Abstract
The aim of the study was to evaluate the immunoexpression of E-cadherin, β-catenin, and Ki-67, as well as the promoter methylation of E-cadherin gene in epithelial ovarian cancer (EOC), as well as to find a possible relationship between the immunoexpression and hypermethylation. Promoter methylation was studied using methylation-specific PCR in 86 malignant cases, 14 low malignant potential (LMP) tumors and 19 benign cystadenomas. Immunohistochemical expression was carried out in 64 malignant cases, 8 LMP tumors, and 11 benign cystadenomas. Immunoexpression of E-cadherin was reduced in EOC, while 100 % expression was seen in LMP tumors and benign cystadenomas. An interesting observation was the nuclear expression of E-cadherin in a high percentage of cancers, which showed a positive correlation with Ki-67. Β-Catenin expression showed heterogeneous localization with increased nuclear localization, which was significantly higher in cases that did not express E-cadherin. Promoter methylation of E-cadherin was 36, 14, and 11 % in EOC, LMP tumors, and benign cystadenomas, respectively. Our results suggest that reduced expression of E-cadherin is associated with promoter methylation of E-cadherin gene, in addition to providing evidence for the aberrant nuclear localization of E-cadherin in EOC.
Similar content being viewed by others
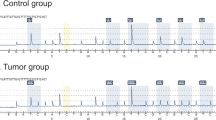
Avoid common mistakes on your manuscript.
Introduction
Ovarian cancer is an important cause of morbidity and mortality especially in the middle-aged women. During the year 2002, it ranked third in frequency (4.1 %) among all cancers in women, with an estimated 204,499 new cases occurring in the world [1]. In India, during the period 2004–2005, the proportion of ovarian cancer varied from 1.7 to 8.7 % of all women with cancers in various urban and rural population based registries (National Cancer Registry, 2008). The incidence of ovarian cancer has been steadily increasing over past 10 years, with an overall life time risk of 1.8 % [2]. Despite new medical and surgical advances, and new chemotherapeutic regimens, the overall 5-year survival for women with advanced stage epithelial ovarian carcinoma has remained relatively unchanged (12 %) over the past 40 years [3].
Epithelial ovarian cancer (EOC) constitutes 90 % of all ovarian tumors. Because ovarian cancer is often asymptomatic in its early stages, the poor prognosis associated with ovarian carcinomas is related to the extensive dissemination of tumor cells beyond the confines of the ovary at the time of diagnosis. Tumor cells that are released into the abdominal cavity are capable of invasion of the peritoneal surfaces following tumor cell implantation [3]. The molecular mechanisms underlying this process are not well characterized. Nevertheless, it has been realized that the loss of epithelial differentiation in carcinomas, which is accompanied by a higher mobility and invasiveness of the tumor cells, is often a consequence of reduced intercellular cell-to-cell adhesion [4].
E-cadherin, a member of the adhesion molecule family of cadherins, mediates predominantly cell–cell adhesion in epithelium and epithelial tumors. It is a tumor suppressor, the loss of which is known to worsen the prognosis of many cancers. The whole function of E-cadherin is not yet well understood, but it might influence the transcription of target genes [5]. Transmembranic glycoprotein E-cadherin interacts with the cytoskeleton via intracellular proteins named catenins. Cell–cell cohesion can be damaged by the loss of E-cadherin expression or changes in catenin expression, which leads to the loss of cadherin function. The cadherin–catenin complex also influences migration and modifies cell growth and the survival of neoplastic cells [6]. In addition, beta-catenin, a member of the catenin family, participates in signal transduction [7].
β-catenin is a 92-kDa multifunctional protein that, in its membrane location, links the intracellular part of the E-cadherin complex to actin cytoskeleton, which is a critical step in morphogenesis and maintenance of tissue integrity [8]. β-catenin interacts with the cytoplasmic tail of cadherins and is critical for the establishment of stable and functional adherent junctions. β-Catenin not only acts as “intercellular glue” in complex with E-cadherin but also takes a part of the Wnt signaling pathway [9]. The Wnt signaling pathway regulates cellular proliferation and differentiation by stabilizing beta-catenin and plays an important role in tumorgenesis. It was suggested that the reduction in β-catenin–E-cadherin complex immunoexpression is related to the intensification of malignancy progression and its metastasizing potential in serous ovarian cancer [10].
