Abstract
Our previous study has shown that acteoside, an antioxidative phenylethanoid glycoside, protect against beta-amyloid (Aβ)-induced cytotoxicity in vitro. However, the precise protective mechanisms remains unclear. Heme oxygenase-1 (HO-1) is a crucial factor in the response to oxidative injury, protecting neurons against Aβ-induced injury. In the present study we examined to determine whether acteoside upregulates HO-1 expression, and thereby protects PC12 cells against Aβ-induced cell death. It was revealed that acteoside is an activator of Nrf2 and inducer of HO-1 expression. We showed that acteoside increased HO-1 expression in vitro and in vivo. Acteoside treatment resulted in nuclear translocation of the transcription factor NF-E2-related factor 2 (Nrf2). Acteoside activated both ERK and PI3 K/Akt, and treatments with the specific ERK inhibitor PD98059, the PI3 K inhibitor LY294002, and the specific Nrf2 siRNA suppressed the acteoside-induced HO-1 expression. The HO-1 inhibitor ZnPP, PD98059, and LY294002 markedly abolished the neuroprotective effect of acteoside against Aβ-induced neurotoxicity. Taken together, these results demonstrate that acteoside is an activator of Nrf2 and inducer of HO-1 expression. We also showed that acteoside increased HO-1 expression through activation of ERK and PI3 K/Akt signal pathways in vitro. Upregulation of HO-1 by acteoside may involve in the neuroprotection against Aβ-induced neurotoxicity.
Similar content being viewed by others
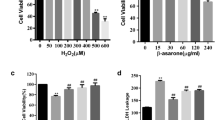
Avoid common mistakes on your manuscript.
Introduction
There is considerable evidence to support oxidative stress as a common pathogenetic mechanism in Alzheimer’s disease (AD) (Markesbery 1997; Reddy 2006). Oxidative damage occurs early in disease (Nunomura et al. 2001), suggesting that oxidative stress plays a role in disease progression. In AD, oxidative stress is suspected to be generated by the beta amyloid peptide (Aβ) (Behl et al. 1994), oxidative stress promotes the production of Aβ. Recent studies showed that there is a vicious circle among Aβ production/accumulation and oxidative stress (Shen et al. 2008; Tamagno et al. 2008). Given the important role of oxidative stress in AD, therapeutic strategies which are directed at early interventions targeted at oxidative stress may be effective in delaying AD development and slowing its progression. Indeed, increased antioxidant activity confers protection and has been reported to lower the risk of AD (Engelhart et al. 2002). Thus, an approach which simultaneously induces various intracellular defence mechanisms against oxidative injury may be more effective in combating neurodegeneration.
One way to render neuronal cells more resistant to oxidative stress is to up-regulate the endogenous protection system, which cells respond to oxidative stress is the antioxidant response element (ARE) pathway. Cells have developed complex mechanisms to defend from oxidative stress. A battery of genes encoding detoxifying and antioxidative enzymes is orchestrated on exposure to electrophiles and reactive oxygen species (Itoh et al. 1997; Motohashi and Yamamoto 2004). This coordinated response is regulated through the binding of nuclear factor E2-related factor 2 (Nrf2) to the ARE within the regulatory region of target genes. Nrf2 regulates the co-ordinated expression of cytoprotective genes (Moi et al. 1994), which includes heme oxygenase-1 (HO-1) among other enzymes.
HO-1 is the inducible form of HO that catalyzes the rate-limiting step in the conversion of heme into biliverdin, carbon monoxide, and free iron. HO-1 is a crucial factor in the response to oxidative injury. Several evidences suggest that HO-1 has neuroprotective effects against oxidative stress-induced neuronal damage (Ahmad et al. 2006; Ryter et al. 2006). HO-1 appears to be involved in protection against oxidative stress (Takahashi et al. 2004).
Particularly interesting is the role played by HO-1 in AD, a neurodegenerative disorder that associates with both oxidative brain injury and Aβ-associated pathology. Significant increases in the levels of HO-1 have been observed in AD brains in association with neurofibrillary tangles, and HO-1 mRNA was found increased in AD neocortex and cerebral vessels; the HO-1 increase also colocalized with senile plaques and glial fibrillary acidic protein-positive astrocytes in AD brains (Schipper 2000). Previous findings support the importance of HO-1, a crucial factor in the response to oxidative injury, in protecting neurons against Aβ-induced oxidative stress dependant injury (Le et al. 1999; Ma et al. 2010; Tanaka et al. 2010; Wruck et al. 2008). The protective role played by HO-1 and its products in AD raised new possibilities regarding the possible use of natural substances, which are able to increase HO-1 levels, as potential drugs for the prevention and treatment of AD. Indeed, our previous study shed light on astaxanthin, the most abundant flavonoids in propolis, protecting against beta-amyloid-induced cytotoxicity by upregulating HO-1 expression through ERK1/2 pathway.
