Abstract
A cloud-point extraction (CPE) was developed for the determination of 12 phenolic compounds (i.e. gallic acid, procatechuic acid, p-hydroxybenzoic acid, vanillic acid, caffeic acid, syringic acid, p-coumaric acid, ferulic acid, guaiacol, p-cresol, o-cresol and 3,5-xylenol) using reversed-phase high performance liquid chromatography (RP-HPLC) with photodiode array (PDA) detection. The optimum CPE conditions were 2.0% (w/v) Triton X-114, 3.0% (w/v) Na2SO4 and 20-min equilibrated at 45 °C. The surfactant-rich phase was then analyzed by HPLC using a Symmetry C18 column, gradient mobile phase of acetonitrile and 1% (v/v) acetic acid, and PDA detection at 280 nm. Under the optimum condition, the target phenolic compounds were separated within 25 min. CPE gave higher enrichment factor up to 15-fold compared to that of direct analysis. The proposed method showed good analytical performances with limits of detection in the range 0.01–0.1 mg L−1 and precisions with relative standard deviation (RSD) lower than 5% for retention time and 10% for peak area. The method was successfully applied to the analysis of phenolic compounds in Thai local wine samples. Gallic acid, procatechuic acid, and vanillic acid were the highest phenolics found in the studied wines with the contents up to 172.4, 99.1, and 26.6 mg L−1, respectively. The recovery of the spiked wine samples (0.5, 1.0, and 2.0 mg L−1) were obtained in the range of 90.4–110%. High total phenolic content, total flavonoids, and antioxidant activity (DPPH method) in the studied wines were also observed.
Similar content being viewed by others
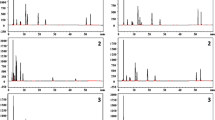
Explore related subjects
Discover the latest articles, news and stories from top researchers in related subjects.Avoid common mistakes on your manuscript.
Introduction
Phenolic compounds are an important group of substances that widely distributed in various plants (e.g. vegetables, fruits, grains, spices, etc.) (Stratil et al. 2007; Vichapong et al. 2010; Pérez-Gregorio et al. 2011; Sharma et al. 2011; Dama et al. 2010; Loganayaki et al. 2011) and also be found in common foods (Vichapong et al. 2010; Rekha and Vijayalakshmi 2010; Sachindra et al. 2010) and plant origin (e.g. teas, wines, and fruit and vegetable juices, etc.) (Alén-Ruiz et al. 2009; García-Falcón et al. 2007; Pérez-Lamela et al. 2007; Roussis et al. 2008; Chen et al. 2011). There is evidence that phenolic substances act as anti-allergic, anti-artherogenic, anti-inflammatory, antioxidant, antimutagens, antimicrobial agents, clarifying agents and metal chelators (Pupponen-Pimiä et al. 2001; Balasundram et al. 2006; Rodrigues et al. 2011). Besides phenolics play an important role in biological system and affect on human health, they are also important to the characteristics and quality of food, especially in their fruit-derived products (e.g. wines, fruits and vegetable juices, etc.). They are served as particular parameter for the organoleptic properties (i.e. color, flavor and teste) of the produced wines (Rodríguez-Delgado et al. 2001; La Torre et al. 2006; García-Falcón et al. 2007; Pérez-Lamela et al. 2007; Alén-Ruiz et al. 2008) and fruit juices (Abad-García et al. 2007; Hamauzu et al. 2008), and also increase wine quality and hygiene due to their anti-bacterial activity (Nave et al. 2007; Malovaná et al. 2001).
