Abstract
Rising temperatures are severely affecting the mortality, laying performance, and meat quality of duck. Our aim was to investigate the effect of acute heat stress on the expression of heat shock proteins (HSPs: HSP90, 70, 60, 40, and 10) and inflammatory factors (nitric oxide synthase (iNOS), cyclooxygenase-2 (COX-2)) and antioxidant enzyme activity (superoxide dismutase (SOD), malondialdehybe (MDA), catalase (CAT), total antioxidant capacity (T-AOC)) in livers of ducks and to compare the thermal tolerance of Pekin and Muscovy ducks exposed to acute heat stress. Ducks were exposed to heat at 39 ± 0.5 °C for 1 h and then returned to 20 °C for 1 h followed by a 3-h recovery period. The liver and other tissues were collected from each individual for analysis. The mRNA levels of HSPs (70, 60, and 40) increased in both species, except for HSP10, which was upregulated in Muscovy ducks and had no difference in Pekin ducks after heat stress. Simultaneously, the mRNA level of HSP90 decreased in the stress group in both species. Morphological analysis indicated that heat stress induced tissue injury in both species, and the liver of Pekin ducks was severely damaged. The activities of several antioxidant enzymes increased in Muscovy duck liver, but decreased in Pekin duck. The mRNA levels of inflammatory factors were increased after heat stress in both duck species. These results suggested that heat stress could influence HSPs, inflammatory factors expression, and the activities of antioxidant enzymes. Moreover, the differential response to heat stress indicated that the Muscovy duck has a better thermal tolerance than does the Pekin duck.
Similar content being viewed by others
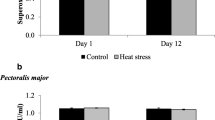
Avoid common mistakes on your manuscript.
Introduction
Warming of the climate has been evident since the 1950s, and many of the observed changes have been unprecedented over the decades. The atmosphere and ocean have warmed, the amounts of snow and ice have diminished, sea level has risen, and the concentrations of greenhouse gases have increased. Climate warming trends are strongly affecting terrestrial biological systems, resulting in changes such as earlier timing of spring events like leaf-unfolding, bird migration, and egg-laying, as well as poleward and upward shifts in the habitat range of animal species (Tomanek 2010). As environmental temperature increases, food consumption, feeding efficiency, growth rate, egg production, and survival ability decline (Mashaly et al. 2004; Sohail et al. 2012). This causes an enormous economic loss in the poultry industry.
We chose two domestic duck species (the Pekin duck and Muscovy duck) for a comparison of their thermal tolerance to acute heat stress. The former species was bred from the mallard (Anas platyrhynchos) in China, and now, most duck meat consumed in the world is that of the Pekin duck or a duck species derived from Pekin duck. The latter species is a type of a domestic duck (Cairina moschata) and is believed to have originated in South America. Each of these two duck species has a distinct biogeographical distribution in China. The Pekin duck was mostly bred in Northern China, where the temperature is much lower than in Southern China, where the Muscovy duck was bred. A knowledge of heat-responsive genes and proteins in these two duck species is therefore critical for further understanding the molecular mechanisms of stress tolerance.
All living organisms respond to environmental stressors, including hyperthermia, by synthesizing a set of proteins known as heat shock proteins (HSPs) (Becker and Craig 1994). HSPs are molecular chaperones essential for maintaining cellular functions by preventing misfolding and aggregation of nascent polypeptides and by facilitating protein folding (Hartl and Hayer-Hartl 2002). In blue mussels, heat stress-induced variations in the expression of HSP90, HSP70, HSP60, and these HSPs had a protective function in cells (Lockwood et al. 2010). In geese, the HSP70 expression levels similarly increased with heat stress (Wang et al. 2008). The mRNA levels of HSP70, HSP60, HSP40, and HSP27 increase when chicken were exposed to high temperature (Yu et al. 2008). However, there have been only a few studies on heat stress-induced HSP changes in ducks. Therefore, in this study, we investigated the level of HSPs in the liver of ducks exposed to a high-temperature environment.
