Abstract
Toxicity evaluation of wastewater, polluted sediment and water streams is a very crucial aspect of environmental pollution monitoring. In this work, a newly developed luminescent bacterial test using a tropical luminescent bacterium, Photobacterium sp. strain MIE was used to assess the toxicity of several heavy metals using a 15-min assay format. The assessment was carried out by exposing strain MIE to different concentrations of heavy metals ranging from 0.001 to 200 mg/L in a DTX microplate 96 wells. The toxicity result based on the inhibitory concentration (IC50) was Hg (0.053 mg/L) > Ag (0.12 mg/L) > Cu (0.85 mg/L) > Ni (12.32 mg/L) > Zn (18.72 mg/L) > Cr (26.02 mg/L). Principal Component Analysis (PCA) and Agglomerative Hierarchical Clustering (AHC) analyses showed the sensitivity (IC50) of strain MIE to several toxic heavy metals are comparable to the commercial luminescent assay, Microtox™ as both clusters together making it a good choice for an alternative near-real-time monitoring of heavy metals. The sensitivity of strain MIE towards heavy metals was proven through field trial works on several heavy metal-polluted sites in Malaysia. Thus, it is a good candidate as an early detection system for heavy metals in aquatic bodies in tropical countries.
Similar content being viewed by others
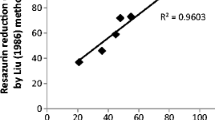
Explore related subjects
Discover the latest articles, news and stories from top researchers in related subjects.Avoid common mistakes on your manuscript.
1 Introduction
Rapid industrialization in Malaysia has been generating profit to this country while causing harm to the ecosystem caused by the untreated wastes comprising of toxic heavy metals (Mohammed et al. 2011; Selamat et al. 2017; Titah et al. 2018). Heavy metal pollution is among the major problems due to an increase in the number of polluted rivers as Malaysia is shifting into a developed country. Based on the Malaysian Department of Environment (DOE) survey, the causes of heavy metal pollution include agriculture, agro-based industries, solid waste disposal, industrial wastes, etc. (Abdullah 1995). The heavy metals that come from these sources may enter water bodies and remain persistence for a long period. Then, it will accumulate in plants and aquatic organisms and then transfer from one organism to another organism disrupting the food chains (Govind and Madhuri 2014).
Since heavy metals are dangerous, their presence must be monitored regularly (Halmi et al. 2016). Quick and ongoing detection of contaminants in waterways is necessary for safeguarding the ecosystem and public welfare (Kalogerakis et al. 2015). Thus, scientists have developed different methods to monitor toxic heavy metals in the environment. Common analytical techniques employing atomic absorption spectroscopy (AAS) and Inductive Coupled Plasma (ICP) have been implemented for measuring heavy metals in the environmental samples. These approaches are sensitive due to its capability to detect heavy metals at a very low concentration (Shukor et al. 2006). Even so, these kinds of techniques endure a lot of drawbacks including time consuming, expensive, require an advanced facility, the final result is limited to concentration, and it does not assess the level of toxicity (Kaira 2013).
The luminescent bacterial assay could be a suitable candidate for biomonitoring of heavy metals due to their low cost, simple operation and rapid response (Halmi et al. 2014). It can be employed for measuring the toxicity of individual, mixes chemical substances and suitable to be used for almost all types of environmental samples, for instance, municipal waste effluent, complex effluents, and sediment (Parvez et al. 2006). Microtox™ is among the widely recognized commercial bioassay utilizing bioluminescent bacteria. This commercial bioassay makes use of the Vibrio fischeri (formerly identified as Photobacterium phosphoreum) that has optimum assay temperatures, which range from 15 to 25 °C (Canna-Michaelidou 1993; Medvedeva et al. 2009). These assaying temperatures are not appropriate for near-real-time biomonitoring for a tropical country like Malaysia which displays a broad variation of regular temperatures up to 34 °C in the mid-day (Wong et al. 2016). Changes in several degrees Celsius out of this specific temperature can drastically influence the luminescence production by the bacterium (Dewhurst et al. 2002). The application of this type of organism would require a refrigerated water bath making the assay system expensive and instrument dependent. Hence, a broad range of activity of bioluminescent bacterium is urgently needed for on-site biomonitoring applications in tropical regions (Halmi et al. 2014).