Phenolic compounds in wine and fruit juices can be classified into two groups: flavonoids and non-flavonoids (phenolic acids). The major flavonoids include derivatives of the flavonols (e.g. quercetin, myricetin, (+)-catechin, anthocyanins etc.), while the non-flavonoids are p-hydroxybenzoic acid, gallic acid, p-coumaric acid, trans-resveratrol, cis-resveratrol etc. (Woraratphoka et al. 2007; Li et al. 2009). Evaluations of antioxidant activities can be performed using several assays (Li et al. 2009; Rekha and Vijayalakshmi 2010), however, almost are based on free radical scavenging and monitor the absorbance with spectrophotometric detection. DPPH assay is the widely used method, it is successfully applied to various samples including, fruits and cereal (Stratil et al. 2007), fruit juice (Abad-García et al. 2007) and wine (Li et al. 2009). Recently, on-line DPPH HPLC-mass spectrometry (MS) (Wu et al. 2008; Nuengchamnong and Ingkaninan 2009) has been also applied for the analysis of phenolic compounds and their antioxidant activities. Although this method is capable to qualify as well as quantify analytes and evaluate antioxidant activity in a single run, high cost and additional complicated instruments are required. For qualitative and quantitative analysis of individual constituent of the phenolic compounds in samples, reversed-phase high performance liquid chromatography (RP-HPLC) is the most popular technique (Rodríguez-Delgado et al. 2001; Nave et al. 2007; Francisco and Reaurreccion 2009; Prasad et al. 2009). The analysis of phenolic compounds can be performed directly, however sample preparation is also used for purification and preconcentration of the analytes before analysis. Sample preparations for phenolic compounds in beverages are liquid-liquid extraction (LLE) and solid-phase extraction (García et al. 2004; Alén-Ruiz et al. 2009; García-Falcón et al. 2007). Recently, cloud-point extraction (CPE) has been proposed as an interesting extraction and preconcentration technique for analysis of target analytes in different sample matrices (Zhou et al. 2009; Santalad et al. 2009). The main advantages of CPE compared to conventional LLE are able to extract and preconcentrate the target analyte in a single step and use no toxic organic solvents (Zhou et al. 2009; Santalad et al. 2009). In CPE process, non-ionic surfactants are used as an extractant, under suitable conditions (surfactant concentration, salt additive, temperature and time, etc.), the extraction occurs at cloud-point temperature where the surfactant becomes cloudy (at a higher temperature than its critical temperature) resulting in two phases separation involving the surfactant-rich phase (SRP) and the aqueous phase (AQ). The analytes in AQ (large volume) more favorably penetrate into SRP (very small volume) resulting in preconcentration under the clouding phenomena. The obtained small volume of SRP (μL level) is then subjected into the instrumental analysis. To our knowledge, there is a work only applied CPE-HPLC for the analysis of synthetic phenols (i.e. nitrophenol’s family) (Santana et al. 2002), but it has not been proposed for the simultaneous analysis of natural phenolic compounds. In addition, the databases of phenolic compounds in Thai local wines produced from various fruits are also not yet available.
The aim of this work was to develop CPE in combination with HPLC for the simultaneous determination of phenolic compounds in the selected Thai local wines. The antioxidant activity, total phenolic compounds, and content of flavonoids are also investigated using spectrophotometry.
Materials and methods
Chemicals and reagents
The highest purity of phenolic compound standards were used. Gallic acid (GAL), protocatechuic acid (PRO), p-hydroxybenzoic acid (p-HYD), vanillic acid (VAN) and caffeic acid (CAF) were obtained from Acros (USA) whereas syringic acid (SYR), 3-hydroxybenzaldehyde (Internal standard, IS), p-coumaric acid (p-COU), guaiacol (GUA), p-cresol (p-CRE), o-cresol (o-CRE) and 3,5-xylenol (XYL) were purchased from Fluka (Switzerland). Ferulic acid (FER) was obtained from Sigma (Germany). All stock standard solutions (1,000 mg L−1) of phenolic compounds were prepared by dissolving an appropriate amount in methanol. Triton X-114 was purchased from Acros (USA) and used without further purification. A stock Triton X-114 solution (25%, w/v) was prepared in deionized water. Sodium sulphate anhydrous (anh Na2SO4) and sodium carbonate (Na2CO3) were obtained from Merck (Germany). Folin-Ciocalteu reagent was purchased from Carlo Erba (Italy) and 1,1′-diphenyl-2-picryl-hydrazyl (DPPH) was obtained from Sigma (USA). Catechin was obtained from Sigma (Germany) and ascorbic acid was from Carlo Erba (Italy). Sodium nitrite (NaNO2) was obtained from Fluka (Switzerland). Aluminum chloride (AlCl3) and sodium hydroxide (NaOH) were obtained from Carlo Erba (Italy). Deionized water obtained from RiOs™ Type I Simplicity 185 (Millipore Waters, USA) with the resistivity of 18.2 MΩ.cm was used throughout the experiments. Methanol and acetonitrile of HPLC grade were obtained from Lab-Scan Asia, Co., Ltd (Thailand). Acetic acid of analytical reagent (AR) grade was obtained from Carlo Erba (Italy).