The E-cadherin/catenin-mediated cell adhesion system is known to act as an “invasion suppressor system.” A reduced or absent expression or abnormal location of E-cadherin/catenin complex has been observed in several carcinomas including gastric, prostate, colon, and breast cancer [11–13].Few evidence suggest a reduction in E-cadherin or catenin expression to poor prognosis [14], while other studies demonstrate no independent prognostic value for E-cadherin or catenin expression over known prognostic parameters [15].Moreover, a few studies reveal an association between preserved membrane E-cadherin expression and shorter survival in cancers like breast and lung [16, 17].
Therefore, the aim of the present study was to correlate the immunohistological expression of E-cadherin, β-catenin in a cohort of epithelial ovarian tumors with the promoter methylation of E-cadherin gene. The study also looked at the possible relationship between this immunoexpression and tumor proliferation activity expressed by Ki-67 immunoexpression.
Materials and methods
Sample collection and DNA extraction
All tissue samples were collected from a consecutive series of surgical excision specimens (from Aug 2010 to Feb 2012) of patients diagnosed to have ovarian tumor in Kidwai Memorial Institute of Oncology, Bangalore, India. The samples were snap frozen after surgical removal and stored at −80 °C. All samples were verified by histology. The patients included 86 with ovarian malignancy, 14 with a low malignant potential tumor, and 19 with benign cystadenomas. Histological classification was established according to the World Health Organisation (WHO) criteria, and tumor stage was established according to International Federation of Gynaecology and Obstetrics (FIGO). Fifteen cases of normal ovarian tissue were collected from patients without cancer undergoing bilateral salphingo-oophorectomy at the time of surgery for benign gynecological diseases. For tissue microarray construction, only 64 out 86 malignant cases could be retrieved and 8 out 14 for low malignant potential (LMP) tumors and 11 out of 19 for benign cystadenomas. The study was approved by the Institutional Ethics Committee, and all samples were collected after obtaining written, informed consent from patients.
Genomic DNA was extracted from 25 mg of frozen tissue specimens using QIAamp DNA mini kit (Qiagen, CA, USA) following the manufacturer’s instruction. The extracted DNA was examined by electrophoresis, and the yield was measured spectrophotometrically using Eppendorf biospectrophotometer kineticTM before use.
Bisulfite modification and methylation-specific PCR
The methylation status of the promoter region of E-cadherin gene was determined by methylation-specific PCR (MSP). This was further modified as a nested two-step approach in order to increase the sensitivity of detecting allelic hypermethylation at targeted sequences and to facilitate the examination of multiple gene loci. Initially, 600 ng of tissue genomic DNA was treated with bisulfite using the EZ DNA Methylation KitTM (D5001, Zymo Research, CA, USA) following the manufacturer’s protocol. Bisulfite treatment of genomic DNA leads to the conversion of all unmethylated cytosines to uracil, while leaving methylated cytosines unaffected.
The first step of MSP uses a primer set that recognizes the bisulfite-modified template, but it does not discriminate between methylated and unmethylated alleles. In the first PCR step, we used ∼100 ng of modified DNA in a 50-μL reaction mixture containing 5 μL of 10× PCR buffer (New England Biolabs Inc), 1.5 mmol/L of MgCl2, 0.2 μmol/L of each primer, 0.2 mmol/L of dNTPs, and 1 U of Taq polymerase (New England Biolabs Inc.). The PCR amplification was performed in a VeritiTM Thermal Cycler (Applied Biosystems) for 35 cycles, each of which consisted of an initial denaturation at 95 °C for 10 min, denaturation at 95 °C for 30 s, annealing for 30 s, extension at 72 °C for 30 s followed by a final 7-min extension at 72 °C. CpGenome Universal Methylated DNA (Zymo Research, CA, USA) was used as a positive control for methylated DNA. Lymphocyte DNA from healthy controls was used for unmethylated control, and distilled water with no DNA was used as negative control. First-step PCR products were diluted 20-fold, and 2 μL was subjected to second step PCR in a 50-μL volume, with primers specific for methylated and unmethylated alleles. The second step PCR comprised of 35 cycles with 95 °C for 30 s, annealing for 15 s and extension at 72 °C for 30 s.
