SHK (0–10 μM) for 24 h. As shown in Fig. 1a, we found that the two cell lines responded differently to the SHK treatment, which may be due to the heterogeneity observed among GBMs. The GBM02 cells were more sensitive to SHK at concentrations of 0.75 μM and above, compared to the U118-MG cells, which showed decreases in viability only at SHK concentrations of 1.5 μM and above. The effect of SHK on the viability of both GBM cell lines was more evident at higher concentrations, such as 2 μM. The differences noted between these cell lines are in agreement with the IC50 values determined for each cell line exposed to SHK, i.e., the SHK IC50 values for GBM02 and U118-MG were 1.68 and 1.87 μM, respectively. Therefore, we decided to use SHK at a concentration of 1.5 μM in all further experiments. Since all TMZ concentrations tested on the two GBM cell lines did not affect their viabilities (Fig. 1b), we decided to use a concentration of 200 μM as previously described to treat the U118-MG and U87 cells [15].
Shikonin in combination with temozolomide reduces glioblastoma-derived cell viability. GBM02 and U118-MG cells were treated with different concentrations of shikonin (SHK, 0.2–10 μM) (a), temozolomide (TMZ, 50–250 μM) (b), and co-treated with both drugs at different concentrations: cells treated simultaneously with 0.75, 1 or 1.5 μM SHK and 200 μM TMZ (c), and cells treated simultaneously with 150, 200 or 250 μM TMZ and 1.5 μM SHK (d) for 24 h. Each value represents the mean ± SD of three independent experiments, *p < 0.05, **p < 0.01, ***p < 0.001, # p < 0.0001, compared to control groups (vehicle) and to treatment with each drug alone
We found that the viability of GBM02 cells was reduced at 1.5 μM SHK and did not change significantly in response to the TMZ treatment compared to the control cells (Fig. 1c). In the case of U118-MG cells, incubation with 1.5 μM SHK reduced their viability, although the TMZ treatment affected them similarly to GBM02 cells. These data reinforce our supposition (see above) about differences between these two cell lines. The differences were more evident when the cells were incubated with 1.5 μM SHK in combination with 200 μM TMZ. The reduction in cell viability was greater than that observed with each drug alone or with the control (Fig. 1c and d). This effect was more pronounced for GBM02 cells than for U118-MG cells (for details see Table 1). Together, these data confirm the differences in the nature of the two lines regarding different responses to SHK and TMZ treatments. These differences emphasize the importance of analyzing the profiles of different tumors, since they may respond differently to the same treatment regimens. Biochemical studies, such as those described here, combined with magnetic resonance imaging (MRI) and anatomopathological analyses may help to improve the treatment of these tumors.
3.2 Glioblastoma cells express GFAP, vimentin and MGMT at different levels
The different responses of GBM02 and U118-MG cells to SHK and TMZ, as outlined above, suggest that these two cell lines are different in nature. Therefore, we assessed the presence of putative additional differences between these two cell lines by determining the expression of the glial fibrillary acidic (GFAP) and vimentin proteins using immunofluorescence and Western blotting (Fig. 2a and b, respectively). We found that the two cell lines exhibited different distributions of the GFAP and vimentin proteins (Fig. 2a), which were accompanied by differences in their synthesis (Fig. 2b) (for details see Table 1). GBM02 cells were found to contain less vimentin than U118-MG cells, suggesting that the U118-MG cells are less glial-differentiated than the GBM02 cells. Since vimentin is expressed by cells with a mesenchymal phenotype, the high vimentin synthesis in U118-MG cells additionally suggests that these cells still have a glial-to-mesenchymal transition (GMT) profile whereas the GBM02 cells are in a more advanced stage of differentiation. These results could be related to the differences observed during SHK and TMZ treatment, wherein the GBM02 cells turned out to be more sensitive than the U118-MG cells based on their mesenchymal profiles, which are known to be associated with the aggressiveness and chemo-resistance of tumor cells. We also determined the MGMT mRNA expression levels in the U118-MG and GBM02 cells using qPCR. We found that the GBM02 cells showed a significantly lower (almost null) MGMT expression level compared to the U118-MG cells (Supplementary Fig. 1). The different MGMT expression levels observed may, at least in part, explain the different responses elicited by SHK and TMZ in these cell lines.
Glioblastoma-derived cells express different levels of GFAP and vimentin. Expression and distribution of vimentin and GFAP in GBM02 and U118-MG cells assessed by immunofluorescence (a) and Western blot analysis (b) using specific antibodies. Actin was used as loading control and 10 μg protein was loaded in each lane. Each value represents the mean ± SD of three independent experiments, *p < 0.05
3.3 SHK in combination with TMZ reduces glioblastoma cell proliferation
In order to determine whether the reduction in viability of GBM cells treated with SHK or TMZ alone could be associated with a decrease in cell proliferation, we next assayed the Ki67 expression and BrdU incorporation levels in these cells. Upon incubation with 1.5 μM SHK the percentage of Ki67-positive cells decreased in both cell lines, compared to control cells (Fig. 3a). In cells incubated with TMZ, the expression of Ki67 decreased less than in the control and SHK-treated cells (Fig. 3a). The effect was clearly enhanced when cells from both lines were incubated with 1.5 μM SHK in combination with 200 μM TMZ. In cells incubated with both drugs combined, we found that the percentage of Ki67-positive cells decreased compared to the control cells and to the treatment with each drug individually (Fig. 3a) (for details see Table 1). By using a BrdU incorporation assay, we found that upon incubation with 1.5 μM SHK alone, the proliferation rate decreased in both GBM02 and U118-MG cells compared to control cells (Fig. 3b). When the GBM cells were treated with 200 μM TMZ alone, the proliferation in both cell lines was found to be less reduced than in control cells and in SHK treated cells (Fig. 3b). Moreover, we found that a combined treatment with SHK and TMZ reduced the cell viability in both cell lines compared to the control and to treatment with each drug individually (for details see Table 1). The anti-proliferation effect was, however, more prominent in GBM02 cells than in U118-MG cells. These results suggest an anti-proliferative effect when both drugs are combined in a dual treatment.