Instrumentation
The HPLC system comprised a Waters 600E multisolvent derivery system, a Waters in-line degasser AF, a Rheodyne injector with a sample loop of 20 μL, a Waters 2996 photodiode array detector and a Waters temperature control system. The Empower software was used for data acquisition. Separations were performed on a C18 Waters Symmetry column (3.9 mm i.d. × 150 mm, 5 μm) coupled to a guard column. A thermostatic water bath (ISOTEMP 228, Thermo Fisher Scientific, Massachusetts, USA) was used to implement cloud-point extraction. A centrifuge (Biomed group Co. Ltd, Bangkok, Thailand) was used for complete phase separation.
Chromatographic separation of phenolic compounds
The chromatographic separation was carried out using gradient elution of acetonitrile (ACN) and 1% (v/v) acetic acid with a flow rate of 0.8 mL min−1. The gradient condition was performed as follow: 7% ACN (initial step), ramped to 15% ACN (0–10 min), ramped to 35% ACN (10–15 min), and then ramped to 55% ACN (15–20 min). After that, ramped to 100% ACN (20–25 min) and was held for 15 min for washing the column. The chromatograms were recorded at 280 nm. Using this condition, 12 studied phenolic compounds and 3-hydroxybenzaldehyde (internal standard, IS) were successfully separated within 25 min (see Fig. 1).
Typical chromatogram of standard phenolic compounds (3.0 mg L−1 each) obtained from CPE. Conditions: HPLC, Symmetry C18 column (3.9 mm × 150 mm), gradient elution of ACN and 1.0% (v/v) acetic acid (see in text) with the flow rate of 0.8 mL min−1, 30 °C and detection at 280 nm; CPE, 2.0% (w/v) Triton X-114, 3.0% (w/v) Na2SO4, 20 min equilibrated at 45 °C and centrifugation at 3,500 rpm for 20 min. Peak assignments: 1, gallic acid (GAL); 2, protocatechuic acid (PRO); 3, p-hydroxybenzoic acid (p-HYD); 4, vanillic acid (VAL); 5, caffeic acid (CAF); 6, syringic acid (SYR); 3-hydroxybenzaldehyde (internal standard, IS); 7, p-coumaric acid (p-COU); 8, ferulic acid (FER); 9, guaiacol (GUA); 10, p-cresol (p-CRE); 11, o-cresol (o-CRE) and 12, 3,5-xylenol (XYL)
Cloud-point extraction
Aliquot (10.00 mL) of standard diluted wine sample (1:10, v/v) was mixed with 3.0% (w/v) Na2SO4. After the addition of 2.0% (w/v) Triton X-114 solution, the solution was incubated at 45 °C for 20 min in a thermostated water bath and then centrifuged at 3,500 rpm for 10 min to complete the phase separation. The solution was then kept in an ice bath for 5 min. The aqueous phase (upper part) was withdrawn using a 10-mL syringe. The surfactant-rich phase (~500 μL) was then diluted with 300 μL methanol (50%, v/v) to decrease viscosity before subjecting to HPLC.
Wine samples
Thai local wine samples were purchased from local supermarket in Khon Kaen province, Northeastern Thailand. The local wines were produced from varieties of fruit in Northeastern region. The samples were protected against sunlight and stored at 4 °C until analyses. Samples were filtered through 0.45 μm membrane filters before analysis. Aliqout of samples (1.0 mL) and internal standard (150 μL) was added and then made up the volume to 10.0 mL with water and subsequently extracted by CPE.