The characteristics of MSP reactions and the primer sequence of E-cadhein gene are summarized in Table 1. Ten microliters of PCR products was loaded onto 3 % agarose gel and visualized by ethidium bromide staining (Fig. 1). Samples that were negative for both methylated and unmethylated PCR were excluded from the study.
Representative MSP profile of E-cadherin gene promoter methylation in epithelial ovarian tumors. In each case, a universally methylated genomic DNA was used as a positive control and peripheral blood-derived DNA from normal healthy subjects as a negative control. PCR products in lane UM indicate the presence of an unmethylated allele, whereas PCR products in lane M indicate the presence of a methylated allele. C008 and C017 are carcinomas, L006 are LMP tumors. –Ve lanes contained sterile distilled water instead of template DNA
Construction of TMA
The paraffin-embedded tumor tissues were used for constructing tissue microarray (TMA) blocks. Selected cancer foci were marked on hematoxylin and eosin (H&E)-stained sections. A tissue-arraying instrument (Beecher Instruments, Sun Prairie, WI, USA) was used to acquire cylindrical core tissue biopsies with a diameter of 2 mm from histologically representative areas of the donor blocks. Thirty-six tissue cores were composited into a single recipient paraffin block at defined array positions. Two core tissue biopsies were obtained from each specimen and represented in duplicate on the array in this study. Four-micrometer sections were cut from the TMA block and mounted on 2 % aminopropyltriethoxysilane-coated glass slides. The presence of tumor tissue on the arrayed samples was verified on H&E section to confirm tissue morphology.
Immunohistochemistry
Sections were deparaffinized in xylene two times for 15 min each and then placed in a graded series of isopropanol (100, 90, 80, and 70 %). For antigen retrieval, sections were pretreated in a pressure cooker at 121 °C for 15 min in 0.01 M Tris EDTA buffer (pH 9.0) and cooled to room temperature, and to quench the endogenous peroxidase activity, the sections were processed to 3 % H2O2 for 20 min and then rinsed in 0.05 M Tris-buffered saline two times for 3 min each. The sections were incubated with 2 % skimmed milk solution for 30 min at room temperature. After this blocking, sections were incubated 2 1/2 h at room temperature with ready to use mouse anti E-cadherin and anti Ki-67 primary antibodies (BioGenex Laboratories Inc, CA, USA), and mouse anti-β-catenin antibody (at a dilution of 1:75; Invitrogen, Life Technologies, CA). The sections were rinsed three times with phosphate-buffered saline (PBS) for 5 min each and sequentially incubated with biotinylated secondary antibodies for 15 min at room temperature, rinsed three times for 5 min each with PBS and streptavidin–biotin–peroxidase for 5 min at room temperature, and then rinsed three times with PBS for 5 min. The peroxidase reaction was visualized by making use of a solution of 3,3-diaminobenzidine tetrahydrochloride supplemented 0.2 % hydrogen peroxidase in PBS. The sections were then lightly counterstained with hematoxylin. Paraffin embedded tissues from colorectal carcinoma was used as positive control for E-cadherin and β-catenin, whereas reactive lymph nodes were used as positive control for Ki-67 immunostaining. For negative control instead of primary antibody, the slides were incubated in bovine albumin.
Immunohistochemical results were scored independently by two pathologists blinded to the diagnosis. They evaluated membranous (E-cadherin and β-catenin), nuclear (Ki-67), and membranous-cytoplasmic (β-catenin) staining.
E-cadherin and β-catenin scores were recorded as follows: 0 (0+), no staining or fewer than 25 % of cells stained; 1+, 25–50 % of the cells stained; 2+, 50–75 % of cells stained; and 3+, if more than 75 % of the cells stained. 0 and 1+ are considered negative, while 2+ and 3+ are considered positive expression for E-cadherin and β-catenin. The Ki-67 immunoexpression was assessed by means of percentage of immunopositve cells in 1,000 tumor cells for each slide was measured.
Statistical analysis
χ 2 and Fischer exact probability tests were used to determine the significance of association between different variables. Correlation coefficients were calculated using Spearman’s method. All statistical analyses were performed with SPSS 11.0 version statistical software. The level of statistical significance was p < 0.05.
