Abstract
The aim of this study was to investigate the effect of Lactobacillus plantarum and Bacillus coagulans on serum lipid profile and lowering potential of probiotic in hypercholesterolemic rats. Twenty-eight male Wistar rats were divided into four groups as follows: (1) control group, fed standard commercial diet; (2) HC group, fed high-cholesterol diet; (3) HC + LP group, fed high-cholesterol diet and gavaging of L. plantarum; and (4) HC + BC group fed high-cholesterol diet and gavaging of B. coagulans. After 28 and 50 days, serum lipid profile; serum ALT and AST; the body and organ weights; fecal total count; Enterobacteriaceae, L. plantarum, and B. coagulans counts; and blood glucose tolerance were measured. We observed that levels of triglyceride, cholesterol, LDL, VLDL, and atherogenic index in serum were significantly lower in the HC + probiotic groups. Also, serum ALT and AST were significantly decreased in probiotic-treated groups. In addition, we found that feeding of a high-cholesterol diet for 50 days produced significant increases in the body weight, in addition to the fact that the administration of L. plantarum and B. coagulans has considerably reduced the body weight gain. B. coagulans and L. plantarum can survive passing through the upper-gastrointestinal tract after oral feeding to the rats and colonized in their colon. These bacteria could be exploited as a potential biotherapeutic remedy to reduce TC, TG, LDL, VLDL, and atherogenic index in hypercholesterolemic condition.
Similar content being viewed by others
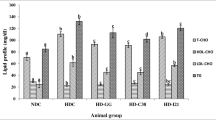
Avoid common mistakes on your manuscript.
Introduction
Probiotics are defined as “living microorganisms, which upon ingestion in certain numbers exert health benefits on the host beyond inherent basic nutrition” [1]. Various investigations on probiotics indicated the positive influence on the intestinal flora of the host, increased mucosal immunity, reduced inflammatory or allergic reactions, and reduced blood cholesterol. Furthermore, they possess anti-colon cancer effects and many other benefits [2].
Hypercholesterolemia occurs when there is an elevated level of total cholesterol (TC) in the bloodstream. This can result from high levels of low-density lipoprotein cholesterol (LDL) as compared to high-density lipoprotein cholesterol (HDL) [3].
Hypercholesterolemia is a major risk factor associated with coronary heart diseases, and it is considered that keeping TC at a desirable level is one of the major preventive strategies for these diseases [3]. Dietary management, behavior modification, regular exercise, and drug therapies are modalities for lowering TC levels [4]. High cost of medicines, undesirable side effects of these compounds, and some health concerns about their long-term therapeutic use are the major limitations for pharmacological therapy. Therefore, use of probiotics for prevention of hypercholesterolemia receives considerable attention of scientific community [2, 5].
Human studies have shown promising evidence that well-established probiotics possess hypocholesterolemic effects, while new strains of probiotics have been evaluated in animal models for their potential hypocholesterolemic effects [6]. There are some cholesterol lowering mechanisms proposed by Lactobacillus strains. One of the most important mechanisms is through the bile salt hydrolase (BSH) enzyme which is the enzyme responsible for bile salt deconjugation in the enterohepatic circulation [7]. In 1974, Mann and Spoerry published the first scientific article associating lactic acid bacteria with a reduction of 18% serum cholesterol of warriors of an African tribe (Masai and Sambura) whose regular diet included milk fermented by Lactobacillus [5].
Eleven strains of lactobacilli were screened and analyzed for bile salt deconjugation ability, bile salt hydrolase activity (BSH), and coprecipitation of cholesterol with deconjugated bile by Liong and Shah (2005). Their result showed that Lactobacillus acidophilus strains had higher deconjugation ability than L. casei strains [8].
“Besides the commonly explored strains, bacterial spore formers, mostly of the genus Bacillus do carry probiotic attributes. The value of non-spore former LAB for the maintenance of human and animal health has been acknowledged both scientifically in terms of published research data and commercially in form of the availability of probiotic products. However, in comparison to LAB, bacterial spore formers have not gained high popularity, particularly in terms of research interest. Several Bacillus strains have been screened for their potential probiotic functionalities, in several in vitro and in vivo models. Besides qualifying the mandatory bench marks for a candidate probiotic, Bacillus spp. offers higher acid tolerance and better stability during heat processing and low temperature storage. Additionally, they have also been shown to possess pathogen exclusion, anti-oxidant, anti-microbial, immuno-modulatory, and food fermentation abilities” [9]. The present study was undertaken to explore the, therapeutic potential and comparing of probiotic Lactobacillus plantarum and Bacillus coagulans, with a particular focus on the cholesterol-lowering potential and lipid profile changes in rats.