Luminescent bacteria are facultative anaerobes marine organism found mainly in marine, freshwater, and terrestrial environments (Girotti et al. 2002). Luminescence is generated as a result of the aerobic oxidation process in the presence of an enzyme called luciferase. The reaction takes place whenever this particular enzyme oxidized the particular substrate luciferin and a reduced coenzyme flavin mononucleotide (FMN) (Kim et al. 2018; Meighen 1988). In the presence of the particular hazardous substance, the metabolic process engaged in the generation of luminescence is going to be disrupted (Choi and Gu 2002). The inhibition of luminescence is dependent upon the amount of the toxic compounds existing inside the samples. The greater the amount or concentration of toxicants, the minimum the quantity of light emitted by tested bioluminescent bacteria (Ma et al. 2014). The impact of toxicants on this bacterium could be confirmed within just 30 min or fewer relying on what kind of toxicants (Girotti et al. 2008). This bioassay system needs a shorter incubation time due to the fact that bioluminescent bacteria is a prokaryotic cell, which possesses faster metabolic activity in comparison to eukaryotic cells. The bacterial species employed for inhibition assay consists of Vibrio fischeri, Photobacterium phosphoreum, Vibrio harveyi and Pseudomonas fluorescens (Girotti et al. 2008).
In the prior work, a tropical luminescent bacterium had been isolated from Rastrelliger kanagurta and identified using 16 s rRNA as Photobacterium sp. strain MIE (GeneBank accession no. KF647218). This isolated tropical bacterium is suitable for near-real-time biomonitoring of heavy metals in the tropical environment because it exhibited a broad range of temperature and pH of the luminescent activity (Halmi et al. 2014). In this study, the sensitivity of strain MIE toward heavy metals by identifying the inhibitory concentration (IC50) using 15-min assay approach was carried out. The Principal Component Analysis (PCA) and Agglomerative Hierarchical Clustering (AHC) were also employed to investigate the correlation of strain MIE sensitivity to several heavy metals with the previous bioassay work. Finally, the sensitivity of strain MIE to heavy metals was proven through field trial works in heavy metal-polluted locations in Malaysia.
2 Materials and methods
2.1 Chemicals
All analytical grade chemicals used in this particular study were purchased either from Sigma (St. Louis, MO, USA) or from Merck (Darmstadt, Germany).
2.2 Statistical analysis
The three independent experimental replicates data were statistically analyzed by identifying means and standard deviation. Comparison among groups of tested heavy metals was acquired through a one-way analysis of variance (ANOVA) with post hoc analysis using a Student’s t test. A P value of < 0.05 was considered statistically significant. Regression curves were built using the PRISM (Prism version 5.00 for Windows), and GraphPad (GraphPad Software Inc., San Diego, CA, USA) for non-linear regression. Principal Component Analysis (PCA) and Agglomerative Hierarchical Clustering (AHC) were performed and analyzed using XLSTAT, Microsoft Excel add-on software (Trial Version).
2.3 Preparation of luminescence media
Luminescence media containing the following ingredient (g/L): NaCl: 15 g, peptone: 10 g, glycerol: 3 ml, yeast extract: 3 g. For solid media substitute with agar: 18 g (Halmi et al. 2014).
2.4 Preparation of minimal salt media (MSM)
Minimal salt media containing the following ingredient (g/l): NaCl: 15 g, glycerol: 3 ml, Na2H PO4: 7.02 g, NaH2PO4: 7.704 g (Halmi et al. 2014).
2.5 Preparation of metal ion stock solutions
Standard solution of metal ions such as mercury (Hg2+), copper (Cu2+), silver (Ag+), lead (Pb2+), chromium (Cr3+), arsenic (As+3), nickel (Ni2+), zinc (Zn2+), cobalt (Co2+), cadmium (Cd2+), (Atomic Absorption Spectrometry standard), sodium molybdenum dehydrate (H4MoNa2O6), potassium sodium tartrate (KNaC4H4O6·4H2O), aluminum oxide (Al2O3), manganese sulfate (MnSO±), lithium chloride (LiCl), sodium fluoride (NaF) and strontium chloride (SrCl2) were prepared at different concentration ranging from 1000 mg/L, 100 mg/L, 10 mg/L, 1.0 mg/L and 0.1 mg/L and stored in acid-washed polypropylene containers.
