Abstract
Aimed to obtain high-quality pyrolysis liquid products, the effects of different calcium-based catalysts and temperatures on liquid products from catalytic pyrolysis of municipal sludge (moisture of 50%) were studied. Results showed that the liquid product yield reached maximum at 600 °C and then decreased. The catalyst capability of catalysts decreased the yield of liquid products in the order of poorly soluble in water (Ca(OH)2, CaCO3, CaO) > soluble in water (CaCl2, Ca(H2PO4)2·H2O). High temperature strengthened the aromatization, and the breaking of the oxygen-containing functional group in liquid products resulted in the decrease of O/C and H/C molar ratios and the increase of four components yield in gaseous products. At 600 °C, the catalysts significantly reduced the O content of liquid products and increased hydrocarbon content. Meantime, CaO additive increased the content of C to 85.34%, and the relative content of aromatics reached 83.99% at 800 °C, which is suitable as chemical raw materials. However, the Ca(OH)2 group had the highest aliphatics content, and the H/C molar ratio was 1.42 at 400 °C, which is suitable as fuel.
Similar content being viewed by others
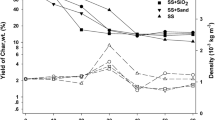
Explore related subjects
Discover the latest articles, news and stories from top researchers in related subjects.Avoid common mistakes on your manuscript.
Introduction
The production of municipal sludge (moisture of 80%) in China increased from 35 million tons in 2015 to exceeded 40 million tons in 2018 [1] and will probably reach 60–90 million tons in 2020 [2]. At present, the major treatment methods of municipal sludge are landfill, composting, and incineration in China [3]. However, these methods have some drawbacks, including the occupation of large amounts of land, excessive levels of heavy metals, and emission of pollutants [4,5,6]. Therefore, it is necessary to develop an environment-friendly and recyclable technology.
Pyrolysis is a promising technique that involves thermal cracking of organic material in the absence of oxygen at high temperature into three-phase products of solid products (char), gaseous products (bio-gas), and liquid products (bio-oil) to realize “reduction, harmlessness, resource” [7]. The char has high calorific value and porous structure, which is suitable for fuels combusting with coal and soil amendment [8]. The bio-gas can be used as fuel for power generation due to it contains hydrogen, methane, and other combustible gases [9]. The liquid products can be used to extract chemical raw materials or further upgrade into fuels [10, 11] because it contains a large number of organic components [12].
Nevertheless, the liquid products of sludge pyrolysis are inferior quality. The utilization of liquid products is limited by high oxygen content, instability, and complex components [13]. Thus, there is more attention to improve the quality and quantity of liquid products by adding catalysts [14, 15]. Recently, metal and zeolite catalysts with high catalytic performance have been widely studied. Wang et al. [16] studied the effect of char on pyrolysis liquid products and found the quality of liquid products to be improved in presence of alkali and alkaline earth metallic. Zhao et al. indicted that alkaline earth metals (AAEMs) in char promoted the degradation of heavy tar [17] and iron ore significantly increased the yield of light tar and light aromatic hydrocarbons [18]. In addition, many studies showed that zeolite-supported metal catalyst could reduce the oxygen content in pyrolysis oil [19], improve the selectivity of total aromatics [20, 21], and increase the yield of pyrolysis gas [22]. According to previous researches, many metallic elements exhibited significant effects on the distribution and quality of liquid products during pyrolysis process.
AAEMs are the most attractive metal catalysts recently [23, 24]. However, catalysts are easy to coking and lead to deactivation in the catalytic pyrolysis process. Therefore, there is an urgent need to develop low-cost catalysts. Ca is relatively inexpensive compared with Co and Ni, which has attracted more attention. Shie et al. [25,26,27] investigated the effect of Al species, Ca species, Na species, and K species on liquid products from catalytic pyrolysis of sludge. The study found that Al species significantly increased the yield of liquid products, while Ca species, Na species, and K species improved the quality of liquid products. Chen et al. [28] studied the influence of CaO on liquid products composition of different waste pyrolysis. The results showed that the addition of CaO reduced tar yield, and the concentration of organic acid decreased with the increase of CaO content. The existing form of metals has important effect on the distribution and composition of pyrolysis products, while the activity of metal catalysts is affected by pyrolysis temperature [29].
Herein, this research is intended to systematically study the effect of different calcium-based catalysts (Ca(OH)2, CaCO3, CaCl2, CaO, Ca(H2PO4)2·H2O) at different temperatures on yield and composition evolution of pyrolysis liquid products. The pyrolysis products were comprehensively characterized to reveal the pyrolysis mechanism at different conditions and look for a suitable catalyst used for sludge pyrolysis.