Methods
Preparation of Probiotic Bacteria
Lyophilized probiotic B. coagulans was donated by the Pardis Roshd Mehregan Company, Iran. It was grown aerobically in Nutrient Yeast Extract Salt Medium (NYSM) broth (this was prepared by the method of Russell et al. [10] and incubated at 37 °C for 48 h). The spore suspension of the bacterium was finally prepared at the concentration of 1 × 109 spores/ml in a sterile saline. Lactococcus plantarum was grown in MRS broth for 24 h at 37 °C to adjust the cell counts to 109 CFU/ml. The cells were pelleted by centrifugation at 6000×g for 5 min, washed twice with sterile saline, then were resuspended in sterile saline to obtain counts of approximately 107 CFU/ml. The suspension was prepared daily for feeding to rats.
Experimental Design
Twenty-eight 8-week-old male Wistar rats (weighing 200 ± 10 g) were prepared from the Razi Vaccine and Serum Research Institute, Shiraz, Iran, and kept in standard polypropylene cages. They were maintained in a central, small animal house at a controlled temperature of 22 ± 2 °C and relative humidity of 56% to 60% with 12-h light exposure in a daily cycle from 7 a.m. to 7 p.m. with ad libitum access to diet and tap water. The animals were fed with commercially standard pellet.
After an acclimatization period of 1 week, the animals were randomly divided into four groups (n = 7/group) as follows: (1) control group, fed standard commercial diet; (2) HC group, fed high-cholesterol diet containing commercial diet with mix of 1% cholesterol (Merck, Darmstadt, Germany) and 10% egg yolk powder (Narin company, Iran); (3) HC + LP group, fed high-cholesterol diet and gavaging of 1 ml/day of 107 CFU/ml of L. plantarum; and (4) HC + BC group, fed high cholesterol and gavaging of 1 ml/day of 109 spores/ml of B. coagulans. Control and HC groups were gavaged 1 ml of normal saline instead. Body weight of the rats was recorded weekly during the 50 days of experiments.
Collection of Blood and Organ Samples
Following 28 and 50 days of the experiments, four and three overnight-fasted rats were, respectively, anesthetized and bled from heart for serum collections. After bleeding, rats were euthanized using saturated diethyl ether in desiccators. Serum was separated by centrifugation at 3000×g for 15 min and kept at − 70 °C for subsequent biochemical analysis.
Liver, heart, spleen, and kidneys were excised, rinsed in chilled saline solution, then blotted on filter paper, and weighed separately to calculate the organ’s weight.
Serum and Liver Sample Analysis
The serum samples were analyzed for TC, triglyceride (TG), and HDL by colorimetric reactions using specific enzymatic kits (Biorex Fars, Iran). The Friedewald equation was used to analyze other plasma lipid fractions including LDL, very low-density lipoprotein cholesterol (VLDL), atherogenic index, LDL/HDL ratio, and TC/HDL ratio [11].
The serum samples were also analyzed for liver enzymes ALT and AST by colorimetric reactions using specific enzymatic kits (Biorex Fars, Iran).
Liver lipids were extracted and purified according to the method of Hara et al. [12] with some changes. Briefly, 18 ml of hexane:isopropanol (3:2) was added to 1 g of liver sample; the mixture was homogenized (DI 18B, IKA, Germany) at 5000 rpm for 30 s and maintained overnight at 4 °C. The mixture was centrifuged at 5000×g for 20 min. Non-lipids in the extract were removed by mixing the supernatant two times with 12 ml of aqueous sodium sulfate (prepared from 1 g of the anhydrous salt and 15 ml of water). Two layers formed in tubes and the lipids were in the upper, hexane-rich layer. TG and cholesterol of the lipid extract were measured by colorimetric reactions using specific enzymatic kits (Biorex Fars, Iran).
Microbiological Analysis of Fecal Sample
For the bacteriological analysis, fecal samples were collected on days 21 and 50 of the experiments in separate sterile tubes which were promptly processed within 1 h of collection. Each sample was, respectively, plated in duplicate on the plate count agar, VRBD agar (Merck, Darmstadt, Germany), and NYSM agar, for total, Enterobacteriacae, and B. coagulans counts, respectively.