2.6 Measurement of luminescence
Beckman Counter DTX 800 multimode detector was used to measure luminescent production by strain MIE. The unit used was Relative Luminescence Unit (RLU). The measurement was taken by collecting 200 µL of working solution in DTX microplate 96 wells before the readings were taken in triplicate (Halmi et al. 2014).
2.7 Cultivation of Photobacterium sp. strain MIE
The luminescent bacterium, known as Photobacterium sp. strain MIE (GeneBank accession no. KF647218) was employed in this particular study. The bacterium was obtained from an in-house culture collection in the Faculty Biotechnology and Biomolecular Sciences at the University of Putra Malaysia (Halmi et al. 2014). One percent (v/v) of fresh bacterial culture (OD600 = 0.7–0.8) was inoculated in a conical flask containing 100 ml of luminescence media. Cells were cultivated at speed 150 rpm for 12 h at ambient temperature on a rotary shaker. The culture was centrifuged at speed 10,000×g for 10 min to remove the supernatant and substituted with minimal salt media to be a bacterial stock solution and stored in the chiller at 4 °C. The range of luminescence unit (RLU) needed for toxicity study was 50,000–100,000 RLU units (Chun et al. 1996; Hong et al. 2010). To obtain this, the bacterial stock solution was diluted using minimal salt media for hundred times. Before the assay was performed, the luminescence of the cells was measured and considered as minutes zero.
2.8 Effect of metal ions on luminescent production at 100 mg/L
Metal ions such as mercury (Hg2+), copper (Cu2+), silver (Ag+), lead (Pb2+), chromium (Cr3+), arsenic (As+3), nickel (Ni2+), zinc (Zn2+), cobalt (Co2+), cadmium (Cd2+), (Atomic Absorption Spectrometry standard), sodium molybdenum dehydrate (H4MoNa2O6), potassium sodium tartrate (KNaC4H4O6·4H2O), aluminum oxide (Al2O3), manganese sulfate (MnSO±), lithium chloride (LiCl), sodium fluoride (NaF) and strontium chloride (SrCl2) were tested at concentration 100 mg/L. The assay was performed mixing 180 µL of the bacterial working solution in DTX with 20 µL of metal ions from the 1000 mg/L of stock solution. For the control study, deionized water was used and replaced the 20 µL of metal ions (Halmi et al. 2014; Hong et al. 2010). The reading of the mixtures was taken after 15 min of incubation. All the results were measured in percentage luminescence (%) and conducted in triplicate. The metal ions exhibiting the potent inhibitory impact on the luminescent production of strain MIE will proceed with IC50 analyses
2.9 IC50 studies
The half maximal inhibitory concentration (IC50) is referring to the effectiveness of a substance in inhibiting biochemical or a specific biological function. IC50 represents the concentration of a metal ion that is required to inhibit 50% of luminescent production (Zeb et al. 2017). The IC50 measurement was performed by screening the bacterium at various concentrations of metal ions ranging from 0.001 to 100 mg/L with deionized water acting as a control to substitute the metal ions. The mixtures were incubated for 15 min at ambient temperatures (Halmi et al. 2014). The reading was taken and treated in the same manner during minutes zero. The IC50 value was determined according to previous works reported by Shukor et al. (2006) and Girotti et al. (2002). The regression curves can be generated using the PRISM (Prism version 5.00 for Windows) non-linear regression analysis software available from GraphPad, (GraphPad Software Inc., San Diego, CA, USA). The sensitivity of this assay also can be determined by calculating LOD (limits of detection) and LOQ (limits of quantification). The standard errors were determined with at least three independent experimental replicates. The LOD is three times the standard deviation of the blank. Meanwhile, LOQ is ten times the standard deviation of the blank (Shukor et al. 2006).
2.10 Field trials
Water samples were collected from different locations in Malaysia. They were obtained from industrial areas, rivers and sea water including Prai Industrial Estate and Juru River which are located in Prai, Pulau Pinang, Perak River in Perak, Port Dickson beach in Negeri Sembilan and Endau Rompin, Pahang. The 200-ml samples were collected at ten different points along the river or beach located near the industrial and agricultural activities areas. Otherwise, for industrial areas, the samples were collected at ten different points in the polluted drain beside the factories (Shukor et al. 2006). The collected samples from these sampling sites were placed in the acid-washed HDPE bottles containing several drops of 1% v/v nitric acid, HNO3. The purpose of this step was to extract out heavy metals that were bound to other compounds in the samples and to prevent it from binding to the walls of the bottle. A syringe filter with 0.45-µm pore was used to filter the samples before the samples were tested using this bioassay.