Thus, in light of the cytoprotective role of HO-1, the specific activation of HO-1 gene expression by pharmacological modulation may represent a novel target for therapeutic treatment of AD. There is, therefore, an excellent rationale for the development of new neuroprotective agents, based on their ability to enhance activity of Nrf2 and upregulate HO-1 expression.
Acteoside (AS), an antioxidative phenylethanoid glycoside, was first extracted from Verbascum sinuatum and named “verbascoside.” Our previous studies has shown that it can protect against beta-amyloid-induced cytotoxicity in human neuroblastoma SH-SY5Y cells (Wang et al. 2009a). However, the precise protective mechanisms remain unclear, and remains as an interesting speculation that awaits further investigation. The present study therefore examined to determine whether acteoside upregulates HO-1 expression, and thereby protects against Aβ-induced oxidative cell death through induction of HO-1 expression in PC12 cells incubated with Aβ25–35. Although there exist several cell culture systems that were intensively studied on amyloid beta toxicity (Calkins et al. 2011; Calkins and Reddy 2011; Manczak et al. 2010), we used PC12 neuronal model system. PC12 cells are more sensitive to Aβ insult and have been used extensively to study Aβ neurotoxicity.
In the study reported here, we have shown that the acteoside is an activator of Nrf2 and inducer of HO-1 expression. We also showed that acteoside increased HO-1 expression through activation of ERK and PI3 K/Akt signal pathways in vitro, and that the increased HO-1 activity induced by acteoside is responsible for the cytoprotective effects against the Aβ25–35-induced neurotoxicity.
Materials and Methods
Acteoside was obtained from Winherb Medical Technology Inc (Shanghai, China). The stock solution of acteoside was made with distilled H2O and stored at 4°C. Nuclear and Cytoplasmic Protein Extraction Kit (#P0027) was from Beyotime (Jiangsu, China). The stock solution was diluted to working concentrations before use. 3-(4,5-dimethylthiazol-2-yl)-2,5-diphenyltetrazolium bromide (MTT) was purchased from Sigma-Aldrich Inc. Aβ25–35 was obtained from GL Biochem Ltd. (Shanghai, China). 4′,6-diamino-2′-phenylindole dihydrochloride (DAPI), and Complete Protease Inhibitor were from Roche Diagnostics GmbH (Penzberg, Germany). Modified Dulbecco’s Eagle’s medium (DMEM) supplement was obtained from Gibco Invitrogen Corporation. PD98059(SC-3532), LY290042 (SC-201462) and ZnPP (SC-200329) were from Santa Cruz. Rabbit anti-phospho-p38 (Thr180/Tyr182) (#9211), anti-p38 (#9212), rabbit anti-phospho-ERK1/2 (Thr202/Tyr204) (#9101) and ERK (#9102), rabbit anti-phospho-Akt (Ser473) (#9271), and rabbit anti-Akt (#9272) antibodies were obtained from Cell Signaling Technology Inc. (Danvers, MA, USA). Rabbit anti-Lamin B1 (ab16048) and anti-Nrf2 (ab31163) were from Abcam. HO-1 antibody was from Stressgen (#SPA895, Victoria, BC, Canada). Horseradish peroxidase-linked IgG antibodies were obtained from Invitrogen. All the other chemicals used were of the high grade available commercially.
Cell Culture and Treatment
PC12 cells were maintained in DMEM supplemented with 10% heat-inactivated horse serum and 5% fetal bovine serum and cultured at 37°C in a humidified atmosphere of 5% CO2 as described previously (Kim et al. 2008; Li et al. 2007). All cells were cultured in poly-d-lysine coated culture dishes. The medium was changed every other day, and the cells were plated at an appropriate density according to the scale of each experiment.
Determination of Cell Viability
Cell viability was assessed using conventional MTT reduction assay as described previously (Kim et al. 2008; Li et al. 2007). After incubation with various chemicals, the cells were treated with MTT solution (1 mg/ml final concentration) for 2 h at 37°C. The dark blue formazan crystals formed inside the intact mitochondria were solubilized with dimethylsulfoxide, and the absorbance of blue color was measured at 570 nm using a microplate reader.
Preparation of Nuclear Extract
The extraction and isolation of nuclear and cytoplasmic protein were performed according to the Nuclear and Cytoplasmic Protein Extraction Kit (Beyotime, Jiangsu, China) as described previously (Wang et al. 2009b).