Oral Glucose Tolerance Test
On days 28 and 50 of the experiments, four and three animals were, respectively, deprived of the diets for 12 h and then were given 5 g/kg BW glucose solution (50% w/v) by intragastric gavage. Blood samples were drawn from the tail every 30 min for oral glucose tolerance testing. Blood glucose was measured at 0, 30, 60, 90, and 120 min by a rapid blood glucose meter (Accu-chek Company, USA) [13].
Animal Ethics
This experimental study was accomplished under the approval of the State Committee on Animal Ethics, Shiraz University, Shiraz, Iran (ethical approved number 1392/909342).
Statistical Analysis
Data analysis was carried out with SPSS Inc. software (version 19). One-way analysis of variance was used to study significant difference between means, with significance level at α = 0.05. Duncan’s multiple comparison test was used to perform multiple comparisons between means. All data presented are three replicates.
Results
In this study we examined the lipid-lowering potential of probiotics L. plantarum and B. coagulans in Wistar rats fed high-cholesterol diet. Results showed that the body weight of all groups increased every week, especially the group of the high-cholesterol-fed groups compared with the control group. The high-cholesterol diet promoted a significant increase in body weight over the 50-day period (Fig. 1). On days 35, 42, and 50 of experiment, the weight gain on HC group was significantly more than the control. The weight gain was strictly controlled in the animals fed on the diet containing L. plantarum and B. coagulans.
The effect of supplementation of diet with probiotics L. plantarum and B. coagulans on body weight of hypercholesterolemia male Wistar rats during 50-day treatment. Control (green diamond), high cholesterol (orange square), high cholesterol + L. plantarum (red triangle), high cholesterol + B. coagulans (blue circle). Means with asterisks in each day of treatment are significant (p < 0.05)
After 28 and 50 days of treatment, liver, heart, and spleen weight of treated rats showed no significant difference in comparison to control (Table 1). On day 28 of experiment, cholesterol-enriched diet had significantly increased kidney weight. However, on day 50, kidney weight of HC group was not increased and was similar to that of control, and HC + LP and HC + BC groups did not show any significant changes in kidney weight (Table 1).
The effects of the dietary treatments on the serum lipid profile (TG, TC, LDL, HDL, VLDL and atherogenic index, TC/HDL and LDL/HDL ratios) are presented in Fig. 2.
The effect of supplementation of diet with probiotic bacteria on serum triglyceride (a), total cholesterol (b), LDL-cholesterol (c), HDL-cholesterol (d), VLDL-cholesterol (e), atherogenic index (f), cholesterol/HDL ratio (g), and LDL/HDL ratio (h) in hypercholesterolemia male Wistar rats. Control (), high cholesterol (
), high cholesterol + L. plantarum (
), high cholesterol + B. coagulans (
). Bars represent standard deviation values. Means with different letters in the same sampling day indicate significant differences at 0.05 level
Treatment groups receiving L. plantarum (HC + LB) and B. coagulans (HC + BC) showed significantly lower TG than HC group on days 28 and 50 (Fig. 2a). The effect of supplementing different prebiotics on TC is shown in Fig. 2b. This result showed that there was a significantly higher TC on days 28 and 50 by 31.1% and 41.6% for HC diet, respectively, and probiotic groups significantly controlled TC and was similar to control diet.
Statistical analysis for the effect of cholesterol-enriched diet and different probiotic on LDL on day 28 indicated significant increase in HC group compared to the control group by 87.8% while HC + LP and HC + BC caused less decrease than control group, i.e., by 37.6% and 69.6%, respectively. On day 50 of treatment, HC diet caused significant increase in serum LDL by 184.6% compared to control and probiotic groups significantly controlled LDL and was similar to control diet (Fig. 2c). Results of serum HDL are shown in Fig. 2d, which showed that HC diet significantly decreased on day 50 while serum HDL in groups having probiotics was similar to control.
HC diet caused significant increase in serum VLDL after 28 and 50 days of treatment and treatment groups receiving HC + LP and HC + BC showed significantly lower VLDL than HC group which was close to control diet on day 50 (Fig. 2e).
In this study HC diet caused 72.5% and 144.6% increase in serum atherogenic index after 28 and 50 days of treatment, respectively. While HC + LP and HC + BC groups showed significant decreasing effect both on days 28 and 50 and probiotics showed significantly less atherogenic index than control group on day 28 (Fig. 2f).