In this study, a working solution of strain MIE was prepared according to the previous section. The luminescence production was measured using a luminometer for real-time monitoring at polluted sites, and the tests were repeated in the lab for confirmation of the results obtained during on-site studies. 180 µl of the working solution was introduced in each well of the microplate. 20 µl of mercury (1 mg/L) was added as the positive control for this assay while 20 µL of deionized water was used as the negative control. The mixtures were incubated for 5 min before the reading for zero min were taken. Then, the mixtures were incubated for 15 min before a final reading was taken. The results were calculated in percentage luminescence (%). All the samples were analyzed in triplicates. In this study, conventional methods were used to identify the presence of toxicants in the water samples. The instruments that were used in this study were inductively coupled plasma–optical emission spectrometry (ICP–OES) for heavy metal detection, flow injection mercury system (FIMS) and high-performance liquid chromatography (HPLC) for xenobiotics. Heavy metals and xenobiotics standards were prepared to quantify the actual concentration of toxicants that exists in every sample. The samples were filtered first by Whatman Filter Paper No. 1 before being analyzed by ICP–OES, FIMS, and HPLC. The results obtained were compared with the results obtained from the bioassay. The purposes of this step were to compare the accuracy and to correlate the results of this bioassay with instrumental analysis.
3 Results and discussion
3.1 Effect of metal ions on luminescent production by strain MIE
Screening result demonstrates heavy metals such as Hg2+, Cu2+, Ag+, Cr3+, Ni2+, and Zn2+ gave an inhibitory effect to the luminescence activity of strain MIE (%) as much as 1.34, 1.36, 2.73, 4.01, 1.47 and 0.35%, respectively, as compared with the control (P < 0.05) that gave 100% of percentage luminescence (Fig. 1). These six toxic heavy metals giving inhibitory effect to strain MIE luminescent were considered for further IC50 studies (Hong et al. 2010). Meanwhile, other metals did not inhibit much since the percentage of luminescence shows more than 90% of luminescent activity. Heavy metal might be inhibitory, stimulatory, or even toxic to luminescent bacteria, subjected to their concentrations and level (Gikas and Romanos 2006). According to this screening result, strain MIE responded sensitively to the heavy metal identified for causing severe harm to the environment. Thus, it could be a suitable candidate for evaluating the toxicity of those heavy metals (Rosado et al. 2016).
3.2 IC50 studies
The summary of IC50, LOD, and LOQ values of inhibited heavy metals are shown in Table 1, and the regression curves are presented in Fig. 2, respectively. The result shows Photobacterium sp. strain MIE responded sensitively to mercury, copper, and silver with the lowest IC50 values 0.053 mg/L, 0.17 mg/L and 0.12 mg/L, respectively. Other heavy metals show the highest IC50 compared to these three heavy metals. The sensitivity of Photobacterium sp. strain MIE toward tested heavy metals can be summarized as the following order: Hg (0.053 mg/L) > Ag (0.12 mg/L) > Cu (0.85 mg/L) > Ni (12.32 mg/L) > Zn (18.72 mg/L) > Cr (26.02 mg/L).
The values of LOD of mercury, copper, and silver of strain MIE are below than the maximum permissible level (MPL) permitted by EPA and WHO. The LOD is the lowest concentration for metals which can be detected with 99% of the confidence interval and is usually three times the standard deviation of the blank for the y-intercept. Meanwhile, LOQ is the concentration level above which the concentration can be determined with acceptable precision (RSD < 10–25%) and accuracy (usually 80–120% recovery) and is usually ten times the standard deviation of the blank for the y-intercept (Shukor et al. 2009). Based on this result, strain MIE was sensitive enough to several toxic heavy metal ions making the bacterium a good choice for the biomonitoring of heavy metal ions in the environment.