Materials and Methods
Materials
Municipal sludge (70% of moisture) from Chang Shanyuan sewage disposal plant (Changsha, Hunan Province, China) underwent dried preservation. And then, the municipal sludge was crushed with a high-speed grinder and sieved with a 100-mesh. Subsequently, distilled water was added to make the form sludge with a moisture content of 50% as raw material. According to the relevant national standards of China, the moisture content of sludge should be less than 60% before it can be disposed in municipal solid waste landfill. According to the investigation, the moisture content of the sludge after treatment is mostly 50% in Changsha. Meantime, the sludge with the moisture content of 50% is selected as the experimental raw material in combination with a project we undertook. Ca(OH)2, CaCO3, CaCl2, CaO, and Ca(H2PO4)2·H2O were used as catalysts in the experiments. They all are analytical reagent. Calcium ion in five kinds of ionic compound catalysts are based on the combination of ionic bond; the bond energy was in the order of CaO > CaCO3 > CaCl2 > Ca(OH)2 > Ca(H2PO4)2·H2O. These five catalysts can be divided into soluble in water (CaCl2, Ca(H2PO4)2·H2O) and poorly soluble in water (Ca(OH)2, CaCO3, CaO). These catalysts were mixed with municipal sludge, and the experimental groups were divided into addition groups and blank group. The addition groups termed Ca(OH)2, CaCO3, CaCl2, CaO, and Ca(H2PO4)2, respectively. The blank group of municipal sludge without catalysts was termed MS.
The proximate analysis of sample (dry basis) was measured according to the procedure described in previous work [30]. The contents of ash, volatile matter, and fixed carbon (by difference) were 65.9 wt.%, 31.7 wt.%, and 2.4 wt.%, respectively. The results of ultimate analysis (dry basis) shown that the contents of C, H, O (by difference), N, and S were 15.4 wt.%, 2.7 wt.%, 15.8 wt.%, 1.9 wt.%, and 0.6 wt.%, respectively.
Experimental Equipment and Conditions
The equipment for our experiment is presented in Fig. S1. In this experiment, 0.8 g of Ca(OH)2, CaCO3, CaCl2, CaO, and Ca(H2PO4)2·H2O were mixed with 40 g of raw material (50% of moisture), respectively, which spread evenly in sample tank placed in quartz tube reactor. The air in the quartz tube was completely discharged by a vacuum pump. The desired temperatures were 400 °C, 600 °C, and 800 °C with a heating rate of 10 °C/min. The residence time was 1 h, and the nitrogen flow rate was 100 mL/min. The liquid products were collected and kept by condensing. CH2Cl2 was added for extraction. After full oscillation and ultrasonic treatment for 20 min, it was transferred to the separatory funnel and taken the lower organic phase stored in 4 °C freezer. Before being tested, the samples were taken out until reached room temperature. Then, they were filtered with 0.45-μm polyvinylidene fluoride filter and measured by GC-MS. A parallel test was conducted to reduce the error. The gaseous products were collected by gas bag and then were analyzed by GC. The solid product was mixed with the catalyst and was difficult to separate from the catalyst. The mass of solid product was calculated by the change of the mass of quartz tube and catalyst before and after reaction. The yield of three phase products from the pyrolysis was calculated as follows.
Analytical Methods
The contents of C, H, O, and N in pyrolysis liquid products were measured by an elemental analyzer (vario EL cube, Germany). The components of liquid products were analyzed by a 6890N-5975B type GC-MS (Agilent, America), equipped with a capillary column (30 m × 0.25 mm inner diameter, with 0.25 μm film thickness, Restek). The GC injector port temperature was at 250 °C. The column oven temperature was 80 °C. The ion temperature was 200 °C with a flow split ratio of 30:1. The column oven temperature was increased from 80 °C to 250 °C at a heating rate of 5 °C/min. The scan mode was scan, the scanned area from m/z 50 to 500, and the solvent interval was 3 min. The gaseous products were analyzed by GC-5890N gas chromatograph (Nanjing kejie analysis instrument Co. Ltd.), equipped with a thermal conductivity detector. The solid products were analyzed by FTIR (ALPHA, Bruker).