Heat stress can enhance the formation of reactive oxygen species (ROS), which can cause oxidative injury due to lipid peroxidation and oxidative damage to proteins and DNA (Feng et al. 2008). The activities of superoxide dismutase (SOD), catalase (CAT), and the levels of malondialdehybe (MDA) were increased in the liver of broiler chickens exposed to high temperatures (Tan et al. 2010; Yang et al. 2010). These studies indicated that there is a potential relationship between heat stress and the antioxidant defense system in animals. Inflammation is an important indicator of animal tissue damage due to heat stress. Therefore, in this study, we investigated the expression of inflammatory factors like nitric oxide synthase (iNOS) and cyclooxygenase-2 (COX-2) in the livers of ducks exposed to high temperature.
Given this background, our objectives were (1) to investigate the effect of acute heat stress on the expression of HSPs (HSP90, 70, 60, 40, and 10), inflammatory factors, and antioxidant enzyme activity in livers of ducks and (2) to compare the thermal tolerance of Pekin ducks and Muscovy ducks exposed to acute heat stress.
Materials and methods
Birds and tissue collection
The animal care and use protocol was approved by the Institutional Animal Care and Use Committee of Nanjing Agricultural University (Nanjing, China). Ducks were raised in Huzhou, Zhejiang Province, China. The animals were kept in a temperature-controlled room with flowing air and sufficient water and fed a standard diet three times daily during the experimental period. For the hyperthermia challenge, both species were maintained at 20 °C for 2 weeks before the experiments. The temperature was increased at 10 °C · h−1 from 20 °C to 39 ± 0.5 °C. The ducks were kept at this temperature for 1 h and then returned to 20 °C for 1 h followed by a 3-h recovery period. Samples were collected from ducks after heat exposure (39 °C) and then at 1 and 3 h after heat exposure (recovery period). Six individuals were selected at each sampling time point and from the controls that had been maintained at 20 °C. The liver, brain, pituitary, adrenal, heart, spleen, and kidney were collected from each individual.
RNA extraction and quantitative real-time PCR
Total RNA was extracted using the EasyPure™ RNA Kit (TransGen Biotech Co. Ltd., Beijing, China). The concentration and purity of the total RNA were determined with a spectrophotometer (NanoVue, GE Healthcare, Piscataway, NJ, USA), and the integrity was examined using 1.2 % agarose gels containing 0.1 % ethidium bromide. Part (1 μg) of the total RNA obtained from each extraction was reverse-transcribed in a 20-μL reaction volume by using the TransScript First-Strand cDNA Synthesis SuperMix (TransGen, Beijing, China) following the manufacturer’s instructions. The primers were designed based on the corresponding gene sequences from A. platyrhynchos (Table 1). The quantitative real-time PCR (qPCR) was performed on an ABI 7300 (Applied Biosystems, Foster City, CA, USA). Reactions were performed in a 20-μL reaction mixture containing 2-μL cDNA template, 0.4-μM forward/reverse primer, 10-μL 2× SYBR qPCR Mix, and 0.4-μL ROX reference dye (Takara, Osaka, Japan). The cycling protocol included an initial step at 94 °C for 3 min, followed by 40 cycles of denaturation at 94 °C for 10 s and annealing at 60 °C for 30 s. Experiments for the detection of all the genes, including β-actin, were performed in triplicate. The relative expression levels of the genes tested were calculated using the 2−ΔΔCt method.
Histopathological examination
After necropsy, tissue specimens of the liver were fixed in 4 % buffered formaldehyde and routinely processed in paraffin. Thin sections of each tissue were sliced from each block and mounted on glass. Tissue sections on the slides were stained with hematoxylin and eosin (H&E). Liver sections were then subjected to a blind analysis using an Olympus light microscope to detect evidence of injury.
Determination of antioxidant enzyme activities
Liver homogenates were prepared in PBS and centrifuged at 2,500g for 10 min at 4 °C. The activities or levels of SOD, CAT, MDA, and total antioxidant capacity (T-AOC) were estimated using assay kits (Nanjing Jiancheng Bioengineering Institute, Nanjing, China). The experiments were performed with the assay kits following the manufacturer’s instruction.