Results
IHC expression of E-cadherin, β-catenin, and Ki-67
Immunohistochemistry (IHC) expression was done on 64 EOC, 8 LMP tumors, and 11 benign cystadenomas (Figs. 2, 3, 4).
Representative immunostaining results for E-cadherin expression. a Serous borderline tumor showing strong E cadherin expression. b Section showing membranous expression of E-cadherin (×400). c Tumor section showing weak membranous staining of E-cadherin (×400). d Tumor section showing nuclear expression with absent membrane expression of E-cadherin (×400)
Representative immunostaining results for β-catenin expression. a Serous type tumor epithelium section showing strong membranous β-catenin expression (×400). b Tumor section showing aberrant localization of β-catenin in cytoplasm and nuclei. c β-Catenin protein expression in the cytoplasm of tumor cells (×400). d Weak β-catenin protein expression detected in the membrane (×400)
E-cadherin expression
Expression of E-cadherin was detected in 45 of 64 (70.3 %) cancer cases, while 100 % expression was seen in LMP tumors and benign cystadenomas. Aberrant nuclear localization of E-cadherin was also seen in 31 of 64 (48.4 %) cases. Thirteen of 31 (41.9 %) cases showed strong nuclear localization with decreased membranous expression (Fig. 2).
β-catenin expression
Fifty-six of 64 (87.5 %) of malignant cases expressed the β-catenin protein. Aberrant protein localization was also seen in the cancer cases. Out of the 56 cases that expressed β-catenin, membranous expression was seen in 29 (51.7 %) cases, while in 27 (48.21 %) of cases, expression was seen in either the cytoplasm or nucleus (Fig. 3).
Ki-67 expression
To correlate proliferation with the above-defined growth patterns and expressions of β-catenin and E-cadherin, we used Ki-67 as proliferation marker. High numbers of Ki-67-expressing cells were found in the central areas of the primary tumors (Fig. 4).
Correlation of E-cadherin and β-catenin protein expression
To understand the relationship between the E-cadherin signaling pathway and associated protein expression, the correlations of E-cadherin and β-catenin protein expression and localization were analyzed (Table 2). As shown in Table 2, in the 19 cases of E-cadherin negative expression, 26.3 % (5 of 19) had β-catenin negative expression, 36.8 % (7 of 19) had β-catenin membrane expression, and 36.8 % (7 of 19) had nuclei/ cytoplasm expression. Additionally, in the 45 cases of E-cadherin-positive expression, 4.4 % (2 of 45) had β-catenin negative expression, 77.7 % (35 of 45) had β-catenin membrane expression, and 17.7 % (8 of 45) had nuclei/ cytoplasm expression. The aberrant protein localization of β-catenin in the E-cadherin negative group was significantly higher than in the positive group.
Correlation of Ki-67 expression with E-cadherin and β-catenin protein expression
In the analyzed group of patients with EOC, we found significant positive correlation between nuclear localization of E-cadherin and Ki-67 expression (r = +0.296; p = <0.05), whereas an inverse correlation was seen between membranous expression of E-cadherin and Ki-67 (r = −0.273; p < 0.05). There was no significant correlation seen between Ki-67 and β-catenin immunoexpression.
Methylation-specific PCR for E-cadherin gene and its correlation with E-cadherin expression
Promoter methylation of E-cadherin was detected in 31 of 86 (36 %) EOC, 2 of 14 (14 %) LMP tumors, and 2 of 19 (11 %) benign cystadenomas. Methylation status of E-cadherin in EOC had no correlation with histological subtype, FIGO stage, tumor grade, menopausal status, and presence or absence of ascitis (Table 3).
Of the 31 malignant cases with E-cadherin promoter methylation, 18 (58 %) were negative for E-cadherin protein expression, which was higher than the loss of E-cadherin protein expression without promoter methylation (3.2 %). There was a significant correlation between E-cadherin gene promoter methylation and E-cadherin protein expression (p < 0.05; Table 4).