Results and Discussion
Distribution of Sludge Catalytic Pyrolysis Three-Phase Products
The distribution of catalytic pyrolysis products at different temperatures are shown in Fig. S2. Catalysts and pyrolysis temperature had great influence on products distribution of the sludge pyrolysis. With the temperature increased from 400 °C to 800 °C, the solid product yield decreased from about 40% to about 34% [31], while the gaseous product yield increased from about 5% to about 9.5% and the liquid product yield reached maximizes at 600 °C and then decreased. The high moisture (50%) of feedstock resulted in high liquid product content. The results indicated that more organic substances in sludge were cracked through devolatilization reaction, and the liquid products underwent secondary cracking for producing gas with the temperature increased. The addition increased the yield of solid products, especially for Ca(H2PO4)2. This suggested that thermally stable complexes were formed and inhibited the carbon decomposition [32]. The yield of liquid products decreased, while the yield of gaseous products increased in all addition groups compared with MS group. It suggests that the catalysts also strengthened secondary cracking of volatiles to produce more gaseous products [33,34,35]. The catalytic effect was ranked as such: poorly soluble in water (Ca(OH)2, CaCO3, CaO) > soluble in water (CaCl2, Ca(H2PO4)2·H2O).
Analysis of Liquid Products of Sludge Catalytic Pyrolysis
Elemental Analysis of Liquid Phase Products
Elemental analysis was performed on the liquid products from different pyrolysis conditions, and the results are presented in Tables 1, 2, and 3.
According to Table 1, the content of O increased in each addition group compared with MS group at 400 °C, while the content of C decreased. The O content of CaCl2 group and Ca(OH)2 group reached 15.94% and 14.10%, while the C content decreased to 73.58% and 71.10%, respectively. The O/C molar ratios were also the highest among all groups, with 0.16 and 0.15, respectively. This indicated that the metal compound of each group could promote the release of O in sludge [36], which might be due to metal ions promoted the fracture of oxygen-containing functional groups. The H/C molar ratios of Ca(OH)2 group (1.42) was the highest at 400 °C, which demonstrated that Ca(OH)2 was beneficial to increase the saturation and calorific value of liquid products [37, 38].
As shown in Table 2, the content of C in each addition group was higher than that in MS group at 600 °C. Among them, the C content of CaCl2 group was the highest (81.96%). The O content of the other groups were lower compared with MS group. The O content (4.69%) and O/C molar ratio (0.04) of CaCl2 group were the lowest. The results showed that the catalysts promoted the secondary cracking of organic macromolecule [39] and the breaking of oxygen-containing functional group [40], which reduced the O content of the liquid products. In addition, the N content of addition groups were higher than that of MS group, which indicated the catalysts promoted N in the sludge transferred to liquid products.
According to Table 3, the highest C content and the lowest O content at 800 °C was CaO group with 85.34% and 3.12%, respectively, which had been highly carbide. The results indicated CaO promoted deoxidation and carbonization. In addition, the N content of each additive group was lower than MS group. It suggested that the catalysts promoted the denitrification of liquid products.
In summary, the C content of each group had a great increase with the temperature increased to 800 °C. The C content of all groups exceeded 81.44%. This inferred that the high temperature was favorable for carbonization of liquid products. The O content of the all groups decreased with the increase of temperature. The results indicated that high temperature could reduce the O content of liquid products. The H/C and O/C molar ratio of each group decreased with the increase of temperature. The results indicated that decarboxylation and dehydration occurred at high temperature.
Composition Analysis of Liquid Products
The components of liquid products from municipal sludge were complex, which were determined by the GC-MS. The liquid product components were classified into aliphatic compounds (aliphatics), aromatic compounds (aromatics), and heterocyclic compounds (heterocyclics), and the results are presented in Figs. 1, 2, and 3. Hydrocarbon components of liquid products were divided into monocyclic aromatic hydrocarbons, polycyclic aromatic hydrocarbons (PAHs), alkanes, and olefins, and the results are shown in Figs. 4, 5, and 6.
Figure 1 shows that Ca(H2PO4)2, CaCl2, and Ca(OH)2 could reduce the content of aromatics at 400 °C. The concentration of aromatics was the lowest (32.34%), and the concentration of aliphatics was the highest (53.20%) in Ca(OH)2 group, which is suitable as fuel. The results could be attribute to Ca(OH)2 dissociate to offer H+, which reacted with aromatics and resulted in ring-opining [41]. In contrast, the aromatics content and heterocyclics content of CaO and CaCO3 group increased compared with MS. It might be due to CaO and CaCO3 promoted cyclization to form heterocyclics. These results indicated that the strength of ionic bond may affect the yield of aromatic group and the catalysts with relatively weak ionic bonds can decrease the yield of aromatic compounds at 400 °C.