Statistical analysis
Statistical analysis was carried out using the SPSS for Windows (version 13, SPSS Inc., Chicago, IL). Data from all the groups of birds were compared using one-way ANOVA followed by Tukey’s honestly significant difference test. The data are expressed as means ± standard error (SEM). Results were considered statistically significant at P < 0.05.
Results
Expression of the liver HSP90, HSP70, HSP60, HSP40, and HSP10 in both duck species
To understand the mRNA expression of HSPs in the liver of Muscovy and Pekin ducks exposed to heat stress, 5 HSPs genes (HSP90, HSP70, HSP60, HSP40, and HSP10) were investigated by qPCR. The results are shown in Fig. 1. Gene expression levels for HSP70, HSP60, and HSP40 showed similar changes in both duck species, with an upregulation in the heat stress groups and downregulation to preheat levels in the recovery groups (P < 0.05). The expression levels of HSP70 in all 6 individuals of each group were shown in Fig. 2. The HSP90 mRNA levels in the liver were significantly decreased compared to the control group in Muscovy and Pekin ducks (P < 0.05). However, we found contrasting changes in the mRNA expression levels of HSP10 in both duck species. When exposed to heat stress, the HSP10 mRNA expression levels were significantly increased in Muscovy ducks (P < 0.05), but did not change in the liver of Pekin ducks.
Effects of heat stress on the mRNA expression of HSP90, HSP60, HSP40, and HSP10 genes in the liver of Muscovy and Pekin ducks. Each value represents the mean ± SEM of six individuals. The expression levels of HSPs mRNA were analyzed by one-way ANOVA with the Turkey’s test (P < 0.05). Different letters indicate significant differences between any two groups of a duck species. M Muscovy duck, PK Pekin duck
Expression pattern of HSP70 in various tissues
The expression of HSP70 gene in various tissues of Muscovy and Pekin ducks exposed to heat stress was analyzed by quantitative real-time PCR (Fig. 3). In the control group, the expression of HSP70 gene in the heart tissue of the Muscovy duck was the highest (Fig. 3a), while the liver tissue had the highest expression in the Pekin duck (Fig. 3b). When exposed to heat stress, the HSP70 mRNA expression levels were significantly increased in the pituitary, adrenal, liver, and renal tissues of Muscovy ducks and the brain, pituitary, adrenal, heart, liver, spleen, and renal tissues of Pekin ducks.
Morphology of the liver
Histological analysis of the liver tissue from the control groups showed normal histological structures (Fig. 4(a1) and Fig. 4(b1)), whereas, the heat stress groups in both duck species displayed some tissue injury. In the Pekin ducks, the heat stress group showed severe liver injury, infiltration of inflammatory cells, and exudation of blood cells compared to the control group (Fig. 4(a2)). The liver injury did not get better after the recovery period (Fig. 4(a3), (a4)). In Fig. 4(b2), variable cellular vacuolization and hydropic degeneration were evident in the liver of Muscovy ducks when exposed to heat stress. However, the injury got better after the 3-h recovery period (Fig. 4(b4)).
Antioxidant enzyme activity
The alterations in SOD, MDA, CAT, and T-AOC are shown in Fig. 5. After 1-h heat stress, the activities of SOD, CAT, and T-AOC were significantly increased (P < 0.05) in the liver of Muscovy ducks. When the heat stress was removed, both the SOD and CAT activities and MDA levels gradually approached preheat stress levels, with the progression of recovery time. However, we found the opposite trend in antioxidant enzyme activities in Pekin ducks. In comparison to the control group, the SOD, MDA, CAT, and T-AOC were significantly decreased in the heat stress group and the recovery group (P < 0.05).