Shikonin in combination with temozolomide affects glioblastoma-derived cell proliferation. GBM02 and U118-MG cells were treated with 1.5 μM shikonin (SHK), 200 μM temozolomide (TMZ), and the combination of 1.5 μM SHK and 200 μM TMZ for 24 h. Cell proliferation was evaluated by Ki67 staining (a) and BrdU incorporation assays (b). The results are expressed as mean ± SD and are representative of three independent experiments, with each experiment performed in triplicate.*p < 0.05, **p < 0.01, ***p < 0.001 compared to the vehicle groups and to the TMZ and SHK treatments alone. Scale bar: 50 μm
3.4 SHK in combination with TMZ reduces glioblastoma cell migration
In order to next evaluate whether SHK and/or TMZ treatments alter the migration capacity of GBM cells, scratch wound assays [25] were performed. We found that when GBM02 and U118-MG cells were incubated with 1.5 μM SHK alone, their migration capacity was reduced compared to that of control cells (Fig. 4b and d). Based on the above observations, we expected that TMZ treatment would not reduce GBM cell migration, but surprisingly, we found that the migration capacity of both the GBM02 and U118-MG cells was decreased compared to control cells (Fig. 4a and b) (for details see Table 1). Furthermore, we found that upon treatment with 1.5 μM SHK and 200 μM TMZ, the reduction in migration capacity was more pronounced in the GBM02 cells (Fig. 4a and b) than in U118-MG cells (Fig. 4c and d). These data suggest that the dual treatment was more effective in reducing cell migration than treatment with SHK or TMZ alone (for details see Table 1).
Shikonin in combination with temozolomide reduces glioblastoma-derived cell migration. GBM02 (a) and U118-MG (b) cell migration capacities were evaluated using a wound-closure (scratch) assay after treatment with 1.5 μM shikonin (SHK), 200 μM temozolomide (TMZ) or a combination of 1.5 μM SHK and 200 μM TMZ. Cells were photographed at the start of the assay and 24 h later. The area of cell migration was measured for both cell lines. *p < 0.05, **p < 0.01, ***p < 0.001 compared to the vehicle groups and to TMZ and SHK treatment alone. Scale bars: 50 μm
3.5 SHK in combination with TMZ changes cytoskeleton organization in glioblastoma cells
In order to evaluate whether the observed reduction in the migratory capacity of GBM cells is associated with alterations in the cytoskeletal architecture, we analyzed the organization of actin filaments and microtubules by immunocytochemistry. To this end, F-actin was stained using phalloidin and an α-tubulin antibody was used to detect microtubules. We found that in untreated GBM02 and U118-MG cells, the F-actin filaments remained organized (Fig. 5a and b). However, when both cell lines were treated with 1.5 μM SHK alone, the organization of F-actin filaments became significantly disrupted (Fig. 5a) compared to the control condition. In contrast, we found that treatment with 200 μM TMZ alone induced fewer alterations in the F-actin organization in GBM02 cells, and did not alter the F-actin filaments in U118-MG cells compared to the changes observed in these cells incubated with 1.5 μM SHK alone (Fig. 5a). When the GBM cells were incubated with 1.5 μM SHK and 200 μM TMZ, the F-actin filaments were more disorganized compared to those treated with SHK alone (Fig. 5a), suggesting an additive effect of these drugs. Regarding the microtubule organization upon incubation with 1.5 μM SHK alone, as seen in Fig. 5b, the organization was impaired in both cell lines compared to that observed in control cells. On the other hand, we found that in both cell lines treated with 200 μM TMZ alone, the microtubule organization remained similar to that observed in control cells (Fig. 5b). Interestingly, when the cells were incubated with 1.5 μM SHK in a dual treatment with 200 μM TMZ (Fig. 5b), the microtubules were considerably more disorganized compared to those in the presence of SHK alone.