Figure 2 shows the alkanes content of addition groups significantly decreased compared with MS group at 400 °C. The results might be attributed to the interaction of bivalent metal cation in the catalyst with π electron system of volatiles, resulting in the organism unstable and break the C-C bond easier [42]. It could provide active sites for the reaction with atoms such as N and O to reduce the hydrocarbon content. Song et al. [43] found that lattice defects induced the oxygen anion migrating, bringing about the adsorption of benzene. PAHs and alkanes contents of addition groups were significantly reduced, which might be related to the catalyst that was favorable for breaking C-C bonds and O atoms to replace more branched chains on the benzene ring.
As shown in Fig. 3, the aliphatics content of the other groups were lower except CaCO3 group (39.18%) at 600 °C. The aliphatics content of Ca(H2PO4)2 group reduced to 7.30 from 53.19% with the temperature from 400 increased to 600 °C, while aromatics content improved significantly to 81.46% and heterocyclics were almost unchanged. The results suggested that Ca(H2PO4)2 promoted the aromatization of aliphatics and generated a large number of aromatics at 600 °C. Meantime, the heterocyclics contents in Ca(OH)2 (32.42%) and CaCl2 (14.3%) groups significantly increased, while aliphatics reduced significantly at 600 °C compared with 400 °C. In addition, aromatics content (75.96%) of CaCl2 group increased sharply. This proved that CaCl2 was conducive to the formation of aromatics and Ca(OH)2 promoted aliphatics convert into heterocyclics.
Figure 4 shows the hydrocarbon distribution of liquid products at 600 °C. The contents of PAHs, alkanes, and olefins of MS group reduced significantly compared with 400 °C. According to the former reports, the aliphatic hydrocarbon bond of municipal sludge was opened entirely as the terminal temperature reaction up to 550 °C [44]. The alkenes were partially oxidized and might react with a large number of dienes; plenty of aromatics with O-containing functional groups were generated finally. Compared with the MS group, the contents of hydrocarbons in the liquid products of the additive groups were higher. The content of PAHs increased significantly, and the content of alkanes and olefin also increased. The results might be mainly due to catalysts promoted the fracture of branch chains and substituents on the benzene ring.
As shown in Fig. 5, the aromatics content of Ca(OH)2 group was the lowest. It indicated that Ca(OH)2 inhibited the formation of aromatics at 800 °C. Except Ca(OH)2 group, aromatics content of the others exceeded 70%, and the catalysts with relatively weak ionic bonds can decrease the yield of aromatic compounds. The catalytic effects of catalysts with relatively weak ionic bonds at 400 °C and 800 °C were the opposite of 600 °C. In particular, the aromatics content of CaO group was significantly increased to 83.99%, and the heterocycles content was significantly reduced to 4.10%. This could be attributed to the electron density of carbon atoms of ring increased by heteroatom; the ring was activated, and the stability was lower than benzene ring. This indicated that heterocyclics such as furan and pyrrole might occur Diels-Alder reaction, and a mass of heterocyclics were converted to aromatics. In addition, compared with MS group, aliphatics content of addition groups increased greatly, while heterocycles declined significantly. The results indicated that the catalysts promoted the ring-opening reaction of N-containing heterocycles and then reacted with oxygen to form O-containing aliphatics [22].
As shown in Fig. 6, except Ca(OH)2 group, hydrocarbons of other groups increased significantly at 800 °C. The hydrocarbons content of CaO group reached 52.30%, which showed CaO promoted the fracture of various O-containing and N-containing functional groups, and the O and N in the liquid products entered the gaseous products. This is also the reason for the low oxygen content of the CaO group.
To sum up, aliphatic content of each experimental group was high at 400 °C, while high temperature enhanced aromatization reaction resulted in rapid rise of aromatics content. It also illustrated that the H/C molar ratio of each group generally decreased at high temperature by olefin cyclization and aromatization dehydrogenation. In addition, the overall trend of heterocyclics content was downward with the temperature increase. However, the hydrocarbon (mainly PHAs) content was rising with the temperature increase. The result indicated that high temperature promoted the aromatization of olefin and the removal of heteroatoms from heterocyclic rings, which was conducive to the generation of hydrocarbons. Meantime, the catalyst exhibited different catalytic properties at different temperature. The catalyst inhibited PHAs and promoted heterocyclics at low temperature, while the catalyst promoted PHAs and inhibited heterocyclics at high temperature. Moreover, the percentage of aromatics in CaO group was as high as 83.99% at 800 °C, which is suitable for chemical raw materials. This might be due to high ash content of municipal sludge [45] and CaO [46], which could promote aromatics content. The proportion of aliphatics and the molar ratio of H/C in liquid products were large when CaCl2, Ca(H2PO4)2, and Ca(OH)2 were used as catalysts at 400 °C, which is suitable as liquid fuel.