Determination of total phenolic compounds and total flavonoids
Total phenolic contents (TPC) were determined by Folin-Ciocalteau method (Bonoli et al. 2004). Sample solution of 100 μL was introduced into a test tube and then 500 μL of Folin-Ciocalteu reagent and 6.0 mL of deionized water were added. After incubation for 2 min, 2 mL of 15% (w/v) Na2CO3 was added, left for 30 s and made volume to 10.0 mL with water. The absorbance was measured at 755 nm after incubation for 2 h. Gallic acid was used as chemical standard of calibration. The TPC was expressed as mg of gallic acid equivalents per liter of sample (mg GAE L−1).
Total flavonoids content was determined using a colorimetric method described previously (Sakanaka et al. 2005). One milliliter of sample was placed in a 10 mL volumetric flask containing 5 mL of deionized water. Then 0.3 mL of 5% (w/v) NaNO2 was added and, after 5 min, 0.3 mL of 10% (w/v) AlCl3 was also added. After 6 min, 2 mL of 1 mol L−1 NaOH was added and diluted with deionized water. The absorbance was measured immediately at 510 nm. The content of total flavonoids was calculated from the calibration curve of catechin standard, and expressed as mg of catechin equivalents per liter of sample (mg CE L−1).
Antioxidant activity determination
DPPH method (Brand-Williams et al. 1995; Chew et al. 2008; Vichapong et al. 2010) was slightly modified to determine free radical scavenging activity of phenolic compounds. Ascorbic acid was used as a standard for calibration and the antioxidant activity was calculated as mg ascorbic acid equivalents per liter of sample (mg AAE L−1). Aliquot 3.0 mL of 5.0 × 10−5 mol L−1 DPPH solution was added into the sample solution (1.0 mL). Subsequently, the mixture was kept for 30 min in the dark to allow the complete reaction, and measured the absorbance at 515 nm. Antioxidant activity of the sample was defined as the amount of antioxidant necessary to reduce the initial DPPH concentration by 50% (Efficient concentration = EC50 mg AAE L−1).
Statistical analysis
All analyses were carried out in replicates (n ≥ 3) and the results were reported as means±standard deviation (SD).
Results and discussion
Optimization of the CPE condition
Effect of salt type and concentrations
The addition of salts in CPE system can facilitate the separation of SRP from AQ phase (Santalad et al. 2009), and promote the efficiency of the extraction (Lopes et al. 2007). In this study, NaCl and Na2SO4 were tested firstly at 1.0% (w/v) and 2.0 (w/v) TX-114. It was found that Na2SO4 (ca. 0.70 mmol) gave higher peak area for the most analytes than that of NaCl (ca 1.71 mmol), Na2SO4 was then investigated in the concentration range of 0–5% (w/v). As in Fig. 2a, the results demonstate that the highest response (peak area) was obtained when Na2SO4 concentration was 3.0% (w/v). Afterward, some compounds including GUA, CAF, SYR, GAL acid, p-HYD, and VAL had peak area nearly constant while the other analytes had decreased peak area by 2–28%. However, when Na2SO4 concentration was higher than 5.0% (w/v), the SRP moved to the middle of solution, and the SRP was present at the surface of the solution when the salt concentration higher than 7.0% (w/v). These are difficult to handle SRP. Therefore, Na2SO4 at 3.0% (w/v) was selected.
Effect of the studied CPE parameters on response of the studied phenolics: a, Na2SO4; b, Triton X-114; and c, Temperature. Each experiment was performed in triplicate (n = 3) and expressed as mean±SD. The other parameters are described in Fig. 1
Effect of concentration of Triton X-114
The amount of Triton X-114 was studied because it affects the extraction efficiency (Wei et al. 2008). Triton X-114 in the range 1.0–2.5% (w/v) was studied. Peak area gradually increased with an increase in Triton X-114 concentration up to 2.0% (w/v) and decreased the most afterward (see Fig. 2b). It is due to the increase in the volume of SRP that make dilution of the target analytes in the final solution. In this study, at 2.0% (w/v) Triton X-114 was chosen for further studies.