Cytoskeleton organization in glioblastoma cells treated with shikonin and temozolomide. GBM02 and U118-MG cells were treated for 24 h with 1.5 μM shikonin (SHK), 200 μM temozolomide (TMZ) or a combination of both drugs. (a) red indicates F-actin staining, and blue indicates nuclear staining (DAPI). (b) green indicates α-tubulin staining, and blue indicates nuclear staining (DAPI). The images are representative of three independent experiments, obtained by DMI8 advanced fluorescence microscopy. Anisotropy was quantified using the FibrilTool plugin. *p < 0.05, **p < 0.01, ***p < 0.001, compared to the vehicle control and to TMZ and SHK treatment alone. Scale bar: 30 μm
To better quantify the fibrillar structures for each condition, the anisotropy of F-actin filaments (Fig. 5a) and microtubules (Fig. 5b) was determined using the Fibril tool plugin for ImageJ [26]. Upon incubation with 1.5 μM SHK alone, the anisotropy of F-actin filaments decreased in both cell lines, compared to control cells (Fig. 5b). In the presence of 200 μM TMZ alone, the anisotropy of F-actin filaments was reduced only in GBM02 cells, and no effect was observed in U118-MG cells (Fig. 5a). In cells incubated with 1.5 μM SHK in combination with 200 μM TMZ, the anisotropy of F-actin filaments was more reduced in GBM02 cells than in U118-MG cells and control cells, which suggests a pronounced additive effect. Regarding the anisotropy of microtubules, we found that in cells treated with 1.5 μM SHK alone, anisotropy was reduced in both cell lines compared to control cells (Fig. 5b). In cells incubated with 200 μM TMZ alone anisotropy decreased, but not significantly, in both GBM02 and U118-MG cells (Fig. 5b) (for details see Table 1). In cells incubated with 1.5 μM SHK in combination with 200 μM TMZ, we found that anisotropy of the microtubules was decreased considerably more in GBM02 than in U118-MG cells compared to control cells and to the treatment with each drug alone. These data suggest that GBM02 and U118-MG cells are sensitive to SHK and TMZ dual treatment regarding cytoskeleton disorganization.
3.6 SHK in combination with TMZ reduces β3 integrin and MMP-2/9 expression in glioblastoma cells
Since we found that SHK and TMZ co-treatment disrupts the cytoskeletal organization and migration of GBM cells, we next set out to investigate whether integrins and metalloproteinases could be affected by the combination of these two drugs. We found that GBM02 and U118-MG cells incubated with the combination of 1.5 μM SHK and 200 μM TMZ showed a significant reduction in the expression of the β3 integrin subunit, compared to control cells and to each treatment alone (Fig. 6a). GBM02 cells showed a higher sensitivity than U118-MG cells, which remained near the control levels. In addition we found that GBM cells incubated with the combination of 1.5 μM SHK and 200 μM TMZ exhibited a reduction in the expression of the metalloproteinases MMP-9 (Fig. 6b) and MMP-2 (Fig. 6c), as determined by immunofluorescence. The expression of the MMPs was significantly reduced in both cell lines compared to control cells and to each treatment alone (Fig. 6b and c). This reduced expression may indirectly lead to the decreased migration of GBM cells. Subsequent Western blot analysis confirmed the immunofluorescence results, i.e., GBM02 and U118-MG cells incubated with the combination of SHK and TMZ showed significant reductions in MMP-2 expression levels compared to control cells (Fig. 6d).
Expression of integrin and metalloproteinases in glioblastoma-derived cells treated with the combination of shikonin and temozolomide. GBM02 and U118-MG cells were treated for 24 h with 1.5 μM shikonin (SHK), 200 μM temozolomide (TMZ) or a combination of both drugs. The (a) β3 integrin (yellow), (b) MMP-9 (pink) and (c) MMP-2 (green) fluorescence intensities were quantified (right-hand plots). (d) Western blot analysis of MMP-2 expression. As a loading control α-tubulin was used. Each value represents the mean ± SD of three independent experiments (*p < 0.05, **p < 0.01, ***p < 0.001, compared to the vehicle control. Scale bar: 30 μm
3.7 SHK in combination with TMZ suppresses GMT in glioblastoma cells
Considering the reduction of cellular migration and cytoskeleton disorganization, we next investigated the effects of the SHK and TMZ co-treatment on the activation of signaling pathways involved in GBM migration and invasion, such as the AKT pathway [28]. The phosphorylation at Ser473 of AKT is known to induce the activation of the GMT phenotype in tumor cells, thereby increasing the expression of Slug [29]. Slug, in turn, is known to be associated with suppression of the epithelial phenotype in tumor cells, and to be necessary for the migration and invasion of GBMs [30]. Additionally, it has been shown that GBMs express high levels of Slug and CD44, the latter of which interacts with MMPs and is correlated with the chemo-resistance and invasiveness of tumors [31]. We found that 200 μM TMZ treatment did not affect the phosphorylation of AKT (phospho-AKT), whereas 1.5 μM SHK treatment reduced the phospho-AKT levels only in U118-MG cells (Fig. 7a). Co-treatment with 1.5 μM SHK and 200 μM TMZ, however, significantly decreased the phospho-AKT levels compared to control cells and SHK treatment alone (Fig. 7a). We found that mesenchymal-related proteins were expressed in both GBM cell lines, as detected by Western blotting (Fig. 7a), and that SHK treatment decreased the expression of GMT genes/activators such as Slug and vimentin in both cell lines compared to untreated cells (Fig. 7a). Moreover, we found that the combined treatment of SHK and TMZ significantly reduced the expression of Slug and vimentin in both cell lines (Fig. 7a). These results strongly suggest that the GMT phenotype can be reduced by SHK and TMZ dual treatment in GBM cells. These results also support the idea that SHK and TMZ dual treatment decreases the aggressiveness of GBM cells through GMT instability and, consequently, reduces the migration of GBM cells (summarized in Fig. 7b).