Animals and Treatments
Male Sprague–Dawley rats (220–250 g) were purchased from the Shanghai Experimental Animal Center of the Chinese Academy of Science, and maintained in a temperature-controlled room (22–25°C). All animal experiments were performed according to the Guide for the Care and Use of Medical Laboratory Animals (Ministry of Health, PR China, 1998) and the guidelines of the Shanghai Medical Laboratory Animal Care and Use Committee. All rats were habituated to the colony and food and water were supplied ad libitum. For immunohistochemical analyses, rats were treated with a single injection for 3, 6 and 12 h with 25 mg/kg body weight acteoside dissolved in saline or saline as a vehicle control, each given intraperitoneally (i.p.). Animals were euthanized 3 h after the last injection. The procedures were carried out according to previous study (Wang et al. 2003; Yan et al. 2010). Rats were anesthetized with sodium pentobarbital and perfused with 0.9% saline solution followed by 4% icecold phosphate-buffered paraformaldehyde (PA). The brains were removed and post-fixed for 4–6 h in the same solution of paraformaldehyde at 4°C, and then cryoprotected by immersion overnight at 4°C in 4% paraformaldehyde containing 20% sucrose, then immersed in 0.1 M PB containing 30% sucrose until sunk. Brain sections were cut on a freezing microtome (Jung Histocut, Model 820-II, Leica, Germany) at a thickness of 30 μm, and stored at −20°C in cryoprotectant solution. For HO-1 staining, sections were incubated with the following primary antibodies: rabbit HO-1 antibody (1:200 dilution). The sections were washed and incubated with corresponding biotinylated secondary antibodies (1:200 dilution) for 1 h at 37°C. Subsequently, they were incubated with 1:200 avidin–biotin–peroxidase for 45 min at 37°C, stained with 0.05% diaminobenzidine (DAB) in the presence of 0.03% H2O2. Controls were performed by omitting primary antibody, and showed no positive staining. For immunoblot analyses, Fresh brain tissue was homogenized in lysis buffer (50 mM Tris–HCl, pH 6.8, 2% SDS, 10% glycerol, 2 mM EDTA, 1 mM alpha-phenylmethanesulfonyl fluoride). The HO-1 levels were determined using a polyclonal antibody to HO-1 by Western blotting as described below.
Western Blot Analysis
Following treatment, cells were washed and collected with PBS. After centrifugation, cell lysis was carried out at 4°C by vigorous shaking for 15 min in RIPA buffer (150 mM NaCl, 1% NP-40, 0.5% sodium deoxycholate, 0.1% SDS, 50 mM Tris–HCl (pH 7.4), 50 mM β-glycerol phosphate, 20 mM NaF, 20 mM EGTA, 1 mM DTT, 1 mM Na3VO4, and protease inhibitors). After centrifugation at 15,000 rpm for 15 min, supernatant was separated and stored at −70°C until use. The protein concentration was determined using the Bradford method, and the lysates were boiled for 5 min. Denatured proteins were separated using sodium dodecyl sulfate polyacrylamide gel electrophoresis on a 8 or 10% polyacrylamide gel and then transferred onto PVDF (Millipore, Billerica, MA, USA). After blocking overnight at 4°C in 5% BSA in Tris-buffered saline/Tween [with 0.05% (v/v) Tween 20], the membranes were first incubated with each antibody at dilutions of 1:2000. The second incubation was performed with horseradish peroxidase-conjugated secondary anti-rabbit IgG antibody. To monitor potential artifacts in loading and transfer among samples in different lanes, the blots for phospho-MAPK were stripped and reprobed with antibodies against total MAPK. The blots were developed using an ECL Western blotting detection reagent (Santa Cruz).
Statistical Analysis
All data were presented as the mean ± SEM. Data were subjected to statistical analysis via one-way ANOVA followed by student’s t-test with GraphPad Prism 4.0 software (GraphPad Software, Inc., San Diego, CA). Mean values were considered to be statistically significant at P < 0.05.
Results
Acteoside Induces HO-1 Protein Expression in Vitro and in Vivo
We first investigated the possibility that acteoside might alter the expression of the antioxidant enzyme HO-1. Treatments of the PC12 cells with acteoside resulted in a time-dependent (Fig. 1a) and concentration-dependent (Fig. 1b) increase in HO-1 protein expression (Fig. 1a). HO-1 has been localized to both the cytosol (Calkins et al. 2005, 2010), as well as the nucleus (Leonard et al. 2006). To determine the subcellular localization of HO-1 in PC12 cells, cells were treated with acteoside for 24 h and HO-1 expression examined by confocal immunofluorescence. Cells that were untreated showed low fluorescence, treatment with acteoside induced HO-1 which was predominantly localized to the cytoplasm (Fig. 1c). In vivo studies showed that acteoside induces HO-1 protein expression in SD rat were treated with acteoside (Fig. 2a, b). Taken together, acteoside can induce HO-1 protein expression in vitro and in vivo.