The statistical analysis on day 28 and 50 showed that serum TC/HDL ratio differed significantly among the experimental groups (Fig. 2g). HC diet significantly increased TC/HDL ratio by 34.5% and 68.8% compared to control, whereas HC + LP and HC + BC diet was significantly lower and was similar to the control diet. Also, there was no significant difference between HC + LP and HC + BC diet.
On day 28 of treatment, LDL/HDL ratio was significantly lower in rats fed HC + LP and HC + BC diet compared with controls, whereas it was significantly greater in rats fed HC diet. LDL/HDL ratio of probiotic groups was similar to control group on day 50 and was significantly less than HC group (Fig. 2h).
Results of serum ALT and AST are shown in Fig. 3. Except control group, no significant difference in serum ALT activity of all groups was seen on day 28, but it significantly decreased in HC + LP and HC + BC groups on day 50 compared to HC diet and were close to control group. On day 28, HC diet had a significant increasing effect on serum AST by 34.6% and the level of this enzyme significantly decreased in HC + LP and HC + BC groups. Another factor that might have contributed to this difference is slight change in the composition of diet due to the addition of supplements.
The effect of supplementation of diet with probiotic bacteria on serum ALT and serum AST in hypercholesterolemia male Wistar rats. Control (), high cholesterol (
), high cholesterol + L. plantarum (
), high cholesterol + B. coagulans (
). Bars represent standard deviation values. Means with different letters in the same sampling day indicate significant differences at 0.05 level
HC diet significantly increased liver triglyceride on days 28 and 50 of treatment. However, probiotic groups significantly controlled liver triglyceride increase on day 50 (Fig. 4a). There was no significant difference between treated groups on liver total cholesterol on day 28. However, effect of high-cholesterol diets on liver total cholesterol showed significant increase on day 50 compared to control (Fig. 4b).
The effect of supplementation of diet with probiotic bacteria on liver triacylglyceride (a) and liver cholesterol (b) in hypercholesterolemia male Wistar rats. Control (), high cholesterol (
), high cholesterol + L. plantarum (
), high cholesterol + B. coagulans (
). Bars represent standard deviation values. Means with different letters in the same sampling day indicate significant differences at 0.05 level
The effects of the dietary treatments on total count, Enterobacteriaceae count, L. plantarum count, and B. coagulans count of feces are presented in Table 2. Except control, all groups had significantly less fecal total count on day 21; however, on day 50, HC diet had significantly less fecal total count and probiotic groups were similar to control.
In groups HC and HC + LP, significant increase in fecal Enterobacteriaceae count compared to control diet after 21 days was observed and B. coagulans significantly controlled the increase of fecal Enterobacteriaceae count (Table 2). Both probiotics significantly decreased fecal Enterobacteriaceae count over the 50 days compared to HC group.
As expected, L. plantarum count significantly increased in HC + LP group compared to other treatment groups on days 21 and 50 of experiment. Also, B. coagulans count significantly increased in HC + BC group compared to others. However, high-cholesterol diet did not significantly increase L. plantarum and B. coagulans count in rat’s feces.
Table 3 shows the results of glucose tolerance test in rats performed before sacrificing. On day 28, at zero time, blood glucose of HC, HC + LP, and HC + BC groups was significantly higher than the control. Also, there was no significant difference between groups on 30, 60, 90, and 120 min. For control and HC + BC groups, blood glucose significantly dropped after 120 min, but for HC and HC + LP groups, it did not drop after 120 min. On day 50, except group HC + BC, the increase of blood glucose was not significant on time zero, but HC diet significantly increased blood glucose at times 60 and 120. Moreover, there was no significant difference between groups on 30, 60, 90, and 120 min.