Effect of shikonin and temozolomide co-treatment on the PI3K/AKT signaling pathway and the expression of GMT-associated proteins in glioblastoma-derived cells. After 24 h, (a) Western blot analysis was performed using specific antibodies directed against phospho-AKT Ser473 (p-AKT) and total-AKT to evaluate the effect of shikonin (SHK) and temozolomide (TMZ) on PI3K/AKT signaling. The Slug and vimentin protein levels were detected to reveal the effect of SHK and TMZ on the GMT of GBM cells. GAPDH was used as loading control. Each value represents the mean ± SD of three independent experiments, *p < 0.05, **p < 0.01, ***p < 0.001, compared to the vehicle control. Scale bar: 30 μm. (b) Schematic summary of the effect of SHK and TMZ on GBM cells. SHK and TMZ co-treatment reduces the expression of GMT proteins
4 Discussion
Since the survival of GBM patients is still poor [3], the identification of new compounds with anti-tumor properties is a major challenge. Previously, preclinical studies and clinical trials have been conducted to investigate the possibility of increasing the efficacy of TMZ by combining it with other pharmacological agents [32]. SHK, a naphthoquinone, has also been subject of cancer research because of its anti-tumor and lipophilic properties, which facilitate its crossing of the blood-brain barrier. Previous work has shown that SHK is a weak inducer of cancer drug resistance, and can be administered chronically for at least 18 months without significantly activating cellular and molecular processes underlying drug resistance. This property makes SHK suited for treating drug-resistant tumor cells [33]. It has been found that combinatorial use of the conventional chemotherapeutic agents paclitaxel and SHK can prolong survival and reduce breast tumor volumes in animal models, suggesting that SHK can be used as an adjuvant in breast cancer treatment [34]. Moreover, combination of SHK with topotecan, a topoisomerase I inhibitor, has been found to promote cell cycle arrest and apoptosis in glioblastoma cells and glioblastoma stem-like cells by activating the caspase 9/3-dependent pathway [20].
This information prompted us to assess the effects of SHK on GBM cells and to determine whether its combination with TMZ may be more effective in reducing the aggressive behavior of GBM cells compared to TMZ alone. We found that SHK significantly decreased the viability of different GBM cells (GBM02 and U118-MG) in a dose-dependent manner. We also found that the sensitivities of these two GBM cell lines differed, and that the effects in GBM02 cells were more pronounced than those in U118-MG cells. Treatment with SHK alone was sufficient to decrease the viability of both cell lines, whereas TMZ alone was not effective. Consistently, it has been reported that GBM cells are resistant to DNA alkylating agents, depending on their expression of MGMT [35]. We observed that both cell lines express MGMT, but that GBM02 cells showed minimal levels compared to U118-MG cells. This suggests that the GBM02 cells may have a different resistance to TMZ treatment. On the other hand, when combining both agents (SHK + TMZ), we observed a significant decrease in viability in both cell lines when compared to each treatment alone. Surprisingly, GBM02 cells were found to be more sensitive to the combined treatment. This observation prompted us to assess whether these two cell lines differ in the expression of GFAP and vimentin, which are known to be related to the aggressiveness of gliomas [36]. We indeed found that the GBM cell lines express different levels of GFAP and vimentin (strong, weak and negative). They were also found to differ in their morphology, i.e., elongated, fibroblastic or flattened shapes. These differences may explain the observed differences in drug responses, including differences in MGMT expression. Moreover, SHK proved to be more effective than TMZ in reducing cellular viability at both concentrations used here. These results confirm that TMZ seems to be less efficacious in decreasing the viability of these GBM cells, which is in agreement with our previous studies [4, 15]. Our results also indicate that dual treatment with SHK and TMZ may have a synergic effect resulting in a more significant decrease in cell viability.
We also found that the reduction in cell viability was accompanied by a decrease in cell proliferation. The combination of SHK with TMZ reduced the number of Ki67-positive cells, as well as BrdU incorporation by 65.4% and 56.3% in GBM02 and U118-MG cells, respectively. Our results are consistent with those reported by Zhang et al. [20] who found that SHK can induce cell cycle arrest in glioblastoma cells. In addition, we found that SHK alone and SHK in combination with TMZ induced a significant reduction in cell migration. Therefore, we hypothesize that SHK may act by triggering cytoskeletal organizing factors implicated in cell motility (i.e., MMPs and integrins), in conformity with previous studies showing a reduction in migration, invasion and adhesion in human breast, gastric and prostate cancer cells [17]. Since both F-actin and microtubules are associated with cell motility, we hypothesized that the impaired migration observed could be explained by a disorganization of these structures. These observations could also explain the decrease observed in cell proliferation induced by the combination of SHK and TMZ. Our data strongly suggest an additive effect. Previous studies have shown that cancer drugs can affect the organization of both F-actin and microtubules as well as cell cycle progression through impairment of specific cellular signaling pathways such as the RhoA-ROCK-LIMPK2-TPPP and the RAS/MAPK pathways [37].
Integrins play important roles in the proliferation, invasion and migration of cells through modulating the cytoskeleton [8, 38]. A non-small-cell lung cancer study has shown that treatment with SHK can significantly reduce both the mRNA and protein levels of β1 integrin [18]. It has also been found that SHK can decrease the MMP-2 and MMP-9 expression levels in prostate cancer cells, leading to a reduction in cell migration and invasion [17]. Here, we found that the treatment of GBM cells with a combination of SHK and TMZ reduced the expression of the β3 integrin subunit. Previous studies have shown that the migration and invasion of cancer cells also depends on a cooperation between MMP-2, MMP-9 and αvβ3 integrin [39]. According to these observations, we considered that the reduction in GBM cell migration could also be associated with a decrease in expression of both metalloproteinases and integrins. Others have also shown that interactions between integrins and MMPs are crucial for the formation of new blood vessels and for the ability of tumors to invade the parenchyma [40, 41]. In addition, it has been shown that MMP-2 and MMP-9 inhibition in GBM cells reduces the migration of endothelial cells and decreases the formation of blood vessels, resulting in a low angiogenic index [40, 42, 43]. Based on our observation that dual treatment with SHK and TMZ induced significant decreases in the expression of β3 integrin, MMP-2 and MMP-9, we conjecture that this drug combination may reduce the ability of glioblastoma cells to migrate.