Acteoside induces HO-1 protein expression in vitro. PC12 cells were incubated with a 30 μM acteoside for the indicated amounts of time and b various concentrations of acteoside for 12 h. c PC12 cells were treated with 30 μM acteoside for 12 h, and was examined by immunochemical staining of HO-1 and confocal microscopic analysis. Cells were stained with an anti-HO-1 antibody (left) and nuclei with DAPI (middle). * P < 0.05; ** P < 0.01
Acteoside induces HO-1 protein expression in vivo. Male Sprague–Dawley rats (200–250 g) were treated with 25 mg/kg acteoside (ip) and euthanized at indicated time. a HO-1 immunostaining, using mouse monoclonal antibody to HO-1 and 30-μm-thick sections, was carried out as described in “Materials and Methods” section. Magnification: 5×. b HO-1 protein of cortical neuron was analyzed by Western blot
Acteoside Activates Nrf2 Transcription Factor
Most of the genes encoding phase II detoxifying and antioxidant enzymes have an ARE sequence in their promoter region. Nrf2 is an important transcription factor that regulates ARE-driven HO-1 gene expression. We analyzed to determine whether acteoside is able to activate Nrf2 in association with HO-1 up-regulation. We analyzed for the Nrf2 accumulation in the nuclei in the presence of acteoside. The cells were treated with acteoside, and the level of Nrf2 protein was then determined by Western blotting. Acteoside induced the accumulation of Nrf2 in the nuclei relative to untreated cells (Fig. 3a, b). To verify the requirement of Nrf2 for acteoside-induced HO-1 expression, cells were transfected with siNrf2 for 24 h before acteoside treatment. As shown in Fig. 4b, the acteoside-induced expression of HO-1was markedly supressed by siRNA knock down of Nrf2 gene. Taken together, these findings support that acteoside activate Nrf2, and that the upregulation of HO-1 elicited by acteoside is mediated via the Nrf2 activation.
Acteoside stimulates activation of Nrf2 in PC12 cells. a PC12 cells were incubated with 30 μM acteoside for the indicated time. Nrf2 protein in the cytosolic and the nuclei were detected by Western blotting. b Translocation of Nrf2 to the nucleus by acteoside. PC12 cells were treated with 30 μM acteoside for 6 h, and the standard immunocytochemical method and confocal microscopic analysis was employed to determine the translocation of Nrf2 molecules. Cells were stained with an anti-Nrf2 antibody (left) and nuclei with DAPI (middle)
Nrf2 activation involves in acteoside-induced upregulation of HO-1. a PC12 cells were transfected with control siRNA (Ctrl siRNA) or Nrf2 siRNA B (siNrf2) for 24 h, and then cells were harvested to detect Nrf2 protein levels by Western blot. ** P < 0.01. b PC12 cells were transfected with Nrf2-siRNA (siNrf2) or Nrf2-negative control-siRNA (Ctrl siRNA) for 24 h. The transfected cells were treated with acteoside (30 μM) for 12 h and expression HO-1 was measured by Western blot analysis
Acteoside Upregulates HO-1 Expression Through ERK and PI3 K/Akt Pathways
To elucidate the plausible signal transduction pathways involved in the acteoside-induced HO-1 expression, we examined the phosphorylation of several upstream kinases. We examined the activation of ERK and Akt, both of which are major signaling enzymes involved in cellular protection against oxidative stress. As shown in Fig. 5a and b, exposure to acteoside caused an increase in the phosphorylation of ERK, and Akt in a time-dependent manner. However, the phosphorylated forms of JNK and p38 were not detected in acteoside-treated cells (data not shown).
Acteoside activates the ERK and the PI3 K/Akt pathways. PC12 cells were stimulated with 30 μM acteoside for the indicated times. Cell lysates were electrophoresed and then immunoblotted with activation-specific antibodies that recognize a phospho-ERK (pERK1/2), then the blots for pERK1/2 were stripped and reprobed with antibodies against total ERK, and b phospho-Akt (pAkt), then the blots for pAkt were stripped and reprobed with antibodies against total Akt
To identify the signaling pathways used by acteoside to induce HO-1 expression, we then analyzed to determine whether ERK and Akt pathways were involved in the induction of HO-1 expression. We examined the effects of the specific ERK inhibitor PD98059, and the PI3 K inhibitor LY294002 on acteoside-induced HO-1 expression. We found that the HO-1 expression was blocked by PD98059 and LY294002 (Fig. 6).
Involvement of ERK and the PI3 K/Akt pathways on HO-1 production induced by acteoside (AS). a–c PC12 cells were preincubated with PD98059 (PD) or/and LY294002 (LY) for the indicated dose for 1 h and further incubated for 6 h after the addition of AS (30 μM). Cell lysates were electrophoresed and then immunoblotted with activation-specific antibodies that recognize a phospho-ERK (pERK1/2) and total ERK, and b phospho-Akt (pAkt) and total Akt, or c HO-1 and β-actin. * P < 0.05; ** P < 0.01
Acteoside Protect Against Aβ-Induced Neurotoxicity by Upregulating HO-1 Expression
It has been demonstrated that upregulation of HO-1 expression and elevated HO activity play a key role in protecting cells against the toxicity caused by a variety of oxidative insults. Previous findings support the importance of HO-1 in protecting neurons against Aβ-induced oxidative stress-dependant injury (Le et al. 1999; Ma et al. 2010; Tanaka et al. 2010; Wruck et al. 2008). In an attempt to determine whether the increased HO-1 expression induced by acteoside is indeed responsible for the cytoprotective effects against the Aβ25–35-derived oxidative cell death, we utilized ZnPP, an inhibitor of HO-1 activity. As illustrated in Fig. 7a, pretreatment of PC12 cells with ZnPP for 1 h before Aβ25–35 challenge attenuated the acteoside-mediated cytoprotection, but ZnPP alone does not result in cytotoxicity. These results suggest that the cytoprotective effect elicited by pretreatment with acteoside is mediated through induction of HO-1 expression. To determine whether activation of ERK and PI3 K/Akt could contribute to the acteoside-mediated protection against the cytotoxic effect of Aβ25–35, pharmacological inhibitors of these kinases, PD98059 and LY294002 were utilized. Acteoside-mediated cytoprotection against Aβ25–35-induced cytotoxicity was attenuated by PD98059 (Fig. 7b) and LY29400 (Fig. 7c). Taken together, these results indicated that kinases of the ERK and PI3 K/Akt pathway might be involved in acteoside-induced expression of HO-1.