Effect of equilibrium temperature and incubation time
Although CPE based on non-ionic Triton X-114 surfactant can be performed at around 25 °C (cloud-point temperature of Triton X-114 is 23 °C) (Santalad et al. 2009). Theoretically, the optimal equilibration temperature for the extraction occurs when temperature is 15–20 °C higher than the cloud-point of surfactant (Han et al. 2008; Wang et al. 2006). In this study, temperature was studied in the range of 35–55 °C (see in Fig. 2c). Peak area for most studied phenolics increased as the temperature was increased up to 45 °C. Beyond this point, the signals remained quite constant and gradually increased for p-CRE, FER, o-CRE, XYL, GUA, and internal standard (less than 12% increments). Therefore, 45 °C was selected.
The effect of incubation time (5–30 min) at 45 °C was also investigated (data not shown), the highest value reached when extracted for 20 min. When the extraction time was longer than 20 min, the peak area of the studied phenolics decreased.
Analytical validations of CPE and HPLC
The calibration graphs were constructed by plotting concentration of the phenolic compounds (mg L−1) against the ratio of peak area of each phenol and internal standard. The results are listed in Table 1. Accuracy was evaluated via the study of recovery of the spiked sample. The precision of the method was demonstrated by replicate analyses (n = 5) for intra-day and inter-day (n = 3 × 5 days) and calculating the relative standard deviation (RSD) of peak area and retention time (tR). Good precisions (intra-day and inter-day) of the method with RSD lower than 5% (tR) and 10% (peak area) were obtained (see Table 1).
The sensitivity was also evaluated in terms of LOD as concentration giving the signal-to-noise ratio of 3 (S/N = 3). To compare the sensitivity of the CPE and HPLC method, enrichment factor (EF) is defined as the ratio of slope obtained from CPE-HPLC and HPLC (direct injection). The EF was obtained to be ca. 1.0 (GAL, VAL, and SYR), ca. 1.5–2.0 (PRO, p-HYD, and CAF), ca. 5 (p-COU and GAU), and ca. 10–15 (FER, p-CRE, o-CRE, and XYL). It is indicated that the method based on CPE gave higher sensitivity than direct injection analysis.
Recovery was studied by spiking standard phenolic compounds at three different concentration levels of phenolic compounds (0.5, 1.0, and 2.0 mg L−1) into wine sample and then extracted by CPE. The surfactant-rich phase was analyzed by HPLC-UV. The results showed that the proposed method gave good percentage recoveries in the range of 90.4–110% for most phenolics studied in wine samples (Table 2).
The amount of phenolic compounds in wine samples
The identification of the studied compounds was performed by comparison of both retention time and their absorption spectra with those obtained from the standard phenolic compounds. Figure 3 shows the typical chromatogram of original wine (sample blank) and spiked standard phenolic compounds (2.0 mg L−1 each) of Grape wine sample. The concentrations of phenolic compounds obtained in all studied samples are summarized in Table 3. GAL, PRO, and VAL were the major phenolic compounds found in the studied samples. GAL was found in the range 0.4–172.4 mg L−1, whereas PRO and VAL were found in range 0.2–99.1 and 0.1–26.6 mg L−1, respectively. The rest phenolic compounds studied were found between not detected and 8.8 mg L−1. GAL was the highest content of phenolic found in the most of studied samples. Jambolan wine had the highest GAL of 172.4 mg L−1, followed by Mao wine (35.4 mg L−1). Makhampom wine had PRO with the content up to 99.1 mg L−1, while VAL was detected at high value in Mangosteen and Makhampom wines with the average concentration of 26.6 and 20.2 mg L−1. The composition and concentration of phenolics in wines depend on the variety of raw materials, wine-making process and chemical reactions that occur during the aging of wines (Czyzowska and Pogorzelski 2002; Peña-Neira et al. 2000). These results are in good agreement with the previous reports (Woraratphoka et al. 2007; Minussi et al. 2003).