Previous studies have reported that MMP-2 and MMP-9 may also contribute to the multi-resistance of tumor cells to conventional treatment regimens [44, 45]. We found that SHK reduced the expression of MMP-2 and MMP-9, but that a dual treatment of SHK and TMZ was more effective in reducing GBM cell proliferation and migration. We used lower concentrations of SHK than those employed by Zhang et al. [46] and, by doing so, found that SHK at a dose of 1.5 μM inhibited MMP-2 and MMP-9 expression in GBM cells, as has also been observed by others in U87 and U251 cells [46]. Co-treatment of SHK with TMZ enhanced the inhibition of MMPs compared to each agent alone, highlighting the importance of combining the two compounds when treating cancer cells.
During the invasion process, GBM cells also acquire a GMT phenotype, which means that they switch their phenotype from glial to mesenchymal-like and begin to express typical EMT proteins such as N-cadherin, vimentin and the transcription factor Slug, which is known to enhance the therapy resistance of tumor cells [7]. Evidence has shown that GMT may be decreased to improve the efficacy of drugs that are usually used to treat cancer. Here, we show that a combination of SHK and TMZ inhibited the GMT of GBMs. SHK and TMZ co-treatment suppressed the expression of vimentin and Slug, which was accompanied by a reduction in the phosphorylation of AKT at Ser473, member of a signaling pathway that is extremely important for the activation of signals for invasion, migration and chemo-resistance of tumor cells [29, 46]. These results are in accordance with other studies that demonstrated the efficacy of SHK in reducing EMT in different cancers, such as breast and hepatocellular cancers [21, 47].
In conclusion, we found that SHK can reduce the expression of a wide range of molecules involved in EMT-like transitions in GBM cells, including MMPs, integrin, Slug and vimentin, via the PI3K/AKT signaling pathway. As a consequence, GBM cell proliferation and migration were found to be reduced. When combined with TMZ, SHK may overrule TMZ chemo-resistance, making GBM cells more susceptible to this alkylating agent and intensifying the reduction in the expression of EMT proteins as was observed in SHK treated cells. Together, these data indicate that a combination of SHK and TMZ may be employed for the design of novel GBM therapeutic approaches.
References
F.B. Furnari, T. Fenton, R.M. Bachoo, A. Mukasa, J.M. Stommel, A. Stegh, W.C. Hahn, K.L. Ligon, D.N. Louis, C. Brennan, L. Chin, R.A. DePinho, W.K. Cavenee, Malignant astrocytic glioma: Genetics, biology, and paths to treatment. Genes. Dev. 21, 2683–2710 (2007). doi:10.1101/gad.1596707
F.R. Lima, S.A. Kahn, R.C. Soletti, D. Biasoli, T. Alves, A.C. da Fonseca, C. Garcia, L. Romao, J. Brito, R. Holanda-Afonso, J. Faria, H. Borges, V. Moura-Neto, Glioblastoma: Therapeutic challenges, what lies ahead. Biochim. Biophys. Acta. 1826, 338–349 (2012). doi:10.1016/j.bbcan.2012.05.004
R. Stupp, M. E. Hegi, W. P. Mason, M. J. van den Bent, M. J. Taphoorn, R. C. Janzer, S. K. Ludwin, A. Allgeier, B. Fisher, K. Belanger, P. Hau, A. A. Brandes, J. Gijtenbeek, C. Marosi, C. J. Vecht, K. Mokhtari, P. Wesseling, S. Villa, E. Eisenhauer, T. Gorlia, M. Weller, D. Lacombe, J. G. Cairncross, R. O. Mirimanoff, European Organisation for Research, Treatment of Cancer Brain Tumour and Radiation Oncology Groups, National Cancer Institute of Canada Clinical Trials Group. Effects of radiotherapy with concomitant and adjuvant temozolomide versus radiotherapy alone on survival in glioblastoma in a randomised phase III study: 5-year analysis of the EORTC-NCIC trial. Lancet. Oncol. 10, 459–466 (2009) doi: 10.1016/S1470–2045(09)70025–7
S.A. Kahn, D. Biasoli, C. Garcia, L.H. Geraldo, B. Pontes, M. Sobrinho, A.C. Frauches, L. Romao, R.C. Soletti, S. Assuncao Fdos, F. Tovar-Moll, J.M. de Souza, F.R. Lima, G. Anderluh, V. Moura-Neto, Equinatoxin II potentiates temozolomide- and etoposide-induced glioblastoma cell death. Current. Top. Med. Chem. 12, 2082–2093 (2012)