Table 2 shows strain MIE was sensitive for certain metals such as mercury, copper, and chromium but shows less sensitive to nickel as compared with Vibrio fischeri Microtox™. Otherwise, the IC50 value of zinc was comparable to Vibrio fischeri Microtox™. The advantage of using this bacterium was that the assaying process can be performed at a temperature range from 15 to 33 °C without the use of expensive thermostat or refrigerator making this bioassay using Photobacterium sp. strain MIE cheaper and temperature stable (Halmi et al. 2014). Comparison with Deltatox showed that IC50 of mercury and copper was comparable but strain MIE is more sensitive to silver, chromium, and nickel but for zinc, Deltatox was more sensitive. Based on the results, strain MIE is more sensitive to Vibrio fischeri used in Deltatox for detection of several toxic heavy metals.
The relative sensitivity, R, of heavy metal toxicity to Photobacterium sp. strain MIE was calculated and compared with other reported bioassays. The relative toxicity is referred to as the ratio of IC50Photobacterium sp. strain MIE to IC50 of another bioassay. High values of R mean that high sensitivity to other bioassay and low sensitivity to strain MIE (Sankaramanachi and Qasim 1999). The relative sensitivity results are summarized in Table 3. It may be noted that strain MIE is considerably sensitive to all listed toxic heavy metals comparable to Vibrio fischeri Microtox™. Nevertheless, Daphnia magna and Rainbow trout demonstrated greater relative sensitivity, R value suggesting that both bioassays were very sensitive to several heavy metals. However, both bioassays required a much longer time analysis in comparison to Photobacterium sp. strain MIE that required less than 15 min of analysis.
Principal Component Analysis (PCA) and Approximation Hierarchical Cluster analysis (AHC) were carried out via self-programmed XLSTAT Microsoft Excel add-on software (Trial Version) using experimented IC50 data of ionic Hg, Ag, Cu, Ni, Zn and Cr and various IC50 data collected from the literature (Palácio et al. 2016). Principal Component Analysis (PCA) was run with the IC50 data of each inhibited heavy metals to correlate the sensitivity of strain MIE with previously reported bioassay (Bihari et al. 2006). Based on PCA (Fig. 3), the sensitivity of strain MIE seemed to be associated with V. fischeri, Microtox™ as both bioassays are far from the center and close to each other indicating that they are significantly positively correlated. Otherwise, Vibrio fischeri, Deltatox™, Daphnia magna and Rainbow trout are grouped together on the opposite side of the center; then they are significantly negatively correlated to strain MIE and V. fischeri, Microtox™ (Schüürmann et al. 1997).
Moreover, Approximation Hierarchical Cluster (AHC) analysis of inhibition profiles was carried out, revealing a comparable potential of the strain MIE to the V. fischeri, Microtox™ (Fig. 4) by grouping both bioassays in one group similar to the result acquired from Principal Component Analysis (PCA) (Sabullah et al. 2015). AHC is among the recognizable approach, which usually presents intuitive similarity relationships among any kind of test sample as well as, the overall experimental dataset, and is commonly specified simply by a tree diagram or dendrogram that gives a brief summary of the clustering process, demonstrating an illustration of the proximity and their group (Jarque et al. 2016). Both statistical results postulate a comparable capability of strain MIE to the gold standard Microtox™ approach, and thereby, strain MIE is suitable to be implemented as a quick tool to pinpoint heavy metal pollution.
3.3 Field trials
In this analysis, water samples had been gathered from different locations in Malaysia such as the Prai Industrial estate, Juru River, Perak River and from a pristine area in the Endau Rompin National Park in the state of Johor. The collected samples were analyzed using the developed bioassay for determining the capability of strain MIE as biomonitoring agent for heavy metals. The positive samples showing inhibitory effect were then validated using ICP–OES to determine the existing toxicants in the water samples. Figure 5 reveals the toxicity screening result of water samples from Prai Industrial Estate. From ten water samples examined, just three gave positive toxic response against luminescent activities. Water samples no. 1, 8 and 10 demonstrated luminescence activity reduction to 2.603, 72.01, and 77.26%, respectively, as compared to the control that giving 100% luminescent activities. The conventional analysis of positive water samples using ICP–OES pointed out the existence of several toxic heavy metals in those water samples as shown in Table 4. The Juru River located in Penang is amongst the contaminated river in Malaysia. This river is bounded by several industrial outlets such as the Prai Industrial Estate and Bukit Tengah Industrial Estate. The Juru River turns into an accumulation point of wastes coming from these industrial outlets that contribute to the water pollution of this river (Shukor et al. 2006). In this study, ten water samples were collected from different points along the Juru River. Out of the ten samples, only samples no. 5, 9 and 10 show any significant inhibitory effect (Fig. 6). The validation test results (Table 5) unveiled that the water samples contained excessive concentrations of copper and zinc exceeding the permissible limits allowed by the Department of Environment (DOE) in Malaysia. Previous biomonitoring by Shukor et al. (2006, 2008, 2009) also demonstrated that this river was polluted with these toxic heavy metals.