Expression of the liver iNOS and COX-2 in both duck species
Effects of acute heat stress on the mRNA levels of specific inflammatory genes in the liver are presented in Fig. 6. In Muscovy ducks, the iNOS mRNA levels of the heat stress groups were significantly higher than those of the control and recovery groups (P < 0.05). However, the iNOS mRNA levels were significantly decreased in the heat stress groups in Pekin ducks (P < 0.05). The mRNA expression levels of COX-2 were significantly increased in the stress groups compared to the control and recovery groups in Muscovy ducks (P < 0.05), but showed no difference in Pekin ducks.
Discussion
Exposure to abiotic or biotic stress can generate excess concentrations of ROS, such as superoxide (O 2 ˗), hydrogen peroxide (H2O2), and hydroxyl radicals (OH−) (Murphy 2009). Enhanced production of ROS can disturb cellular homeostasis by causing peroxidation of lipids, oxidation of proteins, and enzyme inhibition, ultimately leading to cell death (Mittler 2002; Maheshwari and Dubey 2009; Srivastava and Dubey 2011). The antioxidative enzyme system (comprising SOD, CAT, and GSH-Px) acts as the first line of antioxidant defense. Modification of the activity of these enzymes can alter the balance between the production of ROS and the antioxidant system. In the present study, with an increase in environmental temperatures, the activities of the major antioxidative enzymes were upregulated significantly in the liver of Muscovy ducks, but were downregulated in Pekin ducks. The T-AOC activities also indicated a similar trend in both duck species. Antioxidative enzyme activities decreased in the liver of Pekin ducks, which indicated that the ability to scavenge free radicals was impaired. Furthermore, histological analysis of liver tissues of Pekin ducks showed severe injury, infiltration of inflammatory cells, and exudation of blood cells indicating that heat stress caused serious oxidative damage to Pekin ducks. In other words, these changes suggest that Muscovy ducks have a better thermal tolerance than Pekin ducks.
Inflammation is an important indicator of animal tissue damage and host defenses elicited by heat stress. Inducible nitric oxide synthase is responsible for increased nitric oxide synthesis in tissues during inflammatory processes (Concannon et al. 2003; Suzuki et al. 2002; Dijkstra et al. 1998). Cold exposure significantly increased iNOS gene expression in the lungs of broiler chickens (Zhang et al. 2011). In the present study, the mRNA expression of iNOS was increased by heat exposure in Muscovy ducks, but decreased in Pekin ducks, which probably indicates the establishment of a host defense mechanism in Muscovy ducks. COX-2 inhibitors reduce mucosal damage and regulate the role of prostaglandins in tissue damage and the inflammation process (Zheng et al. 2012). In this study, the mRNA expression of COX-2 increased in both duck species, suggesting that they play a potential role in inducing inflammation after heat stress.
In order to determine the effects of heat stress on the expression of HSPs, we investigated the expression levels of HSP90, HSP70, HSP60, HSP40, and HSP10 in the normal duck liver, after exposure to heat stress and after a recovery period. Acute heat stress remarkably decreased the level of HSP90 expression in the broiler chicken’s heart (Yu et al. 2008). The mRNA expression of HSP90 was decreased after cold stress (Zhao et al. 2013). In the present study, the expression of HSP90 was decreased in both duck species after heat stress, which indicated that the liver had been damaged by heat stress. Expression of HSP70, HSP60, and HSP40 were increased significantly after heat stress (Zhao et al. 2013; Yu et al. 2008; Zeng et al. 2013). Consistent with these prior studies, our results showed that when ducks were exposed to heat stress, HSP70, HSP60, and HSP40 expression was increased. More importantly, it showed an abrupt decline in HSP70 mRNA from heat stress to recovery for the Muscovy ducks. The overexpression of HSPs may be the reason for the increased requirement of ATP after heat stress (Koelkebeck and Odom 1995). In our study, we observed a decline in HSP70 mRNA in Muscovy ducks during recovery. We hypothesize that this decline may result in greater production of ATP against heat stress, but further investigation is needed. These HSPs may act as important markers and protective proteins in response to adverse environmental conditions. In addition, we analyzed the expression pattern of HSP70 in various tissues of Muscovy and Pekin ducks. HSP70 mRNA was detected in various organs and was upregulated by heat stress in both duck species, which implies that the HSP70 gene is activated when cells are exposed to heat stress.