V. Moura-Neto, L. Campanati, D. Matias, C.M. Pereira, C. Freitas, J. M. Coelho-Aguiar, T.C.L.S. Spohr, A.L. Tavares-Gomes, D. Pinheiro-Aguiar, S.A. Kahn, J. Balça-Silva, B. Pontes, I. Porto-Carreiro, J. Faria, R.A.P. Martins, S. Lima-Costa, M. F. Dias-Costa, M.C. Lopes, F.R.S. Lima. Glioblastoma and the special role of adhesion molecules in their invasion, ed. by A. Sedo, R. Mentlein (Springer-Verlag Wien, 2014), p. 293. doi:10.1007/978–3–7091-1431-5
H.J. Anderson, D.S. Galileo, Small-molecule inhibitors of FGFR, integrins and FAK selectively decrease L1CAM-stimulated glioblastoma cell motility and proliferation. Cell. Oncol. 39, 229–242 (2016). doi:10.1007/s13402-016-0267-7
B.N. Smith, N.A. Bhowmick, Role of EMT in metastasis and therapy resistance. J. Clin. Med. 5, pii:E17 (2016). doi:10.3390/jcm5020017
J.S. Desgrosellier, D.A. Cheresh, Integrins in cancer: Biological implications and therapeutic opportunities. Nat. Rev. Cancer 10, 9–22 (2010). doi:10.1038/nrc2748
F.A. Mamuya, M.K. Duncan, aV integrins and TGF-β-induced EMT: A circle of regulation. J. Cell. Mol. Med. 16, 445–455 (2012). doi:10.1111/j.1582-4934.2011.01419.x
C.Y. Liu, H.H. Lin, M.J. Tang, Y.K. Wang. Vimentin contributes to epithelial-mesenchymal transition cancer cell mechanics by mediating cytoskeletal organization and focal adhesion maturation. Oncotarget 6, 15966–15983 (2015) doi:10.18632/oncotarget.3862
L.J. McCawley, L.M. Matrisian, Matrix metalloproteinases: Multifunctional contributors to tumor progression. Mol. Med. Today 6, 149–156 (2000). doi:10.1016/S1357-4310(00)01686-5
E. Godefroy, A. Moreau-Aubry, E. Diez, B. Dreno, F. Jotereau, Y. Guilloux. alpha v beta3-dependent cross-presentation of matrix metalloproteinase-2 by melanoma cells gives rise to a new tumor antigen. J. Exp. Med. 202, 61–72 (2005) doi: 10.1084/jem.20042138
R.C. Soletti, G.P. de Faria, J. Vernal, H. Terenzi, G. Anderluh, H.L. Borges, V. Moura-Neto, N.H. Gabilan, Potentiation of anticancer-drug cytotoxicity by sea anemone pore-forming proteins in human glioblastoma cells. Anti-Cancer Drugs 19, 517–525 (2008). doi:10.1097/CAD.0b013e3282faa704
B.L. Santos, M.N. Oliveira, P.L. Coelho, B.P. Pitanga, A.B. da Silva, T. Adelita, V.D. Silva, F. Costa Mde. R.S. El-Bacha, M. Tardy, H. Chneiweiss, M.P. Junier, V. Moura-Neto, S.L. Costa. Flavonoids suppress human glioblastoma cell growth by inhibiting cell metabolism, migration, and by regulating extracellular matrix proteins and metalloproteinases expression. Chem. Biol. Interact. 242, 123–138 (2015) doi: 10.1016/j.cbi.2015.07.014
J. Balca-Silva, D. Matias, A. do Carmo, H. Girao, V. Moura-Neto, A.B. Sarmento-Ribeiro, M.C. Lopes. Tamoxifen in combination with temozolomide induce a synergistic inhibition of PKC-pan in GBM cell lines. Biochim. Biophys. Acta 1850, 722–732 (2015) doi:10.1016/j.bbagen.2014.12.022
X. Chen, L. Yang, J.J. Oppenheim, M.Z. Howard, Cellular pharmacology studies of shikonin derivatives. Phytother. Res. 16, 199–209 (2002). doi:10.1002/ptr.1100
Y. Chen, L. Zheng, J. Liu, Z. Zhou, X. Cao, X. Lv, F. Chen, Shikonin inhibits prostate cancer cells metastasis by reducing matrix metalloproteinase-2/−9 expression via AKT/mTOR and ROS/ERK1/2 pathways. Int. Immunopharmacol. 21, 447–455 (2014). doi:10.1016/j.intimp.2014.05.026
H. Wang, C. Wu, S. Wan, H. Zhang, S. Zhou, G. Liu, Shikonin attenuates lung cancer cell adhesion to extracellular matrix and metastasis by inhibiting integrin β1 expression and the ERK1/2 signaling pathway. Toxicology 308, 104–112 (2013). doi:10.1016/j.tox.2013.03.015
Q. Zhao, N. Kretschmer, R. Bauer, T. Efferth, Shikonin and its derivatives inhibit the epidermal growth factor receptor signaling and synergistically kill glioblastoma cells in combination with erlotinib. Int. J. Cancer 137, 1446–1456 (2015). doi:10.1002/ijc.29483
F.L. Zhang, P. Wang, Y.H. Liu, L.B. Liu, X.B. Liu, Z. Li, Y.X. Xue, Topoisomerase I inhibitors, shikonin and topotecan, inhibit growth and induce apoptosis of glioma cells and glioma stem cells. PLoS One 8, e81815 (2013). doi:10.1371/journal.pone.0081815
D. Hong, S.Y. Jang, E.H. Jang, B. Jung, I.H. Cho, M.J. Park, S.Y. Jeong, J.H. Kim, Shikonin as an inhibitor of the LPS-induced epithelial-to-mesenchymal transition in human breast cancer cells. Int. J. Mol. Med. 36, 1601–1606 (2015). doi:10.3892/ijmm.2015.2373