The inhibitory effect of the water samples obtained from Prai Industrial Area on luminescence produced by Photobacterium sp. strain MIE. The bacterium was incubated with water samples from Prai Industrial for 15 min as described in Sect. 2.10. All values represent mean ± standard error mean (SEM), (n = 3)
The inhibitory effects of water samples obtained from Juru River on luminescence produced by Photobacterium sp. strain MIE. The bacterium was incubated with water samples for 15 min as described in Sect. 2.10. All values represent mean ± standard error mean (SEM), (n = 3)
Ten water samples were collected from the Perak River for this bioassay study. Figure 7 shows that the bioassay registered no toxicity and this was validated by ICP analysis for heavy metals with zero to very low levels of heavy metals detected (Table 6). The Endau Rompin River was chosen as a negative control for the field trial studies because this river has been gazetted as Class 1 by the Malaysia Department of Environment (DOE, 2007). Four different water samples were collected from this area. As expected, the results show that there was no inhibition to luminescence production (Fig. 8). The validation test (Table 7) results also indicate that these samples did not contain other dangerous toxicants. Validation using ICP was chosen whenever a sample showed negative or positive results instead of GC–MS or HPLC for detection of xenobiotics other than heavy metals. This is because DOE has reported that the major toxicants detected in Malaysian rivers are chiefly heavy metals (DOE, 2007). Only when heavy metal analysis shows a negative result for heavy metals, but samples are positive for toxicity according to luminescence assay that further validation using GC–MS or HPLC is carried out. A similar approach is also seen in a number of monitoring projects globally. The Microtox system was used to monitor a river near an industrial site in Spain, and the chief pollutant is heavy metals with mercury, lead and zinc being the major heavy metals detected (Roig et al. 2011). Aside from river samples, sediment samples could also be assessed for heavy metals using the Microtox system. Sediments from ports in Mexico (Gonzalez-Lozano et al. 2010) and Hong Kong (Kwok et al. 2010) showed contamination by heavy metals exceeding the accepted standards using the Microtox system.
The inhibitory effects of the samples obtained from Perak River on luminescence produced by Photobacterium sp. strain MIE. The bacterium was incubated with water samples from Perak River for 15 min as described in Sect. 2.10. All values represent mean ± standard error mean (SEM), (n = 3)
The inhibitory effects of the samples obtained from Endau Rompin River on luminescence produced by Photobacterium sp. strain MIE. The bacterium was incubated with samples from Endau Rompin waterfall for 15 min as described in Sect. 2.10. All values represent mean ± standard error mean (SEM), (n = 3)
4 Conclusion
In conclusion, statistical analysis using Principal Component Analysis (PCA) and Approximation Hierarchical Cluster analysis (AHC) revealed that the sensitivity of Photobacterium sp. strain MIE to several toxic heavy metals was comparable to V. fischeri, Microtox™. The strain MIE is also temperature stable so it is possible to employ the bioassay at ambient temperature covering tropical countries. In the future, a large-scale near-real-time biomonitoring of heavy metals employing strain MIE coupled with conventional methods such as ICP, AAS will be carried to investigate the efficiencies of the bioassay before available as a commercial bioassay.