Increased synthesis of small HSP (sHSP) can be triggered through the ROS-medicated denaturation of cytoskeletal proteins (Arrigo 2007). Upon heat stress, sHSP bind and stabilize cytoskeletal proteins, exert apoptotic activity, and modulate the oxidative and heat stress response, suggesting that sHSP can stabilize cytoskeletal proteins (Concannon et al. 2003). Four sHSP isoforms showed different responses to heat stress in invasive and native blue mussels (Tomanek and Zuzow 2010). HSP24 was induced in warm-adapted mussels in response to heat stress and not in more heat-sensitive mussels (Lockwood et al. 2010). In our study, we found that the expression levels of HSP10 were upregulated after heat stress in Muscovy ducks, but were not altered in the liver of Pekin ducks. This is a further indication that heat stress may adversely affect the molecular responses in Pekin duck liver tissue.
In conclusion, this study is the first to provide insights into the differential thermal responses of Muscovy and Pekin ducks. The activities of the major antioxidative enzymes were upregulated significantly in the liver of Muscovy ducks, but were downregulated in Pekin ducks. These findings suggest that Muscovy ducks have a better thermal tolerance than Pekin ducks. The increased expression levels of HSPs (HSP70, HSP60, and HSP40) demonstrated that HSPs played an important role in the protection of duck liver from heat stress. Moreover, the changes in the mRNA expression levels of HSP10 differed in Muscovy and Pekin ducks. Taken together with previous reports, HSP10 may have a key role in facilitating thermal tolerance in ducks. However, the response mechanism of heat stress and regulatory networks among HSPs are unclear, and further studies are needed.
References
Arrigo AP (2007) The cellular “networking” of mammalian Hsp27 and its functions in the control of protein folding, redox state and apoptosis. Adv Exp Med Biol 594:14–26
Becker J, Craig EA (1994) Heat-shock proteins as molecular chaperones. Eur J Biochem 219(1–2):11–23
Concannon CG, Gorman AM, Samali A (2003) On the role of Hsp27 in regulating apoptosis. Apoptosis 8(1):61–70
Dijkstra G, Moshage H, van Dullemen HM, de Jager-Krikken A, Tiebosch AT, Kleibeuker JH, Jansen PL, van Goor H (1998) Expression of nitric oxide synthases and formation of nitrotyrosine and reactive oxygen species in inflammatory bowel disease. J Pathol 186(4):416–421
Feng J, Zhang M, Zheng S, Xie P, Ma A (2008) Effects of high temperature on multiple parameters of broilers in vitro and in vivo. Poult Sci 87(10):2133–2139
Hartl FU, Hayer-Hartl M (2002) Molecular chaperones in the cytosol: from nascent chain to folded protein. Science 295(5561):1852–1858
Koelkebeck KW, Odom TW (1995) Laying hen responses to acute heat stress and carbon dioxide supplementation: II. Changes in plasma enzymes, metabolites and electrolytes. Comp Biochem Physiol A Physiol 112(1):119–122
Lockwood BL, Sanders JG, Somero GN (2010) Transcriptomic responses to heat stress in invasive and native blue mussels (genus Mytilus): molecular correlates of invasive success. J Exp Biol 213(Pt 20):3548–3558
Maheshwari R, Dubey R (2009) Nickel-induced oxidative stress and the role of antioxidant defence in rice seedlings. Plant Growth Regul 59(1):37–49
Mashaly MM, Hendricks GL 3rd, Kalama MA, Gehad AE, Abbas AO, Patterson PH (2004) Effect of heat stress on production parameters and immune responses of commercial laying hens. Poult Sci 83(6):889–894
Mittler R (2002) Oxidative stress, antioxidants and stress tolerance. Trends Plant Sci 7(9):405–410