R. Mahabir, M. Tanino, A. Elmansuri, L. Wang, T. Kimura, T. Itoh, Y. Ohba, H. Nishihara, H. Shirato, M. Tsuda, S. Tanaka, Sustained elevation of snail promotes glial-mesenchymal transition after irradiation in malignant glioma. Neuro-Oncology 16, 671–685 (2014). doi:10.1093/neuonc/not239
J. Faria, L. Romao, S. Martins, T. Alves, F.A. Mendes, G.P. de Faria, R. Hollanda, C. Takiya, L. Chimelli, V. Morandi, J.M. de Souza, J.G. Abreu, V. Moura Neto, Interactive properties of human glioblastoma cells with brain neurons in culture and neuronal modulation of glial laminin organization. Differentiation 74, 562–572 (2006). doi:10.1111/j.1432-0436.2006.00090.x
L.F. Romao, F.A. Mendes, N.M. Feitosa, J.C. Faria, J.M. Coelho-Aguiar, J.M. de Souza, V. Moura-Neto, J.G. Abreu, Connective tissue growth factor (CTGF/CCN2) is negatively regulated during neuron-glioblastoma interaction. PLoS One 8, e55605 (2013). doi:10.1371/journal.pone.0055605
C.C. Liang, A.Y. Park, J.L. Guan, In vitro scratch assay: A convenient and inexpensive method for analysis of cell migration in vitro. Nat. Protoc. 2, 329–333 (2007). doi:10.1038/nprot.2007.30
A. Boudaoud, A. Burian, D. Borowska-Wykret, M. Uyttewaal, R. Wrzalik, D. Kwiatkowska, O. Hamant, FibrilTool, an ImageJ plug-in to quantify fibrillar structures in raw microscopy images. Nat. Protoc. 9, 457–463 (2014). doi:10.1038/nprot.2014.024
H. Towbin, T. Staehelin, J. Gordon, Electrophoretic transfer of proteins from polyacrylamide gels to nitrocellulose sheets: Procedure and some applications. Biotechnology 24, 145–149 (1979)
K.A. McDowell, G.J. Riggins, G.L. Gallia, Targeting the AKT pathway in glioblastoma. Curr. Pharm. Des. 17, 2411–2420 (2011)
N. Fenouille, M. Tichet, M. Dufies, A. Pottier, A. Mogha, J.K. Soo, S. Rocchi, A. Mallavialle, M.D. Galibert, A. Khammari, J.P. Lacour, R. Ballotti, M. Deckert, S. Tartare-Deckert, The epithelial-mesenchymal transition (EMT) regulatory factor SLUG (SNAI2) is a downstream target of SPARC and AKT in promoting melanoma cell invasion. PLoS One 7, e40378 (2012). doi:10.1371/journal.pone.0040378
Y. Iwadate, Epithelial-mesenchymal transition in glioblastoma progression. Oncol. Lett. 11, 1615–1620 (2016). doi:10.3892/ol.2016.4113
W.Y. Cheng, J.J. Kandel, D.J. Yamashiro, P. Canoll, D. Anastassiou, A multi-cancer mesenchymal transition gene expression signature is associated with prolonged time to recurrence in glioblastoma. PLoS One 7, e34705 (2012). doi:10.1371/journal.pone.0034705
E.M. Goellner, B. Grimme, A.R. Brown, Y.C. Lin, X.H. Wang, K.F. Sugrue, L. Mitchell, R.N. Trivedi, J.B. Tang, R.W. Sobol, Overcoming temozolomide resistance in glioblastoma via dual inhibition of NAD+ biosynthesis and base excision repair. Cancer Res. 71, 2308–2317 (2011). doi:10.1158/0008-5472.CAN-10-3213
H. Wu, J. Xie, Q. Pan, B. Wang, D. Hu, X. Hu, Anticancer agent shikonin is an incompetent inducer of cancer drug resistance. PLoS One 8, e52706 (2013). doi:10.1371/journal.pone.0052706
W. Li, J. Liu, K. Jackson, R. Shi, Y. Zhao, Sensitizing the therapeutic efficacy of taxol with shikonin in human breast cancer cells. PLoS One 9, e94079 (2014). doi:10.1371/journal.pone.0094079
M.E. Hegi, A.C. Diserens, T. Gorlia, M.F. Hamou, N. de Tribolet, M. Weller, J.M. Kros, J.A. Hainfellner, W. Mason, L. Mariani, J.E. Bromberg, P. Hau, R.O. Mirimanoff, J.G. Cairncross, R.C. Janzer, R. Stupp, MGMT gene silencing and benefit from temozolomide in glioblastoma. N. Engl. J. Med. 352, 997–1003 (2005). doi:10.1056/NEJMoa043331
C. Kubelt, K. Hattermann, S. Sebens, H.M. Mehdorn, J. Held-Feindt, Epithelial-to-mesenchymal transition in paired human primary and recurrent glioblastomas. Int. J. Oncol. 46, 2515–2525 (2015). doi:10.3892/ijo.2015.2944
Y.W. Heng, H.H. Lim, T. Mina, P. Utomo, S. Zhong, C.T. Lim, C.G. Koh, TPPP acts downstream of RhoA-ROCK-LIMK2 to regulate astral microtubule organization and spindle orientation. J. Cell. Sci. 125, 1579–1590 (2012). doi:10.1242/jcs.096818
M. Silginer, M. Weller, U. Ziegler, P. Roth, Integrin inhibition promotes atypical anoikis in glioma cells. Cell. Death Dis. 5, e1012 (2014). doi:10.1038/cddis.2013.543
M. Rolli, E. Fransvea, J. Pilch, A. Saven, B. Felding-Habermann, Activated integrin alphavbeta3 cooperates with metalloproteinase MMP-9 in regulating migration of metastatic breast cancer cells. Proc. Natl. Acad. Sci. U S A 100, 9482–9487 (2003). doi:10.1073/pnas.1633689100