Chromatograms of grape wine sample obtained by CPE: a represents of original sample, b represents of spiked original sample with 2.0 mg L−1 each phenolic. The conditions of HPLC and CPE are described in Fig. 1
Total phenolic compounds, total flavonoids, and antioxidant activity
Table 4 summarizes total phenolic compound as gallic acid equivalent (GAE), total flavonoid content (TFC) as catechin equivalent (CTE) and antioxidant activity as EC50 of ascorbic acid equivalent (AAE). The results show no correlation between TPC, TFC and antioxidant activity in the studied wine samples. For examples, Rossele wine contains lower both TPC and TFC than that of Grape wine, but gave more antioxidant activity (DPPH). The possible explanations of the above results include, (i) the influence of the different flavonoid and non-flavonoid subgroups on the antioxidant activity, (ii) the degree of polymerization and the ratio between monomeric and polymeric forms, (iii) the possible synergy or antagonism among the different classes of polyphenols, and (iv) the radical molecules contained in wines (Majo et al. 2008). However, high antioxidant activity was also observed in all studied wines and TPC and TFC are found in the ranges with the other reports as given in Table 4.
Conclusion
This work demonstrates the application of CPE for sample preparation and preconcentration of phenolic compounds in wine samples before their analysis by HPLC. CPE in combination with HPLC is a simple, accurate and sensitive method, and less consumption of samples. The individual phenolic compound, total phenolic and total flavonoid contents found in Thai local wines studied showed significant differences among the varieties of local fruits-derived wine products. The antioxidant activity (EC50 up to 8,506 mg AAE L−1) of the studied samples indicated that wines were as a source of antioxidants in foods.
References
Abad-García B, Berrueta LA, López-Márquez DM, Crespo-Ferrer I, Gallo B, Vicente F (2007) Optimization and validation of a methodology based on solvent extraction and liquid chromatography for the simultaneous determination of several polyphenolic families in fruit juices. J Chromatogr A 1154:87–96
Alén-Ruiz F, Pérez-Gregorio MR, Martínez-Carballo E, García-Falcón MS, Simal-Gándara J (2008) Influence of polyphenols on colour and antioxidant value in plant foods. EJEAFChe 7:3171–3176
Alén-Ruiz F, García-Falcón MS, Pérez-Lamela MC, Martínez-Carballo E, Simal-Gándara J (2009) Influence of major polyphenols on antioxidant activity in Mencía and Brancellao red wines. Food Chem 113:53–60
Balasundram N, Sundram K, Samman S (2006) Phenolic compounds in plants and agro-industrial by-products: antioxidant activity, occurrence, and potential uses. Food Chem 99:191–203
Bonoli M, Verardo V, Marconi E, Caboni MF (2004) Antioxidant phenols in barley (Hordeum vulgare L.) flour: comparative spectrophotometric study among extraction methods of free and bound phenolic compounds. J Agric Food Chem 52:5195–5200
Brand-Williams W, Cuvelier M-E, Berset C (1995) Use of a free radical method to evaluate antioxidant activity. Lebensm Wiss Technol 28:25–30
Chen H, Zhang Y, Lu X, Qu Z (2011) Comparative studies on the physicochemical and antioxidant properties of different tea extracts. J Food Sci Technol. doi:10.1007/s13197-011-0291-6
Chew YL, Lima YY, Omar M, Khoo KS (2008) Antioxidant activity of three edible seaweeds from two areas in South East Asia. LWT 41:1067–1072
Czyzowska A, Pogorzelski E (2002) Changes to polyphenols in the process of production of must and wines from blackcurrants and cherries. Part I. Total polyphenols and phenolic acids. Eur Food Res Technol 214:148–154
Dama CL, Kumar S, Mishra BK, Shukla KB, Mathur S, Doshi A (2010) Antioxidative enzymatic profile of mushrooms stored at low temperature. J Food Sci Technol 47(6):650–655