Acteoside protects against Aβ-induced neurotoxicity by upregulating HO-1 expression. a HO-1 enzyme inhibitor ZnPP reversed the protective effect of acteoside against Aβ-induced cell death. PC12 cells were pretreated with a ZnPP, b PD98059 (PD) or c LY294002 (LY) for 1 h in the absence or presence of acteoside and were exposed to Aβ25–35 for 24 h. Cell viability was measured by MTT assay. Data represent the means ± SE of three independent experiments. ** P < 0.01; ## P < 0.01; # P < 0.05
Discussion
In this study, we have identified acteoside, an antioxidative phenylethanoid glycoside, as a new activator of the transcription factor Nrf2 (Nuclear factor erythroid 2 p45-related factor 2), and the inducer of HO-1 expression. One of the most salient features of this study is that acteoside activate Nrf2-mediated-HO-1 induction through ERK1/2 and PI3 K-Akt pathways, thereby protecting the PC12 cells from Aβ25–35-induced oxidative neurotoxicity.
In this study, we have revealed that the acteoside is an activator of Nrf2. Recently, It has been hypothesized that Nrf2-ARE activation is a novel neuroprotective pathway that confers resistance to a variety of oxidative stress-related, neurodegenerative insults (Johnson et al. 2008). Activation of Nrf2-ARE pathway has been studied in a variety of tissues and cell types using many chemical activators. For example, tert-butyl hydroquinone (tBHQ) was shown to have displayed cytoprotective efficacy against toxins in neuroblastoma cells and primary neuronal cultures systems (Kraft et al. 2004) in vitro and in vivo (Shih et al. 2003, 2005a, b). Recent finding provided a direct evidence that the Nrf2-ARE pathway may be involved in the neuropathology of AD, and that activation of this endogenous antioxidant pathway provides protection against the toxicity of Aβ peptide (Kanninen et al. 2008). We showed that acteoside efficiently increase the nuclear levels of the Nrf2 (Fig. 3a, b).The central sensor of intracellular oxidative stress is the cytosolic Keap1-Nrf2 complex. In response to oxidative stress, Nrf2 is released from Keap1 and transmits the stress signal to the nucleus for activation of distinct set of genes encoding phase II detoxifying enzymes as well as several stress responsive proteins including heme oxygenase-1 (HO-1) (Itoh et al. 1999).
Several evidences suggest that HO-1 has neuroprotective effects against oxidative stress-induced neuronal damage (Ahmad et al. 2006; Bae et al. 2010; Chen et al. 2000; Hung et al. 2008; Ryter et al. 2006). HO-1 can be highly up-regulated during the formation of neurofibrillary tangles in Alzheimer’s brain (Schipper et al. 1995). Particularly interesting is the role played by HO-1 in AD, a neurodegenerative disorder that is associated with both oxidative brain injury and Aβ-associated pathology (Calabrese et al. 2010). Significant increases in the levels of HO-1 have been observed in AD brains in association with neurofibrillary tangles, and HO-1 mRNA was found to be increased in AD neocortex and cerebral vessels; the HO-1 increase also colocalized with senile plaques and glial fibrillary acidic protein-positive astrocytes in AD brains (Schipper 2000). Previous findings support the importance of HO-1 factor in the response to oxidative injury, in protecting neurons against Aβ-induced oxidative stress-dependant injury (Le et al. 1999; Ma et al. 2010; Tanaka et al. 2010; Wang et al. 2010; Wruck et al. 2008).
The protective role played by HO-1 in AD raised new possibilities regarding the possible use of natural substances, which are able to increase HO-1 levels, as potential drugs for the prevention and treatment of AD. In light of this, are the very promising polyphenolic compounds that are contained in some herbs and spices, for example, curcumin. The present study has illustrated that acteoside induces HO-1 in vitro and in vivo. Treatments of the PC12 cells with acteoside resulted in an increase in HO-1 protein expression (Fig. 1a, b). The acteoside-induced HO-1 expression requires Nrf2 activation, because the acteoside-induced expression of HO-1 was markedly suppressed by siRNA knock down of Nrf2 gene (Fig. 4).