Discussion
It is well known that high concentrations of cholesterol and LDL-cholesterol in the blood account for increasing risk of cardiovascular diseases [14]. Hence, the incidence of CVD may be lowered by decreasing of serum/plasma cholesterol (total and LDL) in hypercholesterolemic patients [13]. Probiotics are defined as “living microbial supplements that beneficially affect the host animals by improving its intestinal microbial balances” [6]. There have been reputable studies on the ability of probiotics to lower cholesterol levels in the body [4, 5]. The possible mechanisms involved in the hypocholesteremic effect include the deconjugation of bile by BSH, an assimilation of cholesterol by growing cells, the binding action of bile by fiber, the binding of cholesterol to the microbial cellular surface, the microbial production of short-chain fatty acids (SCFAs) in the colon, and coprecipitation of cholesterol with deconjugated bile [15]. These hypotheses need to be confirmed in animal and human studies [4, 16, 17]. L. plantarum and B. coagulans, are proved probiotic strain, have rarely been tested and compared for their cholesterol-lowering potential. Hence, this study was designed specifically to test their effect on lipid profile and intestinal micro biota of hypercholesterolemia-induced Wistar rats.
In our case, although only a limited number of rats were involved, the addition of cholesterol significantly increased the weight gain of rats after 50 days from the beginning of its application. Also, the weight gain was controlled in the animals fed on the diet containing L. plantarum and B. coagulans. These findings are in agreement with Haroun et al. [15]. However, An et al. [18] showed that the gain in body weight of rats after 7 weeks was significantly higher in the groups fed high-fat diet alone than in the group fed high-fat diet and lactic acid bacteria together.
Our research presented no increase in body organs weight of rats after supplementation of their diet with cholesterol. In An et al.’s research [18], no significant change in weight of spleen, kidney, and liver of rats fed high-fat diet and high fat with lactic acid bacteria were observed. These results showed that probiotics did not influence overgrowth and increase of body or organ weights, and also, high-cholesterol diets did not affect weight gain of important organs of treated animals.
The present study showed that treatment groups receiving L. plantarum (HC + LB) and B. coagulans (HC + BC) had significantly lower concentration of TG than HC group on days 28 and 50. These results are in agreement with Ranasinghe et al. [19], who suggested that probiotic feeding has an influence on lipid metabolism of animals to decrease TG. Also, Nguyen et al. [20] showed that TG was lowered by 10% as a result of the L. plantarum treatment. Sudha et al. [2] suggested that the lowering of triglycerides by probiotic strains may be due to production of lipase by these organisms, which breaks larger molecules of fats into simple and easily digestible substrates.
There was a significant increasing effect of HC diet on TC on days 28 and 50 (31.1% and 41.6%, respectively), and L. plantarum (HC + LB) and B. coagulans (HC + BC) groups significantly controlled results which are similar to control diet. Abd El-Gawad et al. [3] showed that feeding yoghurt containing two strains of Bifidobacteria to rats for 6 weeks reduced the TC to 48.7% and 37.3%, respectively. Also, Kumar et al. [13] reported 32% decrease in the TC by feeding Lactobacillus rhamnosus to hypercholesterolemic rats. In vitro research of Sirilun et al. [21] on Lactobacillus spp. isolated from non-human origin indicated that L. plantarum exhibited the BSH activity and a TC reducing potential by 81.46% in active cells and the range of 13.11 to 23.28% in dead cells.
The hypocholesterolemic effect of the probiotics has been attributed to their ability to bind cholesterol in the small intestines. Another important mechanism is through the BSH enzyme which is responsible for bile salt deconjugation in the enterohepatic circulation [5, 22].
Significant differences in VLDL of rats in HC groups were noted. Also, groups fed L. plantarum and B. coagulans had lower VLDL compared to the control rats. Kumar et al. [13] observed significantly less plasma VLDL concentrations on group receiving probiotic L. rhamnosus GG by 41% compared to control throughout the experiment.
In this study, serum atherogenic index was also significantly lowered as a result of the probiotic treatment, compared to HC and control groups (Fig. 4). Our data agree well with the finding of Abd El-Gawad et al. [3], which showed that feeding yoghurt containing different strains of Bifidobacteria significantly decrease the values of atherogenic index of rats fed the cholesterol-enriched diet.
The activities of ALT and AST were measured to access hepatocellular damage [23]. The present study showed that probiotics had a significant positive effect on serum ALT and AST in experimental animals. These results agree with An et al. [18]. Similar results have been observed with some specific probiotics strains [24, 25].
This study presented an increase in liver triglycerides and cholesterol of rats after supplementation of their diet with high cholesterol, and probiotics significantly controlled this increase after 50 days of experiment. In the study of Chiu et al. [14], the effects of Lactobacillus-fermented milk on lipid metabolism in hamsters fed high-cholesterol diet showed a great reduction in liver cholesterol and TG content compared to the control group. Also, statistical analysis of El-Shafie et al. [26] for the effect of feeding different probiotic bacterial strains on liver total lipid, total cholesterol, and triacylglycerol in comparison to positive control revealed that there was a higher significant decreasing effect for all groups.