Composition Analysis of Gaseous and Solid Products
To further reveal details of the decomposition of sludge. The analysis results of gaseous products are shown in Fig. S3, Fig. S4, and Fig. S5. CxHyOz can be represented as municipal sludge, and the pyrolysis reaction could happen as follows:
The reaction process of pyrolysis products in Eq. (1) may be as follows:
As Fig. S3 shows, the relative content of CO2 was more than 50% in each group at 400 °C. It could be attributed to decarboxylation at the low temperature. The decrease of O content in gaseous products demonstrated more O transferred to liquid products. The relative content of H2 in CaO group (38.45%) increased significantly compared with MS group. This was because that CaO reacted with CO2 prompted the water–gas shift reaction Eq. (7) to produce more H2 [47]. The catalysts of soluble in water promote CO2 and inhibit hydrogen compared with poorly soluble in water.
As Fig. S4 shows, CaCl2 and Ca(H2PO4)2 inhibited the formation of CO2. In addition, CaCl2 promoted methanation reaction Eq. (8) and resulted in the relative content of CH4, which was higher than MS group. The relative content of H2 in Ca(H2PO4)2 group and Ca(OH)2 group reached 45.44% and 44.59%. This could be because these catalysts with relatively weak ionic promoted Eq. (6), more hydrogen transferred to gaseous products.
Figure S5 shows the component distribution of gaseous products of sludge at 800 °C. The accumulation of H2 in each group exceeded CO2, and H2 content increased with the increase of temperature [48]. It demonstrated that these catalysts promoted aromatization of liquid products resulted in dehydrogenation. The contents of CO2 and CO in CaO group were higher relative. It meant CaO was more favorable to decarbonylation and decarboxylation. CaCl2 and Ca(H2PO4)2 increased the content of CO2 and decreased the contents of CO and H2, which promoted Eqs. (4) and (5).
In sum, the relative contents of CO2 were lower, while the relative content of H2 and CH4 were higher with the increase of temperature. This might be related to the secondary cracking of organic macromolecules and aromatization, which could generate more H radicals and then produced H2 and CH4. The catalysts of soluble in water inhibited the formation of CO2 at high temperature, which might be due to more hydrated calcium ion by hydration and better diffusion compared with poorly soluble in water. Figures S6–S8 show that with the rise of temperature, the actual production of H2, CO, and CH4 increased even though the relative content of H2, CO, and CH4 decreased slightly under different conditions. Moreover, even the actual production of CO2 increased with the increase of temperature. It meant high temperatures increased the production of four components in gaseous products.
Figures S9–S11 show that the surface of solid products is rich in functional groups, and the strong absorption peaks of 1030 cm−1 certify the existence of C-O and C-O-C. The catalyst reduced the peak strength and promotes the release of oxygen at 400 °C, while catalyst increased the peak strength and promoted the formation of oxygen-containing functional groups on the surface of solid products at 600 °C. The decrease of peak number indicates that pyrolysis leads to the decrease of functional groups on the surface and promoted the yield of gaseous products.
Conclusion
In this paper, the quantity and quality of liquid products and gaseous products from catalytic pyrolysis of municipal sludge (moisture of 50%) were studied. The existence forms of calcium ion and temperature had a great impact on the pyrolysis process. The high temperature and catalysts strengthened secondary cracking of volatiles, resulted in the liquid product yield, reached maximizes at 600 °C, and then decreased. The catalytic effect was ranked as such: poorly soluble in water (Ca(OH)2, CaCO3, CaO) > soluble in water (CaCl2, Ca(H2PO4)2·H2O).
At 400 °C, the catalysts decreased the hydrocarbon content and increased the O content in liquid product. However, high temperature promoted the aromatization and polymerized of olefin, and the breaking of the oxygen-containing functional group resulted in the increase of hydrocarbon content and the decreased of O content in liquid product. Moreover, the catalysts promote the fracture of branched chain and the substituent groups on the benzene ring at 600 °C and 800 °C, generating more hydrocarbons (mainly PHAs) compared with MS group.
The content of aromatics in liquid product was 83.99% at 800 °C, which is suitable as chemical raw materials. At 400 °C, the Ca(OH)2 group had the highest aliphatics content, and the H/C molar ratio was 1.42, which is suitable as fuel.