Francisco MLL, Reaurreccion AVA (2009) Development of a reversed-phase high performance liquid chromatography (RP-HPLC) procedure for the simultaneous determination of phenolic compounds in peanut skin extracts. Food Chem 117:356–363
García AA, Grande BC, Gàndara JS (2004) Development of a rapid method based on solid-phase extraction and liquid chromatography with ultraviolet absorbance detection for the determination of polyphenols in alcohol-free beers. J Chromatogr A 1054:175–180
García-Falcón MS, Pérez-Lamella C, Martínez-Carballo E, Simal-Gándara J (2007) Determination of phenolic compounds in wines: influence of bottle storage of young red wines on their evolution. Food Chem 105:248–259
Hamauzu Y, Irie M, Kondo M, Fujita T (2008) Antiulcerative properties of crude polyphenols and juice of apple, and Chinese quince extracts. Food Chem 108:488–495
Han F, Yin R, Shi X, Jia Q, Liu H, Yao H, Xu L, Li S (2008) Cloud point extraction-HPLC method for determination and pharmacokinetic study of flurbiprofen in rat plasma after oral and transdermal administration. J Chromatogr B 868:64–69
La Torre GL, Saitta M, Vilasi F, Pellicanò T, Dugo G (2006) Direct determination of phenolic compounds in Sicilian wines by liquid chromatography with PDA and MS detection. Food Chem 94:640–650
Li H, Wang X, Li Y, Li P, Wang H (2009) Polyphenolic compounds and antioxidant properties of selected China wines. Food Chem 112:454–460
Loganayaki N, Siddhuraju P, Manian S (2011) Antioxidant activity and free radical scavenging capacity of phenolic extracts from Helicteres isora L. and Ceiba pentandra L. J Food Sci Technol. doi:10.1007/s13197-011-0389-x
Lopes AS, Garcia JS, Catharino RR, Santos LS, Eberlin MN, Arruda MAZ (2007) Cloud point extraction applied to casein proteins of cow milk and their identification by mass spectrometry. Anal Chim Acta 590:166–172
Majo DD, Guardia ML, Giammanco S, Neve LL, Giammanco M (2008) The antioxidant capacity of red wine in relationship with its polyphenolic constituents. Food Chem 111:45–49
Malovaná S, Montelongo FJG, Pérez JP, Rodríguez-Delgado MA (2001) Optimisation of sample preparation for the determination of trans-resveratrol and other polyphenolic compounds in wines by high performance liquid chromatography. Anal Chim Acta 428:245–253
Minussi RC, Rossi M, Bologna L, Cordi L, Rotilio D, Pastore GM, Durán N (2003) Phenolic compounds and total antioxidant potential of commercial wines. Food Chem 82:409–416
Nave F, Cabrita MJ, da Costa CT (2007) Use of solid-supported liquid–liquid extraction in the analysis of polyphenols in wine. J Chromatogr A1169:23–30
Nuengchamnong N, Ingkaninan K (2009) On-line HPLC–MS–DPPH assay for the analysis of phenolic antioxidant compounds in fruit wine: Antidesma thwaitesianum Muell. Food Chem 118:147–152
Peña-Neira A, Hernández T, García-Vallejo C, Estrella I, Suarez JA (2000) A survey of phenolic compounds in Spanish wines of different geographical origin. Eur Food Res Technol 210:445–448
Pérez-Gregorio MR, Regueiro J, Alonso-González E, Pastrana-Castro LM, Simal-Gándara J (2011) Influence of alcoholic fermentation process on antioxidant activity and phenolic levels from mulberries (Morus nigra L.). LWT-Food Sci Technol 44:1793–1801
Pérez-Lamela C, García-Falcón MS, Simal-Gándara J, Orriols-Fernández I (2007) Influence of grape variety, vine system and enological treatments on the colour stability of young red wines. Food Chem 101:601–606
Prasad KN, Yang B, Yang S, Chen Y, Zhao M, Ashraf M, Jiang Y (2009) Identification of phenolic compounds and appraisal of antioxidant and antityrosinase activities from litchi (Litchi sinensis Sonn.) seeds. Food Chem 116:1–7
Pupponen-Pimiä R, Nohynek L, Meier C, Kähkönen M, Heinonen M, Hopia A, Oksman-Caldentey K-M (2001) Antimicrobial properties of phenolic compounds from berries. J Appl Microbiol 90:494–507