References
Abdullah AR (1995) Environmental pollution in Malaysia: trends and prospects. TRAC Trends Anal Chem 14:191–198
Bihari N, Fafand M, Hamer B, Kralj-Bilen B (2006) PAH content, toxicity and genotoxicity of coastal marine sediments from the Rovinj area, Northern Adriatic. Croatia Sci Total Environ 366:602–611
Canna-Michaelidou S (1993) MicrotoxTM response to volatile organic pollutants—implementation of the test in investigating accidental or deliberate watersupply pollution. Sci Total Environ 134:969–977
Choi SH, Gu MB (2002) A portable toxicity biosensor using freeze-dried recombinant bioluminescent bacteria. Biosens Bioelectron 17:433–440
Chun U-H, Simonov N, Chen Y, Britz ML (1996) Continuous pollution monitoring using Photobacterium phosphoreum. Resour Conserv Recycl 18:25–40
Dewhurst R, Wheeler J, Chummun K, Mather J, Callaghan A, Crane M (2002) The comparison of rapid bioassays for the assessment of urban groundwater quality. Chemosphere 47:547–554
Gikas P, Romanos P (2006) Effects of tri-valent (Cr(III)) and hexa-valent (Cr(VI)) chromium on the growth of activated sludge. J Haz Mater 133:212–217
Girotti S, Bolelli L, Roda A, Gentilomi G, Musiani M (2002) Improved detection of toxic chemicals using bioluminescent bacteria. Anal Chim Acta 471:113–120
Girotti S, Ferri EN, Fumo MG, Maiolini E (2008) Monitoring of environmental pollutants by bioluminescent bacteria. Anal Chim Acta 608:2–29
Gonzalez-Lozano MC, Mendez-Rodriguez LC, Maeda-Martinez AM, Murugan G, Vazquez-Botello A (2010) Evaluation of toxicity of polluted marine sediments from Bahía Salina Cruz, México. J Environ Sci Health Part A 45(1):121–127
Govind P, Madhuri S (2014) Heavy metals causing toxicity in animals and fishes. Res J Anim Vet Fish Sci 2:17–23
Halmi M, Jirangon H, Johari W, Abdul Rachman A, Shukor M, Syed M (2014) Comparison of Microtox and Xenoassay light as a near real time river monitoring assay for heavy metals The Scientific World Journal 2014
Halmi MIE, Khayat ME, Gunasekaran B, Masdor NA, Rahman MFA (2016) Near real-time biomonitoring of copper from an industrial complex effluent discharge site using a plant protease inhibitive assay. Bioremed Sci Technol Res 4:10–13
Hong Y, Chen Z, Zhang B, Zhai Q (2010) Isolation of Photobacterium sp. LuB-1 and its application in rapid assays for chemical toxicants in water. Lett Appl Microbiol 51:308–312
In SD (2003) Environmental technology verification report, Deltatox®, Rapid Toxicity Testing System
Jarque S, Masner P, Klánová J, Prokeš R, Bláha L (2016) Bioluminescent Vibrio fischeri assays in the assessment of seasonal and spatial patterns in toxicity of contaminated river sediments. Front Microbiol 7:1738
Kaira M (2013) Use of microorganisms, enzymes and plant proteases for heavy metals biomonitoring-a mini review. Asian J Plant Biol 1:20–24
Kalogerakis N et al (2015) The role of environmental biotechnology in exploring, exploiting, monitoring, preserving, protecting and decontaminating the marine environment. New Biotechnol 32:157–167
Khangarot B, Ray P (1987) Correlation between heavy metal acute toxicity values in Daphnia magna and fish. Bull Environ Contam Toxicol 38:722–726
Kim D-S, Choi J-R, Ko J, Kim K (2018) Re-engineering of bacterial luciferase for new aspects of bioluminescence. Curr Protein Peptide Sci 19:16–21
Kwok CK, Yang SM, Mak NK, Wong CKC, Liang Y, Leung SY, Young L, Wong MH (2010) Ecotoxicological study on sediments of Mai Po marshes, Hong Kong using organisms and biomarkers. Ecotoxicol Environ Saf 73(4):541–549
Ma XY, Wang XC, Ngo HH, Guo W, Wu MN, Wang N (2014) Bioassay based luminescent bacteria: interferences, improvements, and applications. Sci Total Environ 468:1–11
Medvedeva SE, Tyulkova NA, Kuznetsov AM, Rodicheva EK (2009) Bioluminescent bioassays based on luminous bacteria. J Sib Fed Univ Biol 2:418–452
Meighen EA (1988) Enzymes and genes from the lux operons of bioluminescent bacteria. Ann Rev Microbiol 42:151–176