Many studies have suggested that ERK and PI3 K/Akt are part of a central pathway involved in Nrf2 activation and translocation for highly specialized protein synthesis including HO-1 (Andreadi et al. 2006; Calabrese et al. 2005; Martin et al. 2004). To identify the signaling pathways used by acteoside to activate Nrf2 and induce HO-1 expression, we tested to determine whether acteoside-induced expression of HO-1 occurs through a specific MAPK and PI3 K/Akt pathway. We found that acteoside activated the ERK MAPK cascade, and PI3 K/Akt, but did not activate JNK and p38MAPK (Fig. 5). In addition, the use of specific inhibitors for ERK1/2 and PI3 K/Akt pathways confirmed the involvement of these two pathways in acteoside-induced HO-1 expression (Fig. 6).
In accordance with the postulation that an elevation of HO-1 by various stimuli may be a protective cellular response to delay the cell death and HO-1 play a role in protecting neurons against Aβ-induced oxidative stress-dependent injury (Le et al. 1999; Ma et al. 2010; Tanaka et al. 2010; Wang et al. 2010; Wruck et al. 2008), we were interested in determining the potential role of HO-1 in the Aβ-induced PC12 cell damage and in the acteoside-mediated neuroprotection. We demonstrated that the protective effects against Aβ-induced cytotoxicity by acteoside were greatly reduced by ZnPP (Fig. 7a). These results suggest that acteoside-stimulated HO-1 may protect PC12 cells against the Aβ-induced cytotoxicity. To determine whether such activation of ERK and PI3 K/Akt could contribute to the acteoside-mediated protection against the cytotoxic effect of Aβ25–35, pharmacological inhibitors of these two kinases were utilized. Acteoside-mediated cytoprotection against Aβ25–35-induced cytotoxicity was attenuated by PD98059 (Fig. 7b) and LY294002 (Fig. 7c).
Taken together, these results demonstrate that acteoside is an activator of Nrf2 and inducer of HO-1 expression. Acteoside attenuates Aβ25–35-induced neurotoxicity by induction of HO-1 via ERK and PI3 K/Akt signaling. These results contribute to shed some light on the mechanisms whereby acteoside protects against beta-amyloid-induced cytotoxicity.
References
Ahmad A, Zhuang H, Doré S (2006) Heme oxygenase-1 protects brain from acute excitotoxicity. Neuroscience 141:1703–1708
Andreadi C, Howells L, Atherfold P, Manson M (2006) Involvement of Nrf2, p38, B-Raf, and nuclear factor-kappaB, but not phosphatidylinositol 3-kinase, in induction of hemeoxygenase-1 by dietary polyphenols. Mol Pharmacol 69:1033–1040
Bae JW, Kim MJ, Jang CG, Lee SY (2010) Protective effects of heme oxygenase-1 against MPP(+)-induced cytotoxicity in PC-12 cells. Neurol Sci 31:307–313
Behl C, Davis J, Lesley R, Schubert D (1994) Hydrogen peroxide mediates amyloid beta protein toxicity. Cell 77:817–827
Calabrese V, Ravagna A, Colombrita C, Scapagnini G, Guagliano E, Calvani M, Butterfield D, Giuffrida Stella A (2005) Acetylcarnitine induces heme oxygenase in rat astrocytes and protects against oxidative stress: involvement of the transcription factor Nrf2. J Neurosci Res 79:509–521
Calabrese V, Cornelius C, Dinkova-Kostova AT, Calabrese EJ, Mattson MP (2010) Cellular stress responses, the hormesis paradigm, and vitagenes: novel targets for therapeutic intervention in neurodegenerative disorders. Antioxid Redox Signal 13:1763–1811
Calkins MJ, Reddy PH (2011) Amyloid beta impairs mitochondrial anterograde transport and degenerates synapses in Alzheimer’s disease neurons. Biochim Biophys Acta 1812(4):507–513
Calkins MJ, Jakel RJ, Johnson DA, Chan K, Kan YW, Johnson JA (2005) Protection from mitochondrial complex II inhibition in vitro and in vivo by Nrf2-mediated transcription. Proc Natl Acad Sci USA 102:244–249
Calkins MJ, Vargas MR, Johnson DA, Johnson JA (2010) Astrocyte-specific overexpression of Nrf2 protects striatal neurons from mitochondrial complex II inhibition. Toxicol Sci 115:557–568
Calkins MJ, Manczak M, Mao P, Shirendeb U, Reddy PH (2011) Impaired mitochondrial biogenesis, defective axonal transport of mitochondria, abnormal mitochondrial dynamics and synaptic degeneration in a mouse model of Alzheimer’s disease. Hum Mol Genet 20(23):4515–4529