References
Feng LY, Luo JY, Chen YG (2015) Dilemma of sewage sludge treatment and disposal in China. Environ Sci Technol 49(8):4781–4782. https://doi.org/10.1021/acs.est.5b01455
Wang Z, Ma X, Yao Z et al (2018) Study of the pyrolysis of municipal sludge in N2/CO2 atmosphere. Appl Therm Eng 128:662–671. https://doi.org/10.1016/j.applthermaleng.2017.09.044
Han H, Hu S, Syed-Hassan SSA, Xiao Y, Wang Y, Xu J, Jiang L, Su S, Xiang J (2017) Effects of reaction conditions on the emission behaviors of arsenic, cadmium and lead during sewage sludge pyrolysis. Bioresour Technol 236:138–145. https://doi.org/10.1016/j.biortech.2017.03.112
Hospido A, Carballa M, Moreira M, Omil F, Lema JM, Feijoo G (2010) Environmental assessment of anaerobically digested sludge reuse in agriculture: potential impacts of emerging micropollutants. Water Res 44:3225–3233. https://doi.org/10.1016/j.watres.2010.03.004
Liu HT, Wang YW, Huang WD, Lei M (2016) Response of wine grape growth, development and the transfer of copper, lead, and cadmium in soil-fruit system to sludge compost amendment. Environ Sci Pollut Res 23:24230–24236. https://doi.org/10.1007/s11356-016-7676-z
Liu HT, Wang YW, Liu XJ et al (2017) Reduction in greenhouse gas emissions from sludge biodrying instead of heat drying combined with mono-incineration in China. J Air Waste Manage Assoc 67(2):212–218. https://doi.org/10.1080/10962247.2016.1227282
Agar DA, Kwapinska M, Leahy JJ (2018) Pyrolysis of wastewater sludge and composted organic fines from municipal solid waste: laboratory reactor characterisation and product distribution. Environ Sci Pollut Res 12:1–9. https://doi.org/10.1007/s11356-018-1463-y
Hu BH, Huang QX, Bourtsalas ACT et al (2017) Effect of chlorine on the structure and reactivity of char derived from solid waste. Energy Fuel 31(7):7606–7616. https://doi.org/10.1021/acs.energyfuels.7b01042
Yu G, Chen D, Arena U et al (2018) Reforming sewage sludge pyrolysis volatile with Fe-embedded char: minimization of liquid product yield. Waste Manag 73:464–475. https://doi.org/10.1016/j.wasman.2017.08.004
Rahman MM, Liu RH, Cai JM (2018) Catalytic fast pyrolysis of biomass over zeolites for high quality bio-oil – a review. Fuel Process Technol 180:32–46. https://doi.org/10.1016/j.fuproc.2018.08.002
Wang Y, Dai L, Fan L et al (2017) Catalytic co-pyrolysis of waste vegetable oil and high density polyethylene for hydrocarbon fuel production. Waste Manag 61:276–282. https://doi.org/10.1016/j.wasman.2017.01.010
Chiang HL, Lin KH, Lai N et al (2014) Element and PAH constituents in the residues and liquid oil from biosludge pyrolysis in an electrical thermal furnace. Sci Total Environ 481(2):533–541. https://doi.org/10.1016/j.scitotenv.2014.02.083
Mante OD, Agblevor FA, Oyama ST, McClung R (2012) The influence of recycling non-condensable gases in the fractional catalytic pyrolysis of biomass. Bioresour Technol 111:482–490. https://doi.org/10.1016/j.biortech.2012.02.015
Cheng S, Wang Y, Fumitake T et al (2017) Effect of steam and oil sludge ash additive on theproducts of oil sludge pyrolysis. Appl Energy 185:146–157. https://doi.org/10.1016/j.apenergy.2016.10.055
Kalogiannis KG, Stefanidis SD, Lappas AA (2019) Catalyst deactivation, ash accumulation and bio-oil deoxygenation during ex situ catalytic fast pyrolysis of biomass in a cascade thermal-catalytic reactor system. Fuel Process Technol 186:99–109. https://doi.org/10.1016/j.fuproc.2018.12.008
Wang N, Chen DZ, Arena U et al (2017) Hot char-catalytic reforming of volatiles from MSW pyrolysis. Appl Energy 191:111–124. https://doi.org/10.1016/j.apenergy.2017.01.051
Zhao HY, Song Q, Liu SC et al (2018) Study on catalytic co-pyrolysis of physical mixture/staged pyrolysis characteristics of lignite and straw over an catalytic beds of char and its mechanism. Energy Convers Manag 161:13–26. https://doi.org/10.1016/j.enconman.2018.01.083
Zhao HY, Li YH, Song Q et al (2019) Catalytic reforming of volatiles from co-pyrolysis of lignite blended with corn straw over three different structures of iron ores. J Anal Appl Pyrolysis 144:104714. https://doi.org/10.1016/j.jaap.2019.104714