Mohammed AS, Kapri A, Goel R (2011) Heavy metal pollution: source, impact, and remedies. In: Biomanagement of metal-contaminated soils. Springer, pp 1–28
Palácio SM et al (2016) Assessment of anthropogenic impacts on the water quality of Marreco River, Brazil, based on principal component analysis and toxicological assays. Water Air Soil Pollut 227:307
Parvez S, Venkataraman C, Mukherji S (2006) A review on advantages of implementing luminescence inhibition test (Vibrio fischeri) for acute toxicity prediction of chemicals. Environ Int 32:265–268
Roig N, Nadal M, Sierra J, Ginebreda A, Schuhmacher M, Domingo JL (2011) Novel approach for assessing heavy metal pollution and ecotoxicological status of rivers by means of passive sampling methods. Environ Int 37(4):671–677
Rosado D, Usero J, Morillo J (2016) Assessment of heavy metals bioavailability and toxicity toward Vibrio fischeri in sediment of the Huelva estuary. Chemosphere 153:10–17
Sabullah MK, Sulaiman MR, Shukor MS, Yusof MT, Johari WLW, Shukor MY, Syahir A (2015) Heavy metals biomonitoring via inhibitive assay of acetylcholinesterase from Periophthalmodon schlosseri. Rend Fis Acc Lincei 26:151–158
Sankaramanachi SK, Qasim SR (1999) Metal toxicity evaluation using bioassay and microtox™. Int J Environ Stud 56:187–199. https://doi.org/10.1080/00207239908711199
Schüürmann G, Segner H, Jung K (1997) Multivariate mode-of-action analysis of acute toxicity of phenols. Aquat Toxicol 38:277–296
Selamat SN, Halmi MIEB, Abdullah SRS, Idris M, Hasan HA, Anuar N (2018) Optimization of lead (Pb) bioaccumulation in Melastoma malabathricum L. by response surface methodology (RSM). Rend Fis Acc Lincei 29:43–51
Shukor Y, Baharom NA, Rahman FA, Abdullah MP, Shamaan NA, Syed MA (2006) Development of a heavy metals enzymatic-based assay using papain. Anal Chim Acta 566:283–289
Shukor MY, Masdor N, Baharom NA, Jamal JA, Abdullah MPA, Shamaan NA, Syed MA (2008) An inhibitive determination method for heavy metals using bromelain, a cysteine protease. Appl Biochem Biotechnol 144(3):283–291
Shukor MY, Baharom NA, Masdor NA, Abdullah MPA, Shamaan NA, Jamal JA, Syed MA (2009) The development of an inhibitive determination method for zinc using a serine protease. J Environ Biol 30(1):17–22
Titah HS, Halmi MIEB, Abdullah SRS, Hasan HA, Idris M, Anuar N (2018) Statistical optimization of the phytoremediation of arsenic by Ludwigia octovalvis-in a pilot reed bed using response surface methodology (RSM) versus an artificial neural network (ANN). Int J Phytoremed 20:721–729
Wong CL, Liew J, Yusop Z, Ismail T, Venneker R, Uhlenbrook S (2016) Rainfall characteristics and regionalization in Peninsular Malaysia based on a high resolution gridded data set. Water 8:500
Zeb B et al (2017) Assessment of combined toxicity of heavy metals from industrial wastewaters on Photobacterium phosphoreum T3S. Appl Water Sci 7:2043–2050
Acknowledgements
This work was supported by the research grant from the Ministry of Science, Technology, and Innovation (MOSTI) under the Science Fund Grant no. 02-01-04-SF0445 and Geran Putra IPM/2017/9532800.
Author information
Authors and Affiliations
Corresponding author
Ethics declarations
Conflict of interests
The authors declare that there is no conflict of interests regarding the publication of this paper.
Additional information
Publisher's Note
Springer Nature remains neutral with regard to jurisdictional claims in published maps and institutional affiliations.
Rights and permissions
About this article
Cite this article
Halmi, M.I.E., Kassim, A. & Shukor, M.Y. Assessment of heavy metal toxicity using a luminescent bacterial test based on Photobacterium sp. strain MIE. Rend. Fis. Acc. Lincei 30, 589–601 (2019). https://doi.org/10.1007/s12210-019-00809-5
Received:
Accepted:
Published:
Issue Date:
DOI: https://doi.org/10.1007/s12210-019-00809-5