Chen K, Gunter K, Maines MD (2000) Neurons overexpressing heme oxygenase-1 resist oxidative stress-mediated cell death. J Neurochem 75:304–313
Engelhart MJ, Geerlings MI, Ruitenberg A, van Swieten JC, Hofman A, Witteman JC, Breteler MM (2002) Dietary intake of antioxidants and risk of Alzheimer disease. JAMA 287:3223–3229
Hung SY, Liou HC, Kang KH, Wu RM, Wen CC, Fu WM (2008) Overexpression of heme oxygenase-1 protects dopaminergic neurons against 1-methyl-4-phenylpyridinium-induced neurotoxicity. Mol Pharmacol 74:1564–1575
Itoh K, Chiba T, Takahashi S, Ishii T, Igarashi K, Katoh Y, Oyake T, Hayashi N, Satoh K, Hatayama I, Yamamoto M, Nabeshima Y (1997) An Nrf2/small Maf heterodimer mediates the induction of phase II detoxifying enzyme genes through antioxidant response elements. Biochem Biophys Res Commun 236:313–322
Itoh K, Ishii T, Wakabayashi N, Yamamoto M (1999) Regulatory mechanisms of cellular response to oxidative stress. Free Radic Res 31:319–324
Johnson JA, Johnson DA, Kraft AD, Calkins MJ, Jakel RJ, Vargas MR, Chen PC (2008) The Nrf2-ARE pathway: an indicator and modulator of oxidative stress in neurodegeneration. Ann N Y Acad Sci 1147:61–69
Kanninen K, Malm T, Jyrkkänen H, Goldsteins G, Keksa-Goldsteine V, Tanila H, Yamamoto M, Ylä-Herttuala S, Levonen A, Koistinaho J (2008) Nuclear factor erythroid 2-related factor 2 protects against beta amyloid. Mol Cell Neurosci 39:302–313
Kim J, Li M, Jang J, Na H, Song N, Lee C, Johnson J, Surh Y (2008) 15-Deoxy-delta(12,14)-prostaglandin J(2) rescues PC12 cells from H2O2-induced apoptosis through Nrf2-mediated upregulation of heme oxygenase-1: potential roles of Akt and ERK1/2. Biochem Pharmacol 76:1577–1589
Kraft AD, Johnson DA, Johnson JA (2004) Nuclear factor E2-related factor 2-dependent antioxidant response element activation by tert-butylhydroquinone and sulforaphane occurring preferentially in astrocytes conditions neurons against oxidative insult. J Neurosci 24:1101–1112
Le W, Xie W, Appel S (1999) Protective role of heme oxygenase-1 in oxidative stress-induced neuronal injury. J Neurosci Res 56:652–658
Leonard MO, Kieran NE, Howell K, Burne MJ, Varadarajan R, Dhakshinamoorthy S, Porter AG, O’Farrelly C, Rabb H, Taylor CT (2006) Reoxygenation-specific activation of the antioxidant transcription factor Nrf2 mediates cytoprotective gene expression in ischemia-reperfusion injury. FASEB J 20:2624–2626
Li HY, Zhong YF, Wu SY, Shi N (2007) NF-E2 related factor 2 activation and heme oxygenase-1 induction by tert-butylhydroquinone protect against deltamethrin-mediated oxidative stress in PC12 cells. Chem Res Toxicol 20:1242–1251
Ma W, Yuan L, Yu H, Ding B, Xi Y, Feng J, Xiao R (2010) Genistein as a neuroprotective antioxidant attenuates redox imbalance induced by beta-amyloid peptides 25–35 in PC12 cells. Int J Dev Neurosci 28:289–295
Manczak M, Mao P, Calkins MJ, Cornea A, Reddy AP, Murphy MP, Szeto HH, Park B, Reddy PH (2010) Mitochondria-targeted antioxidants protect against amyloid-beta toxicity in Alzheimer’s disease neurons. J Alzheimers Dis 20(Suppl 2):S609–S631
Markesbery W (1997) Oxidative stress hypothesis in Alzheimer’s disease. Free Radic Biol Med 23:134–147
Martin D, Rojo A, Salinas M, Diaz R, Gallardo G, Alam J, De Galarreta C, Cuadrado A (2004) Regulation of heme oxygenase-1 expression through the phosphatidylinositol 3-kinase/Akt pathway and the Nrf2 transcription factor in response to the antioxidant phytochemical carnosol. J Biol Chem 279:8919–8929
Moi P, Chan K, Asunis I, Cao A, Kan YW (1994) Isolation of NF-E2-related factor 2 (Nrf2), a NF-E2-like basic leucine zipper transcriptional activator that binds to the tandem NF-E2/AP1 repeat of the beta-globin locus control region. Proc Natl Acad Sci USA 91:9926–9930
Motohashi H, Yamamoto M (2004) Nrf2-Keap1 defines a physiologically important stress response mechanism. Trends Mol Med 10:549–557
Nunomura A, Perry G, Aliev G, Hirai K, Takeda A, Balraj EK, Jones PK, Ghanbari H, Wataya T, Shimohama S, Chiba S, Atwood CS, Petersen RB, Smith MA (2001) Oxidative damage is the earliest event in Alzheimer disease. J Neuropathol Exp Neurol 60:759–767