Oral administration of L. plantarum and B. coagulans significantly lowered fecal total count of these bacteria in rats. It is possible that high concentration of TC in the diet of HC group has prevented growth of some species of bacteria in the large intestine of rats. However, it appears that the stimulating effect of probiotics on bacterial growth has indeed overcome the inhibitory influence of TC. An et al. [18] indicated that the fecal total lactic acid bacteria counts were clearly lower in rats fed high-fat diet than in the control group over the 7 weeks of experimental period. However, after treatment with dose of 109 CFU of lactic acid bacteria, these counts were significantly increased compared with those in the high-fat-diet group. Our results show that L. plantarum and B. coagulans can survive passing through the upper-gastrointestinal tract after oral feeding and ingested probiotics affect the intestinal environment to favor their colonization.
The effect of supplementation of diet with probiotics L. plantarum and B. coagulans on blood glucose (mg/dl) of hypercholesterolemic male Wistar rats given glucose solution (5 g/kg body weight) is presented in Table 3. The result was very complex and difficult to interpret.
Conclusions
In this communication, the cholesterol-lowering effect of supplementation of diets with two different probiotic bacteria is presented. The results of this study indicate that L. plantarum and B. coagulans could be exploited as a potential biotherapeutic remedy to reduce TC, TG, LDL, VLDL, and atherogenic index. These hypocholesterolemic effects of diets containing probiotics L. plantarum and B. coagulans which have been demonstrated in the hypercholesterolemia rats in the present study could make an effective and economic contribution in treating hypercholesterolemia, and these effects could be confirmed in human volunteers.
References
Ranadheera RDCS, Baines SK, Adams MC (2010) Importance of food in probiotic efficacy. Food Res Int 43:1–7
Sudha MR, Chauhan P, Dixit K, Babu S, Jami K (2009) Probiotics as complementary therapy for hypercholesterolemia. Biol Med 1(4):1–13
Abd El-Gawad IA, El-Sayed EM, Hafez SA, El-Zeini HM, Saleh FA (2005) The hypocholesterolaemic effect of milk yoghurt and soy-yoghurt containing Bifidobacteria in rats fed on a cholesterol-enriched diet. Int Dairy J 15:37–44
Kumar M, Nagpal R, Kumar R, Hemalatha R, Verma V, Kumar A, Chakraborty C, Singh B, Marotta F, Jain S, Yadav H (2012) Cholesterol-lowering probiotics as potential biotherapeutics for metabolic diseases. Exp Diabetes Res 12:1–14
Anandharaj M, Sivasankari B, Parveen R, Rizwana PR (2014) Effects of probiotics prebiotics, and synbiotics on hypercholesterolemia: a review. Chin J Biol 2014:1–7. https://doi.org/10.1155/2014/572754
Ooi LG, Liong MT (2010) Cholesterol-lowering effects of probiotics and prebiotics: a review of in vivo and in vitro findings. Int J Mol Sci 11:2499–2522
Liong MT, Shah NP (2005) Bile salt deconjugation and BSH activity of five bifidobacterial strains and their cholesterol co-precipitating properties. Food Res Int 38:135–142
Liong MT, Shah NP (2005) Acid and bile tolerance and cholesterol removal ability of lactobacilli strains. J Dairy Sci 88:55–66
Elshaghabee FMF, Rokana N, Gulhane RD, Sharma C, Panwar H (2017) Bacillus as potential probiotics: status, concerns, and future perspectives. Front Microbiol 8:1490
Russell BL, Jelley SAA, Yousten AA (1989) Selective medium for mosquito pathogenic strains of Bacillus sphaericus 2362. J Appl Environ Microbiol 55:294–297
Friedewald WT, Levy RI, Fredrickson DS (1972) Estimation of the concentration of low-density lipoprotein cholesterol in plasma, without use of the preparative ultracentrifuge. Clin Chem 18:499–502