Veses A, Puértolas B, López JM et al (2016) Promoting deoxygenation of bio-oil by metal-loaded hierarchical ZSM-5 zeolites. ACS Sustain Chem Eng 4:1653–1660. https://doi.org/10.1021/acssuschemeng.5b01606
Valle B, Gayubo AG, Alonso A et al (2010) Hydrothermally stable HZSM-5 zeolite catalysts for the transformation of crude bio-oil into hydrocarbons. Appl Catal B-Environ 100(1):318–327. https://doi.org/10.1016/j.apcatb.2010.08.008
Lin B, Wang J, Huang Q et al (2017) Aromatic recovery from distillate oil of oily sludge through catalytic pyrolysis over Zn modified HZSM-5 zeolites. J Anal Appl Pyrolysis 128:291–303. https://doi.org/10.1016/j.jaap.2017.09.021
Gu B, Cao JP, Fu W et al (2019) Nitrogen migration mechanism and formation of aromatics during catalytic fast pyrolysis of sewage sludge over metal-loaded HZSM-5. Fuel 244:151–158. https://doi.org/10.1016/j.fuel.2019.02.005
Yang Z, Cao JP, Ren XY et al (2019) Preparation of hierarchical HZSM-5 based sulfated zirconium solid acid catalyst for catalytic upgrading of pyrolysis vapors from lignite pyrolysis. Fuel 237:1079–1085. https://doi.org/10.1016/j.fuel.2018.10.049
Iliopoulou EF, Stefanidis SD, Kalogiannis KG et al (2012) Catalytic upgrading of biomass pyrolysis vapors using transition metal-modified ZSM-5 zeolite. Appl Catal B-Environ 127:281–290. https://doi.org/10.1016/j.apcatb.2012.08.030
Shie JL, Lin JP, Chang CY et al (2002) Use of calcium compouds as additives for oil sludge pyrolysis. J Chin Inst Environ Eng 12(4):363–371
Shie JL, Lin JP, Chang CY et al (2003) Pyrolysis of oil sludge with additives of sodium and potassium compounds. Resour Conserv 39(1):51–64. https://doi.org/10.1016/S0921-3449(02)00120-9
Shie JL, Lin JP, Chang CY et al (2004) Pyrolysis of oil sludge with additives of catalytic solid wastes. J Anal Appl Pyrolysis 71(2):695–707. https://doi.org/10.1016/j.jaap.2003.10.001
Chen X, Chen YQ, Yang HP, Chen W, Wang X, Chen H (2017) Fast pyrolysis of cotton stalk biomass using calcium oxide. Bioresour Technol 233:15–20. https://doi.org/10.1016/j.biortech.2017.02.070
Li X, Wu H, Hayashi JI et al (2004) Volatilisation and catalytic effects of alkali and alkaline earth metallic species during the pyrolysis and gasification of Victorian brown coal Part VI Further investigation into the effects of volatile-char interactions. Fuel 83(10):1273–1279. https://doi.org/10.1016/S0016-2361(01)00127-2
Eke J, Onwudili JA, Bridgwater AV (2017) Physical pretreatment of biogenic-rich trommel fines for fast pyrolysis. Waste Manag 70:81–90. https://doi.org/10.1016/j.wasman.2017.09.009
Liao R, Gao B, Fang J (2013) Invasive plants as feedstock for biochar and bioenergy production. Bioresour Technol 140:439–442. https://doi.org/10.1016/j.biortech.2013.04.117
Xu X, Hu X, Ding Z, Chen Y (2017) Effects of copyrolysis of sludge with calcium carbonate and calcium hydrogen phosphate on chemical stability of carbon and release of toxic elements in the resultant biochars. Chemosphere 189:76–85. https://doi.org/10.1016/j.chemosphere.2017.09.021
Loannidou O, Zabaniotou A, Antonakou EV et al (2009) Investigating the potential for energy, fuel, materials and chemicals production from corn residues (cobs and stalks) by non-catalytic and catalytic pyrolysis in two reactor configurations. Renew Sust Energ Rev 13:750–762. https://doi.org/10.1016/j.rser.2008.01.004
Xie Q, Peng P, Liu S, Min M, Cheng Y, Wan Y, Li Y, Lin X, Liu Y, Chen P, Ruan R (2014) Fast microwave-assisted catalytic pyrolysis of sewage sludge for bio-oil production. Bioresour Technol 172:162–168. https://doi.org/10.1016/j.biortech.2014.09.006
Alvarez J, Amutio M, Lopez G et al (2015) Fast co-pyrolysis of sewage sludge and lignocellulosic biomass in a conical spouted bed reactor. Fuel 159:810–818. https://doi.org/10.1016/j.fuel.2015.07.039
Khan MR, Seshadri K (1991) Compositional changes in the mild gasification liquids produced in the presence of calcium compounds. Fuel Process Technol 27(1):83–94. https://doi.org/10.1016/0378-3820(91)90010-A