S.S. Lakka, C.S. Gondi, N. Yanamandra, W.C. Olivero, D.H. Dinh, M. Gujrati, J.S. Rao, Inhibition of cathepsin B and MMP-9 gene expression in glioblastoma cell line via RNA interference reduces tumor cell invasion, tumor growth and angiogenesis. Oncogene 23, 4681–4689 (2004). doi:10.1038/sj.onc.1207616
C. Chetty, S.S. Lakka, P. Bhoopathi, J.S. Rao, MMP-2 alters VEGF expression via alphaVbeta3 integrin-mediated PI3K/AKT signaling in A549 lung cancer cells. Int. J. Cancer 127, 1081–1095 (2010). doi:10.1002/ijc.25134
A.V. Fonseca, D. Corbeil, The hematopoietic stem cell polarization and migration: A dynamic link between RhoA signaling pathway, microtubule network and ganglioside-based membrane microdomains. Commun. Integr. Biol. 4, 201–204 (2011). doi:10.4161/cib.4.2.14419
U. Jadhav, S. Chigurupati, S.S. Lakka, S. Mohanam, Inhibition of matrix metalloproteinase-9 reduces in vitro invasion and angiogenesis in human microvascular endothelial cells. Int. J. Oncol. 25, 1407–1414 (2004)
Y. Jiao, X. Feng, Y. Zhan, R. Wang, S. Zheng, W. Liu, X. Zeng, Matrix metalloproteinase-2 promotes αvβ3 integrin-mediated adhesion and migration of human melanoma cells by cleaving fibronectin. PLoS One 7, e41591 (2012). doi:10.1371/journal.pone.0041591
J.M. Yang, Z. Xu, H. Wu, H. Zhu, X. Wu, W.N. Hait, Overexpression of extracellular matrix metalloproteinase inducer in multidrug resistant cancer cells. Mol. Cancer Res. 1, 420–427 (2003)
F.Y. Zhang, Y. Hu, Z.Y. Que, P. Wang, Y.H. Liu, Z.H. Wang, Y.X. Xue, Shikonin inhibits the migration and invasion of human glioblastoma cells by targeting phosphorylated β-catenin and phosphorylated PI3K/Akt: A potential mechanism for the anti-glioma efficacy of a traditional Chinese herbal medicine. Int. J. Mol. Sci. 16, 23823–23848 (2015). doi:10.3390/ijms161023823
P.L. Wei, C.C. Tu, C.H. Chen, Y.S. Ho, C.T. Wu, H.Y. Su, W.Y. Chen, J.J. Liu, Y.J. Chang, Shikonin suppresses the migratory ability of hepatocellular carcinoma cells. J. Agric. Food Chem. 61, 8191–8197 (2013). doi:10.1021/jf4009586
Acknowledgements
This study was supported by the Brazilian agencies Conselho Nacional de Desenvolvimento Científico e Tecnológico (CNPq), Coordenação de Aperfeiçoamento de Pessoal de Nível Superior (CAPES), Fundação de Amparo à Pesquisa do Rio de Janeiro (FAPERJ), Pró-Saúde Associação Beneficente de Assistência Social e Hospitalar and Ary Frauzino Foundation for Cancer Research. It was also supported by the Portuguese agencies FEDER funds through the Operational Programme Factors Competitiveness COMPETE, and National Funds through the FCT Fundação para a Ciência e Tecnologia.
Author information
Authors and Affiliations
Corresponding author
Ethics declarations
Conflict of interest
We confirm that this manuscript has been approved by all authors and that there are no known conflicts of interest associated with this publication.
Electronic supplementary material
Supplementary Fig. 1
mRNA expression levels for MGMT. U118-MG and GBM02 cells were cultured under standard conditions (at 37 °C, 5% CO2, 10% FBS). Total RNA was extracted using a PureLink RNA Mini Kit following the manufacturer’s instructions. Five micrograms of total RNA and a High-Capacity cDNA Reverse Transcription Kit were used to perform cDNA synthesis. Astrocytes were used as positive control. The qPCR reaction was performed in quadruplicate using the MGMT Taqman Probe (Hs04419844_s1) and GAPDH (array plate) as endogenous control. To calculate the relative fold variation in mRNA expression, the 2-ΔΔCT method was used. The results show that GBM02 cells exhibit ~zero MGMT expression and U118-MG cells ~15% MGMT expression relative to astrocytes. The MGMT expression differed statistically between the two GBM cell lines. Each value represents the ±SD, *p < 0.05. (GIF 14 kb)
Rights and permissions
About this article
Cite this article
Matias, D., Balça-Silva, J., Dubois, L.G. et al. Dual treatment with shikonin and temozolomide reduces glioblastoma tumor growth, migration and glial-to-mesenchymal transition. Cell Oncol. 40, 247–261 (2017). https://doi.org/10.1007/s13402-017-0320-1
Accepted:
Published:
Issue Date:
DOI: https://doi.org/10.1007/s13402-017-0320-1