Hara AS, Radain N (1978) Lipid extraction of tissues with a low-toxicity solvent. Anal Biochem 90:420–426
Kumar M, Rakesh S, Nagpal R, Hemalatha R, Ramakrishna A, Sudarshan V, Ramagoni R, Shujauddin M, Verma V, Kumar A, Tiwari A, Singh B, Kumar R (2013) Probiotic Lactobacillus rhamnosus GG and Aloe-vera gel improve lipid profiles in hypercholesterolemic rats. Nutrition 29:574–579
Chiu CH, Lu TY, Tseng YY, Pan TM (2006) The effects of lactobacillus-fermented milk on lipid metabolism in hamsters fed on high-cholesterol diet. Appl Microbiol Biotechnol 71:238–245
Haroun BM, Refaat BM, El-Waseif AA, El- Menoufy HA, Amin HA (2013) Hypolipidemic activity of the probiotic Lactobacillus plantarum NRRL B-4496 and their prebiotic exopolysaccharide in vitro and in vivo. JASR 9(1):1015–1020
Kumar M, Verma V, Nagpal R, Kumar A, Gautam SK, Behare PV, Grover CR, Aggarwal PK (2011) Effect of probiotic fermented milk and chlorophyllin on gene expressions and genotoxicity during afb1-induced hepatocellular carcinoma. Gene 490:54–59
Lye HS, Rusul G, Liong MT (2010) Removal of cholesterol by lactobacilli via incorporation and conversion to coprostanol. J Dairy Sci 93:1383–1392
An HM, Park SY, Lee DK, Kim RJ, Cha MK, Lee SW, Lim HT, Kim KJ, Ha NJ (2011) Anti-obesity and lipid-lowering effects of Bifidobacterium spp. in high fat diet-induced obese rats. Lipids Health Dis 10(116):1–8
Ranasinghe JGS, Silva SSP, Herath N (2013) Changes of serum lipid and proteins during probiotic feeding and its exposure. IJSRP 3:1–5
Nguyen TDT, Kang JH, Lee MS (2007) Characterization of Lactobacillus plantarum PH04, a potential probiotic bacterium with cholesterol-lowering effects. Int J Food Microbiol 113:358–361
Sirilun S, Chaiyasut C, Kantachote D, Luxananil P (2010) Characterisation of non-human origin probiotic Lactobacillus plantarum with cholesterol-lowering property. Afr J Microbiol Res 4:994–1000
Oner O, Aslim B, Aydas SB (2013) Mechanisms of cholesterol lowering effects of Lactobacil and Bifidobacteria strains as potential probiotics with their bsh gene analysis. J Mol Microbiol Biotechnol 24:12–18
El Mahmoudy AM, Abdel-Fattah FA, Abd El-Mageid AD, Gheith IM (2014) Effect of the growth promotant mannan-oligosaccharide on the lipogram and organ function profile in hyperlipidemic albino rats. Am J Phytomedicine Clin Ther 2(3):334–347
Yin YN, Yu QF, Fu N, Liu XW, Lu FG (2010) Effects of four Bifidobacteria on obesity in high fat diet induced rats. World J Gastroenterol 16:3394–3401
Ma X, Hua J, Li Z (2008) Probiotics improve high fat diet induced hepatic steatosis and insulin resistance by increasing hepatic NKT cells. J Hepatol 49:821–830
El-Shafie HA, Yahia NI, Ali HA, Khalil FA, El-Kady EM, Moustafa YA (2009) Hypocholesterolemic action of Lactobacillus plantarum NRRL-B-4524 and Lactobacillus paracasei in mice with hypercholesterolemia induced by diet. Aust J Basic Appl Sci 3(1):218–228
Acknowledgements
We would like to thank Miss M. Aghazi and Mr. G. Niknia for their technical assistance.
Funding
This research was financially supported by Shiraz University.
Author information
Authors and Affiliations
Corresponding author
Ethics declarations
Competing Interests
The authors declare that they have no conflict of interest.
Ethical Approval
This work was approved by the Ethics Committee of the School of Veterinary Medicine, Shiraz University, Shiraz, Iran (ethical approved number 1392/909342).
Rights and permissions
About this article
Cite this article
Aminlari, L., Shekarforoush, S.S., Hosseinzadeh, S. et al. Effect of Probiotics Bacillus coagulans and Lactobacillus plantarum on Lipid Profile and Feces Bacteria of Rats Fed Cholesterol-Enriched Diet. Probiotics & Antimicro. Prot. 11, 1163–1171 (2019). https://doi.org/10.1007/s12602-018-9480-1
Published:
Issue Date:
DOI: https://doi.org/10.1007/s12602-018-9480-1