Reddy PH (2006) Amyloid precursor protein-mediated free radicals and oxidative damage: implications for the development and progression of Alzheimer’s disease. J Neurochem 96:1–13
Ryter S, Alam J, Choi A (2006) Heme oxygenase-1/carbon monoxide: from basic science to therapeutic applications. Physiol Rev 86:583–650
Schipper H (2000) Heme oxygenase-1: role in brain aging and neurodegeneration. Exp Gerontol 35:821–830
Schipper H, Cissé S, Stopa E (1995) Expression of heme oxygenase-1 in the senescent and Alzheimer-diseased brain. Ann Neurol 37:758–768
Shen C, Chen Y, Liu H, Zhang K, Zhang T, Lin A, Jing N (2008) Hydrogen peroxide promotes Abeta production through JNK-dependent activation of gamma-secretase. J Biol Chem 283:17721–17730
Shih AY, Johnson DA, Wong G, Kraft AD, Jiang L, Erb H, Johnson JA, Murphy TH (2003) Coordinate regulation of glutathione biosynthesis and release by Nrf2-expressing glia potently protects neurons from oxidative stress. J Neurosci 23:3394–3406
Shih AY, Imbeault S, Barakauskas V, Erb H, Jiang L, Li P, Murphy TH (2005a) Induction of the Nrf2-driven antioxidant response confers neuroprotection during mitochondrial stress in vivo. J Biol Chem 280:22925–22936
Shih AY, Li P, Murphy TH (2005b) A small-molecule-inducible Nrf2-mediated antioxidant response provides effective prophylaxis against cerebral ischemia in vivo. J Neurosci 25:10321–10335
Takahashi T, Morita K, Akagi R, Sassa S (2004) Heme oxygenase-1: a novel therapeutic target in oxidative tissue injuries. Curr Med Chem 11:1545–1561
Tamagno E, Guglielmotto M, Aragno M, Borghi R, Autelli R, Giliberto L, Muraca G, Danni O, Zhu X, Smith M, Perry G, Jo D, Mattson M, Tabaton M (2008) Oxidative stress activates a positive feedback between the gamma- and beta-secretase cleavages of the beta-amyloid precursor protein. J Neurochem 104:683–695
Tanaka A, Hamada N, Fujita Y, Itoh T, Nozawa Y, Iinuma M, Ito M (2010) A novel kavalactone derivative protects against H2O2-induced PC12 cell death via Nrf2/ARE activation. Bioorg Med Chem 18:3133–3139
Wang X, Hauptmann N, Taylor E, Foreman M, Khawli LA, Maines MD (2003) Neotrofin increases heme oxygenase-1 selectively in neurons. Brain Res 962:1–14
Wang H, Xu Y, Yan J, Zhao X, Sun X, Zhang Y, Guo J, Zhu C (2009a) Acteoside protects human neuroblastoma SH-SY5Y cells against beta-amyloid-induced cell injury. Brain Res 1283:139–147
Wang J, Du XX, Jiang H, Xie JX (2009b) Curcumin attenuates 6-hydroxydopamine-induced cytotoxicity by anti-oxidation and nuclear factor-kappa B modulation in MES23.5 cells. Biochem Pharmacol 78:178–183
Wang HQ, Sun XB, Xu YX, Zhao H, Zhu QY, Zhu CQ (2010) Astaxanthin upregulates heme oxygenase-1 expression through ERK1/2 pathway and its protective effect against beta-amyloid-induced cytotoxicity in SH-SY5Y cells. Brain Res 1360:159–167
Wruck C, Götz M, Herdegen T, Varoga D, Brandenburg L, Pufe T (2008) Kavalactones protect neural cells against amyloid beta peptide-induced neurotoxicity via extracellular signal-regulated kinase 1/2-dependent nuclear factor erythroid 2-related factor 2 activation. Mol Pharmacol 73:1785–1795
Yan J, Sun XB, Wang HQ, Zhao H, Zhao XY, Xu YX, Guo JC, Zhu CQ (2010) Chronic restraint stress alters the expression and distribution of phosphorylated tau and MAP2 in cortex and hippocampus of rat brain. Brain Res 1347:132–141
Acknowledgment
This study was supported in part by the National Natural Science Foundation of China (Grants 81071017 and 90919004).
Author information
Authors and Affiliations
Corresponding author
Additional information
Hong-Quan Wang and Yu-Xia Xu contributed equally to this work.
Rights and permissions
About this article
Cite this article
Wang, HQ., Xu, YX. & Zhu, CQ. Upregulation of Heme Oxygenase-1 by Acteoside Through ERK and PI3 K/Akt Pathway Confer Neuroprotection Against Beta-Amyloid-Induced Neurotoxicity. Neurotox Res 21, 368–378 (2012). https://doi.org/10.1007/s12640-011-9292-5
Received:
Revised:
Accepted:
Published:
Issue Date:
DOI: https://doi.org/10.1007/s12640-011-9292-5