Ellis N, Masnadi MS, Roberts DG et al (2015) Mineral matter interactions during co-pyrolysis of coal and biomass and their impact on intrinsic char co-gasification reactivity. Chem Eng J 279(1):402–408. https://doi.org/10.1016/j.cej.2015.05.057
Jones JM, Kubacki M, Kubica K et al (2005) Devolatilisation characteristics of coal and biomass blends. J Anal Appl Pyrolysis 74(1–2):502–511. https://doi.org/10.1016/j.jaap.2004.11.018
He M, Xiao B, Liu S et al (2010) Syngas production from pyrolysis of municipal solid waste (MSW) with dolomite as downstream catalysts. J Anal Appl Pyrolysis 87(2):181–187. https://doi.org/10.1016/j.jaap.2009.11.005
Fonts I, Gea G, Azuara M et al (2012) Sewage sludge pyrolysis for liquid production: a review. Renew Sust Energ Rev 16(5):2781–2805. https://doi.org/10.1016/j.rser.2012.02.070
Liu H, Zhang Q, Hu H et al (2014) Dual role of conditioner CaO in product distributions and sulfur transformation during sewage sludge pyrolysis. Fuel 134:514–520. https://doi.org/10.1016/j.fuel.2014.06.020
Ma R, Huang X, Zhou Y, Fang L, Sun S, Zhang P, Zhang X, Zhao X (2017) The effects of catalysts on the conversion of organic matter and bio-fuel production in the microwave pyrolysis of sludge at different temperatures. Bioresour Technol 238:616–623. https://doi.org/10.1016/j.biortech.2017.04.103
Song Q, Zhao HY, Xing WL et al (2018) Effects of various additives on the pyrolysis characteristics of municipal solid waste. Waste Manag 78:621–629. https://doi.org/10.1016/j.wasman.2018.06.033
Shi L, Liu Q, Zhou B et al (2016) Interpretation of methane and hydrogen evolution in coal pyrolysis from the bond cleavage perspective. Energy Fuel 31(1):429–437. https://doi.org/10.1021/acs.energyfuels.6b02482
Domínguez A, Menendez JA, Inguanzo M et al (2003) Gas chromatographic- mass spectrometric study of the oil fractions produced by microwave-assisted pyrolysis of different sewage sludges. J Chromatogr A 1012(2):193–206. https://doi.org/10.1016/S0021-9673(03)01176-2
Dı́ez MA, Domı́nguez A, Barriocanal C et al (1999) Hydrogen donor and acceptor abilities of pitches from coal and petroleum evaluated by gas chromatography. J Chromatogr A 830(1):155–164. https://doi.org/10.1016/s0021-9673(98)00900-5
Tang S, Zheng C, Yan F et al (2018) Product characteristics and kinetics of sewage sludge pyrolysis driven by alkaline earth metals. Energy 153:921–932. https://doi.org/10.1016/j.energy.2018.04.108
Sun Y, Chen J, Zhang Z (2019) General roles of sludge ash, CaO and Al2O3 on the sludge pyrolysis toward clean utilizations. Appl Energy 233-234:412–423. https://doi.org/10.1016/j.apenergy.2018.10.034
Acknowledgments
The authors would like to thank the Lab and Engineering staff at MEE for their invaluable support through the project.
Funding
This work was financially supported by key Research and Development Project of Hunan Province (No. 2017SK2354), Science and Technology project of Changsha (No. kh1801219), National Natural Science Foundation of China (Grant No. 21706060, 51,703,061), Natural Sciences Foundation of Hunan Province, China (Grant No. 2017JJ3103), Scientific Research Foundation of Hunan Provincial Education Department (Grant No. 17B118), and Hunan Provincial Engineering Technology Research Center for Optical Agriculture (Grant No. 2018TP2003).
Author information
Authors and Affiliations
Corresponding author
Additional information
Publisher’s Note
Springer Nature remains neutral with regard to jurisdictional claims in published maps and institutional affiliations.
Professor Zhang provided important Suggestions for the revision of the article.
Electronic Supplementary Material
ESM 1
(DOCX 2034 kb)
Rights and permissions
About this article
Cite this article
Luo, W., Su, YF., Hu, Q. et al. Effect of Calcium-Based Catalysts on Pyrolysis Liquid Products from Municipal Sludge. Bioenerg. Res. 13, 887–895 (2020). https://doi.org/10.1007/s12155-020-10109-8
Published:
Issue Date:
DOI: https://doi.org/10.1007/s12155-020-10109-8