Murphy MP (2009) How mitochondria produce reactive oxygen species. Biochem J 417(1):1–13
Sohail MU, Hume ME, Byrd JA, Nisbet DJ, Ijaz A, Sohail A, Shabbir MZ, Rehman H (2012) Effect of supplementation of prebiotic mannan-oligosaccharides and probiotic mixture on growth performance of broilers subjected to chronic heat stress. Poult Sci 91(9):2235–2240
Srivastava S, Dubey R (2011) Manganese-excess induces oxidative stress, lowers the pool of antioxidants and elevates activities of key antioxidative enzymes in rice seedlings. Plant Growth Regul 64(1):1–16
Suzuki T, Kumamoto H, Ooya K, Motegi K (2002) Expression of inducible nitric oxide synthase and heat shock proteins in periapical inflammatory lesions. J Oral Pathol Med 31(8):488–493
Tan GY, Yang L, Fu YQ, Feng JH, Zhang MH (2010) Effects of different acute high ambient temperatures on function of hepatic mitochondrial respiration, antioxidative enzymes, and oxidative injury in broiler chickens. Poult Sci 89(1):115–122
Tomanek L (2010) Variation in the heat shock response and its implication for predicting the effect of global climate change on species’ biogeographical distribution ranges and metabolic costs. J Exp Biol 213(6):971–979
Tomanek L, Zuzow MJ (2010) The proteomic response of the mussel congeners Mytilus galloprovincialis and M. trossulus to acute heat stress: implications for thermal tolerance limits and metabolic costs of thermal stress. J Exp Biol 213(Pt 20):3559–3574
Wang BW, Wu XP, Zhang XH, Jia XH, Zhang MA, Long FY, Yang ZG, Wang L (2008) Expression and purification of goose HSP70 and compound formation with virus polypeptide. Agr Sci China 7:239–247
Yang L, Tan GY, Fu YQ, Feng JH, Zhang MH (2010) Effects of acute heat stress and subsequent stress removal on function of hepatic mitochondrial respiration, ROS production and lipid peroxidation in broiler chickens. Comp Biochem Physiol C Toxicol Pharmacol 151(2):204–208
Yu J, Bao E, Yan J, Lei L (2008) Expression and localization of Hsps in the heart and blood vessel of heat-stressed broilers. Cell Stress Chaperones 13(3):327–335
Zeng T, Jiang X, Li J, Wang D, Li G, Lu L, Wang G (2013) Comparative proteomic analysis of the hepatic response to heat stress in muscovy and pekin ducks: insight into thermal tolerance related to energy metabolism. PLoS One 8(10):e76917
Zhang ZW, Lv ZH, Li JL, Li S, Xu SW, Wang XL (2011) Effects of cold stress on nitric oxide in duodenum of chicks. Poult Sci 90(7):1555–1561
Zhao FQ, Zhang ZW, Wang C, Zhang B, Yao HD, Li S, Xu SW (2013) The role of heat shock proteins in inflammatory injury induced by cold stress in chicken hearts. Cell Stress Chaperones 18(6):773–783
Zheng M, Kang YM, Liu W, Zang WJ, Bao CY, Qin DN (2012) Inhibition of cyclooxygenase-2 reduces hypothalamic excitation in rats with adriamycin-induced heart failure. PLoS One 7(11):e48771
Acknowledgments
This work was sponsored by the earmarked fund for National Waterfowl-industry Technology Research System (CARS-43-2) and National Supporting Projects for Science and Techniques (2012BAD12B10).
Author information
Authors and Affiliations
Corresponding authors
Rights and permissions
About this article
Cite this article
Zeng, T., Li, Jj., Wang, Dq. et al. Effects of heat stress on antioxidant defense system, inflammatory injury, and heat shock proteins of Muscovy and Pekin ducks: evidence for differential thermal sensitivities. Cell Stress and Chaperones 19, 895–901 (2014). https://doi.org/10.1007/s12192-014-0514-7
Received:
Revised:
Accepted:
Published:
Issue Date:
DOI: https://doi.org/10.1007/s12192-014-0514-7