Discussion
The molecular mechanisms of cancer are complex and it is evident that genes and proteins may have opposing roles. Cell–cell adhesion appears to be a vital process in tumor cell invasion and metastasis. Experimental evidence has shown that E-cadherin is an important tumor suppressor in a variety of tumor types and has frequently been observed in cancer progression [18, 19]. Reduced cell–cell adhesiveness results in increased cellular motility, resulting in destruction of the histological structure, the morphologic hallmark of malignant tumors [20]. Several reports have indicated that E-cadherin, an epithelial- specific cadherin, is a key molecule for the maintenance of epithelial integrity and of polarized states in association with alpha-, beta-, and gamma-catenin and that the reduction of E-cadherin-mediated cell–cell adhesion favours the dispersion of cancer cells [21].
Immunohistochemical studies have demonstrated that loss of E-cadherin expression is frequently associated with parameters of enhanced biological aggressiveness such as poor histological differentiation, increased invasiveness, metastatic disease, and a poorer survival in patients with oral [22], breast [13], renal [23], nasopharyngeal [24], and esophageal carcinoma [25].
In the current study, we analyzed the expression patterns of E-cadherin, β-catenin, and Ki-67 in EOC, which constitute 90 % of all ovarian malignancies. Firstly, we observed reduced expression of E-cadherin by IHC staining in EOCs against the 100 % expression, which was seen in borderline tumors and benign cystadenomas. Interestingly, in our study, nuclear expression of E-cadherin was observed in 48.4 % of the cancer, while reduced membranous expression with strong nuclear staining was seen in 41.9 % of the cancers.
We have not been able to find any published report of nuclear E-cadherin expression in EOC, although aberrant staining of E-cadherin in the nucleus has been reported in other types of cancer, including Merkel cell carcinoma, signet ring cell carcinoma, colorectal cancer, and solid pseudopapillary tumors of the pancreas [26–29].
Nuclear expression of E-cadherin in our study was significantly correlated with tumor proliferation as assessed by Ki-67 expression, while an inverse correlation was seen between membranous expression of E-cadherin and Ki-67.
The mechanism by which the E-cadherin molecule is translocated to the nucleus is currently not clear. A recent study has shown that E-cadherin nuclear accumulation may result from cleavage of E-cadherin by γ-secretase, releasing the C-terminal fragment, which then translocates into the nucleus assisted by p120-catenin [30]. In the nucleus, the C-terminal fragment can regulate the p120-Kaiso-mediated signaling pathway, which includes the putative Kaiso target gene cyclin D1 [29]. In a recent study of esophageal squamous cell carcinoma, the presence of nuclear E-cadherin staining was shown to be associated with elevated levels of nuclear cyclin D1, and in functional studies, the cytoplasmic domain of E-cadherin was shown to induce cyclin D1 promoter activity [31]. Interestingly, an elevated level of cylin D1 has also been observed in advanced serous EOC predicting poor prognosis of the disease [32].
E-cadherin is only part of a complex cell adhesion system in which the cytoplasmic domain of E-cadherin interacts with the actin filament of the cytoskeleton through the catenins. Several reports are available describing altered expression of β-catenin in cancer cells. Neoplastic cells demonstrated a distinct shift from a membranous localization to a more widespread distribution (membranous, cytoplasmic, and nuclear). Altered expression of β-catenin might compromise the integrity of the E-cadherin/ β-catenin complex and result in weaker cell–cell adhesion in cancer cells.
In our study, also we found that β-catenin was heterogeneously expressed in the cell membrane and cytoplasm of cancer cells. β-catenin showed intense nuclear staining in several epithelial cells. In addition, the frequency of nuclear localization of β-catenin was significantly higher in the E-cadherin expression negative group than in the positive group. The nuclear β-catenin involved in the progression of proliferation has been found in several types of cancers [33]. Loss of β-catenin expression has been reported in advanced stages of serous and clear cell ovarian cancer, associated with poor tumor differentiation and metastasis [34]. Xiao et al. have reported higher abnormal cytoplasmic expression of β-catenin in malignant and borderline ovarian tumors than in benign ones [35]. Reduced E-cadherin- β-catenin complex expression on the cell surface is associated with serous and endometroid subtype and poor differentiation of the tumor. In our study, also decreased expression of E-cadherin in EOC was significantly associated with tumor dedifferentiation (G1 < G2 < G3) in agreement with several previous studies [36].