Rekha CR, Vijayalakshmi G (2010) Infl uence of natural coagulants on isofl avones and antioxidant activity of tofu. J Food Sci Technol 47(4):387–393
Rodrigues AS, Pérez-Gregorio MR, García-Falcón MS, Simal-Gándara J, Almeida DPF (2011) Effect of meteorological conditions on antioxidant flavonoids in Portuguese cultivars of white and red onions. Food Chem 124:303–308
Rodríguez-Delgado MA, Malovaná S, Pérez JP, Borgesa T, García Montelongo FJ (2001) Separation of phenolic compounds by high-performance liquid chromatography with absorbance and fluorimetric detection. J Chromatogr A 912:249–257
Roussis IG, Lambropoulos I, Tzimas P, Gkoulioti A, Marinos V, Tsoupeis D, Boutaris I (2008) Antioxidant activities of some Greek wines and wine phenolic extracts. J Food Compos Anal 21:614–621
Rupasinghe HPV, Clegg S (2007) Total antioxidant capacity, total phenolic content, mineral elements, and histamine concentrations in wines of different fruit sources. J Food Compos Anal 20:133–137
Sachindra NM, Airanthi MKWA, Hosokawa M, Miyashita K (2010) Radical scavenging and singlet oxygen quenching activity of extracts from Indian seaweeds. J Food Sci Technol 47:94–99
Sakanaka S, Tachibana Y, Okada Y (2005) Preparation and antioxidant properties of extracts of Japanese persimmon leaf tea (kakinoha-cha). Food Chem 89:569–575
Santalad A, Srijaranai S, Burakham R, Glennon JD, Deming RL (2009) Cloud-point extraction and reversed-phase high-performance liquid chromatography for the determination of carbamate insecticide residues in fruits. Anal Bioanal Chem 394:1307–1317
Santana CM, Ferrera ZS, Rodriguez JJS (2002) Use of non-ionic surfactant solutions for the extraction and preconcentration of phenolic compounds in water prior to their HPLC-UV detection. Analyst 127:1031–1037
Sharma KD, Stähler K, Smith B, Melton L (2011) Antioxidant capacity, polyphenolics and pigments of broccoli-cheese powder blends. J Food Sci Technol 48(4):510–514
Stratil P, Klejdus B, Kubáň V (2007) Determination of phenolic compounds and their antioxidant activity in fruits and cereals. Talanta 71:1741–1751
Vichapong J, Sookserm M, Srijesdaruk V, Swatsitang P, Srijaranai S (2010) High performance liquid chromatographic analysis of phenolic compounds and their antioxidant activities in rice varieties. LWT-Food Sci Technol 43:1325–1330
Wang L, Cai Y-Q, He B, Yuan CG, Shen D-Z, Sha J, Jiang G-B (2006) Determination of estrogens in water by HPLC–UV using cloud point extraction. Talanta 70:47–51
Wei W, Yin X-B, He X-W (2008) pH-mediated dual-cloud point extraction as a preconcentration and clean-up technique for capillary electrophoresis determination of phenol and m-nitrophenol. J Chromatogr A 1202:212–215
Woraratphoka J, Intarapichet K, Indrapichate K (2007) Phenolic compounds and antioxidative properties of selected wines from the northeast of Thailand. Food Chem 104:1485–1490
Wu J-H, Huang C-Y, Tung Y-T, Chang S-T (2008) Online RP-HPLC-DPPH screening method for detection of radical-scavenging phytochemicals from flowers of Acacia confuse. J Agric Food Chem 56:328–332
Zhou J, Chen J, Cheng Y, Li D, Hu F, Li H (2009) Determination of Prometryne in water and soil by HPLC–UV using cloud-point extraction. Talanta 79:189–193
Acknowledgements
Financial support from the Center for Innovation in Chemistry (PERCH-CIC), Commission on Higher Education, Ministry of Education is gratefully acknowledged.
Author information
Authors and Affiliations
Corresponding author
Rights and permissions
About this article
Cite this article
Vichapong, J., Santaladchaiyakit, Y., Burakham, R. et al. Cloud-point extraction and reversed-phase high performance liquid chromatography for analysis of phenolic compounds and their antioxidant activity in Thai local wines. J Food Sci Technol 51, 664–672 (2014). https://doi.org/10.1007/s13197-011-0556-0
Revised:
Accepted:
Published:
Issue Date:
DOI: https://doi.org/10.1007/s13197-011-0556-0