The reduced E-cadherin expression in tumor cells may be caused by epigenetic silencing via promoter methylation or transcriptional repression, which is reversible [37]. CpG methylation has recently been shown to be an important mechanism in the transcriptional inactivation of E-cadherin, and CpG methylation around the promoter region of E-cadherin was found to be significantly correlated with reduced expression of E-cadherin in various cancers and noncancerous diseases [38, 39]. Hypermethylation in the promoter region of E-cadherin has not been studied in EOC in Indian women. Our study provides evidence to show that promoter methylation of E-cadherin may be involved in the reduction of E-cadherin protein expression.
In our study, promoter methylation of E-cadherin was more frequent in epithelial ovarian tumors (36 %) than LMP tumors (14 %) and benign cystadenomas (11 %). We did not find any promoter methylation in normal ovarian tissue. Our findings are concordant with the findings of Makarla et al. who has reported a methylation frequency of 26 %, 17 % and 13 % for EOC, LMP tumors and benign cystadenomas respectively [40]. Rathi et al. demonstrated that the methylation rate in the E-cadherin gene was significantly higher in tumors compared with non-malignant ovarian tissue [41].
Sixty-seven percent of EOC cases with E-cadherin promoter methylation were negative for E-cadherin protein expression. In addition, 33 % of the EOC cases with E-cadherin protein expression showed E-cadherin promoter methylation, suggesting that decreased expression of E-cadherin protein might be related to promoter hypermethylation.
In conclusion, our study provides evidence to show that promoter methylation of E-cadherin gene may be involved in the reduction of E-cadherin protein expression. Additionally, we also provided evidence for the detection of aberrant protein localization of E-cadherin and β-catenin. Further biomolecular studies are needed to obtain deeper insights into the dynamics of the E-cadherin signalling pathway and tumor progression. To the best of our knowledge no studies have been reported on the relationship of Ki-67 and E-cadherin–β-catenin complex and promoter methylation of the E-cadherin gene among the Indian population in EOC.
References
Parkin DM, Bray F, Ferlay J, Pisani P. Global cancer statistics, 2002. CA Cancer J Clin. 2005;55:74–108.
Jemal A, Thomas A, Murray T. Cancer statistics, 2002. CA Cancer J Clin. 2002;52:23–47.
Ozols RF, Rubin SC, Thomas GM Robboy SJ. Epithelial ovarian cancer. In: Hoskins WJ, Perez CA, Young RC, editors. Principles and practice of gynecologic oncology, 3rd edition. Philadelphia: Lippincott, Williams & Wilkins; 2000. p. 981–1058.
Shiozaki H, Oka H, Inoue M, Tamura S, Monden ME. cadherin mediated adhesion system in cancer cells. Cancer. 1996;77:1605–13. 1540.
Gervais ML, Henry PC, Saravanan A, Burry TN, Gallie BL, Jewett MA, et al. Nuclear E-cadherin and VHL immunoreactivity are prognostic indicators of clear-cell renal cell carcinoma. Lab Invest. 2007;87:1252–64.
Meyer T, Hart IR. Mechanisms of tumour metastasis. Eur J Cancer. 1998;34:214–21.
Karim R, Tse G, Putti T, Scolyer R, Lee S. The significance of the Wnt pathway in the pathology of human cancers. Pathology. 2004;36:120–8.
Aberle H, Butz S, Stappert J, Weissig H, Kemler R, Hoschuetzky H. Assembly of the cadherin-catenin complex in vitro with recombinant proteins. J Cell Sci. 1994;107(Pt 12):3655–63.
Nelson WJ, Nusse R. Convergence of Wnt, β-catenin, and cadherin pathways. Science. 2004;303:1483–7.
Faleiro-Rodrigues C, Macedo-Pinto IM, Maia SS, Vieira RH, Lopes CS. Biological relevance of E-cadherin-catenin complex proteins in primary epithelial ovarian tumors. Gynecol Obstet Invest. 2005;2:75–83.
Chen HC, Chu RY, Hsu PN, Hsu PI, Lu JY, Lai KH, et al. Loss of E-cadherin expression correlates with poor differentiation and invasion into adjacent organs in gastric adenocarcinomas. Cancer Lett. 2003;201:97–106.
Koksal IT, Ozcan F, Kilicaslan I, Tefekli A. Expression of E-cadherin in prostate cancer in formalin-fixed, paraffin-embedded tissues: correlation with pathological features. Pathology. 2002;34:233–8.
Kanazawa T, Watanabe T, Kazama S, Tada T, Koketsu S, Nagawa H. Poorly differentiated adenocarcinoma and mucinous carcinoma of the colon and rectum show higher rates of loss of heterozygosity and loss of E-cadherin expression due to methylation of promoter region. Int J Cancer. 2002;102:225–9.
Yoshida R, Kimura N, Harada Y, Ohuchi N. The loss of E-cadherin, a and b-catenin expression is associated with metastasis and poor prognosis in invasive breast cancer. Int J Oncol. 2001;18:513–20.
Gonzalez MA, Pinder SE, Wencyk PM, Bell JA, Elston CW, Nicholson RI, et al. An immunohistochemical examination of the expression of E-cadherin, alpha- and beta/gamma-catenins, and alpha2- and beta1-integrins in invasive breast cancer. J Pathol. 1999;187:523–9.
Gillett CE, Miles DW, Ryder K, Skilton D, Liebman RD, Springall RJ, et al. Retention of the expression of E-cadherin and catenins is associated with shorter survival in grade III ductal carcinoma of the breast. J Pathol. 2001;193:433–41.
Tan DS, Potts HW, Leong AC, Gillett CE, Skilton D, Harris WH, et al. The biological and prognostic significance of cell polarity and E-cadherin in grade I infiltrating ductal carcinoma of the breast. J Pathol. 1999;189:20–7.
Kase S, Sugio K, Yamazaki K, Okamoto T, Yano T, Sugimachi K. Expression of E-cadherin and beta-catenine in human non-small cell lung cancer and the clinical significance. Clin Cancer Res. 2000;6:4789–96.
Gofuku J, Shiozaki H, Tsujinaka T, Inoue M, Tamura S, Doki Y, et al. Expression of E-cadherin and alpha-catenine in patients with colorectal carcinoma. Correlation with cancer invasion and metastasis. Am J Clin Pathol. 1999;111:29–37.
Hirohashi S. Inactivation of the E-cadherin-mediated cell adhesion system in human cancers. Am J Pathol. 1998;153:333–9.
Birchmeier W, Behrens J. Cadherin expression in carcinomas: role in the formation of cell junctions and the prevention of invasiveness. Biochim Biophys Acta. 1994;1198:11–26.
Ba´nkfalvi A, Krabort M, Buchwalow VA, Vegh A, Felszeghy E, Piffko J. Gains and losses of adhesion molecules (CD44, E-cadherin, b-catenin) during oral carcinogenesis and tumour progression. J Pathol. 2002;198:343–51.
Katagiri A, Watanabe R, Tomita Y. E-Cadherin expression in renal cell cancer and its significance in metastasis and survival. Br J Cancer. 1995;71:376–9.
Xu L, Jiang Y, Zheng J, Xie G, Li J, Shi L, et al. Aberrant expression of β-catenin and E-cadherin is correlated with poor prognosis of nasopharyngeal cancer. Hum Pathol. 2013. doi:10.1016/j.humpath.2012.10.025.
Krishnadath KK, Tilanus HW, van Blankenstein M, Hop WC, Kremers ED, Dinjens WN, et al. Reduced expression of the cadherin–catenin complex in oesophageal adenocarcinoma correlates with poor prognosis. J Pathol. 1997;182:331–8.
Han AC, Soler AP, Tang CK, Knudsen KA, Salazar H. Nuclear localization of E-cadherin expression in Merkel cell carcinoma. Arch Pathol Lab Med. 2000;124:1147–51.
Moon KC, Cho SY, Lee HS, Jeon YK, Chung JH, Jung KC, et al. Distinct expression patterns of E-cadherin and beta-catenin in signet ring cell carcinoma components of primary pulmonary adenocarcinoma. Arch Pathol Lab Med. 2006;130:1320–5.
Cespedes MV, Larriba MJ, Paron MA, Alamo P, Casanova I, Parreno M, et al. Site- dependent E-cadherin cleavage and nuclear translocation in metastatic colorectal cancer model. Am J Pathol. 2010;177(4):2067–79.
Serra S, Salahshor S, Fagih M, Niakosari F, Radhi JM, Chetty R. Nuclear expression of E-cadherin in solid pseudopapillary tumours of the pancreas. J Pancreas. 2007;8:296–303.
Ferber EC, Kajita M, Wadlow A, Tobiansky L, Niessen C, Ariga H, et al. A role for the cleaved cytoplasmic domain of E-cadherin in the nucleus. J Biol Chem. 2008;283:12691–700.
Salahshor S, Naidoo R, Serra S, Shih W, Tsao MS, Chetty R, et al. Frequent accumulation of nuclear E-cadherin and alterations in the Wnt signaling pathway in esophageal squamous cell carcinomas. Mod Pathol. 2008;21:271–81.
Hashimoto T, Yanaihara N, Okamoto A, Nikaido T, Saito M, Takakura S, et al. Cyclin D1 predicts the prognosis of advanced serous ovarian cancer. Exp Ther Med. 2011;2(2):213–9.
Ishida K, Ito S, Wada N, Deguchi H, Hata T, Hosoda M, et al. Nuclear localization of beta-catenin involved in precancerous change in oral leukoplakia. Mol Cancer. 2007;6:62.
Faleiro-Rodriquez C, Macedo-Pinto I. Association of E-cadherin and beta-catenin immunoexpression with clinicopathologic features in primary ovarian carcinomas. Hum Pathol. 2004;6:647–8.
Xiao L, Yu L, Can M. Expression of beta-catenin and APC protein in ovarian epithelial tumor and its implication. Chin J Canc Res. 2007;19:72–5.
Sakuragi N, Nishiya M, Ikeda K, Ohkouch T, Furth EE, Hareyama H, et al. Decreased E-cadherin expression in endometrial carcinoma is associated with tumor dedifferentiation and deep myometrial invasion. Gynecol Oncol. 1994;53:183–9.
Cleton-Jansen AM. E-cadherin and loss of heterozygosity at chromosome 16 in breast carcinogenesis: different genetic pathways in ductal and lobular breast cancer? Breast Cancer Res. 2002;4:5–8.
Park SY, Kim BH, Kim JH, Cho NY, Choi M, Yu EJ, et al. Methylation profiles of CpG island loci in major types of human cancers. J Korean Med Sci. 2007;22:311–7.
Young C-H, Chiu Y-T, Shih T-S, Lin W-R, Chiang C-C, Chou Y-E. E-cadherin promoter methylation may contribute to protein inactivation in pterygia. Mol Vis. 2010;16:1047–53.
Makarla PB, Saboorian MH, Ashfaq R, Toyooka KO, Toyooka S, Minna JD, et al. Promoter hypermethylation profile of ovarian epithelial neoplasms. Clin Cancer Res. 2005;11:5365–9.
Rathi A, Virmani AK, Schorge JO, Elias KJ, Maruyama R, Minna JD, et al. Methylation profiles of sporadic ovarian tumors and nonmalignant ovaries from high-risk women. Clin Cancer Res. 2002;8:3324–31.
Acknowledgment
Rahul Bhagat acknowledges the Director General, Indian Council of Medical Research, New Delhi for providing a senior research fellowship for the present study. The authors thank Dr Sanjay Navani for helping in construction of tissue microarray. We also thank Mr Prashant T. H and Mr Shivshankar for help in IHC staining of tissue microarray slides.
Ethical clearance
The study was approved by Institutional Ethics Committee and all samples were collected after obtaining written, informed consent from patients.
Conflicts of interest
None
Author information
Authors and Affiliations
Corresponding author
Rights and permissions
About this article
Cite this article
Bhagat, R., Premalata, C.S., Shilpa, V. et al. Altered expression of β-catenin, E-cadherin, and E-cadherin promoter methylation in epithelial ovarian carcinoma. Tumor Biol. 34, 2459–2468 (2013). https://doi.org/10.1007/s13277-013-0797-9
Received:
Accepted:
Published:
Issue Date:
DOI: https://doi.org/10.1007/s13277-013-0797-9