Abstract
The interspecific hybridization for tomato leaf curl virus (ToLCV) resistance was carried out among 10 genetically diverse tomato genotypes (diversified by 50 SSR markers). Among the 10 parents, four susceptible cultivars of Solanum lycopersicum were crossed with six resistant wilds, such as S. pimpinellifolium, S. habrochaites, S. chemielewskii, S. ceraseforme, S. peruvianum and S. chilense in a line × tester mating design. All the 24 hybrids and their parents were grown in the field and glasshouse conditions to determine the general combining-abilities (GCA) and specific-combining abilities (SCA). The variances due to SCA and GCA showed both additive and nonadditive gene effects. Based on GCA estimates, EC-520061 and WIR-5032 were good general combiners while based on SCA estimates, PBC × EC-520061 and PBC × EC-521080 were best specific combiners for coefficient of infection and fruit yield per plant in both the environments. These lines could be selected and utilized in ToLCV resistance and high yield breeding programme for improving the traits.
Similar content being viewed by others
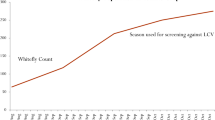
Avoid common mistakes on your manuscript.
Introduction
Tomato (S. lycopersicum) is a herbaceous, usually sprawling plant of Solanum species that is typically cultivated for human consumption, like vegetables as well as fruits (Chomdej et al. 2008). Generally, wild species of tomato are polymorphic and most of the interspecific hybrids expressed their morphological attributes from wild taxa over the lycopersicum types (Chen et al. 2011). There is tremendous morphological variation among and within the species which provides a rationale to make the interspecific hybridization a success. If one accession of a species is not compatible, the other may be compatible and can be hybridized so there is a possibility of getting hybrid seeds in some combinations. F1 hybrids of S. lycopersicum × S. habrochaites f. glaboratum, S. lycopersicum × S. pimpinellifoliumand S. lycopersicum × S. cheesmanii were morphologically almost similar to their respective wild parents (Kalloo and Banerjee 1989; Chen et al. 2011). Molecular diversity through SSRs markers has been recently made achieving tools for identifying the interspecific relationship (Frary et al. 2005). Many genetic maps consisting molecular markers have been developed in recent years for many crop plants (Wu et al. 2009; Lioi and Galasso 2013).
Since ages, tomato leaf curl virus disease (ToLCV) has been a severe problem in tomato production causing up to 100% yield losses worldwide, especially in those areas where tomato is grown commercially (Singh et al. 2010; Banerjee and Kalloo 1987). To date no cultivars/hybrids are stable for resistance to ToLCV for a long duration, except for a number of wild species and their hybrids (Kalloo and Banerjee 1989; Chen et al. 2011). The quantitative characteristics and ToLCV resistance are controlled by a large number of genes and are strongly influenced by the environmental factors (Ahmad et al. 2009). Therefore, it is desirable to make a strict individual plant selection on quantitative characters which could be useful for combining ability (CA). Although, combining ability studies have been more reliable in providing useful information for the selection of diverse parents with regard to performance of the resistant hybrids, while elucidating the nature and magnitude of various types of gene effects involved in the expression of quantitative traits (with good-quality traits), production of resistant hybrids has been limited due to lack of superior combiners (Ahmad et al. 2009). Genetic analysis provides a guideline to assess the relative breeding potential of parents or identify best combiners in crop (Ahmad et al. 2009), which can be utilized either to exploit the combining ability in F1’s or accumulate fixable genes to evolve a variety. Entire genetic variability observed in the analysis for each trait was partitioned into components, i.e. GCA and SCA as defined by Sprague (1966) with additive and nonadditive gene actions, respectively. In this study, the diverse tomato parents are used for interspecific hybridization to identify good combiners for ToLCV resistance and yield components under two different environments.
Materials and methods
The experimental materials comprised of 10 diverse tomato genotypes of different species. Four cultivars (Punjab Chhuhara (PBC), Kashi Vishesh (H-86), Hissar Anmol (H-24) and Kashi Anupam (DVRT-2) of S. lycopersicum showed determined plant growth, high yield with superior fruit quality; while six wild species (EC-521080 (S. pimpinellifolium), EC-520061 (S. habrochaites), EC-520049 (S. chmielewskii), EC-528372 (S. cerasiforme), WIR-3957 (Solanum peruvianum) and WIR-5032 (S. chilense)) displayed vigourous plant growth, high fruit yield and resistance/tolerance to many biotic and abiotic stresses. These plants were selected from the germplasm stock and maintained at the Indian Institute of Vegetable Research (IIVR), Varanasi, India.
Genetic diversity analysis
Genomic DNA was isolated from young leaves of 10 parental lines using the modified DNA isolation cetyltrimethyl ammonium bromide (CTAB) method as suggested by Doyle and Doyle (1990). DNA samples were purified and quantified for the PCR amplification of SSR primers. A set of 50 SSR markers of tomato were selected from different chromosomal loci of Sol Genomic Network (http://www.sgn.cornell.edu) database for diversity analysis among 10 diverse parental lines. Cluster analysis was carried out on standardized data based on the Euclidian distance coefficient and unweighted pair group method with arithmetic means (UPGMA) using NTSYS-pc ver. 2.11a, an advanced version of 2.02 (Rohlf 1994).
Hybridization and evaluation
A crossing programme was conducted among four cultivars × six wild accessions in ‘line × tester’ mating design. Twenty-four interspecific hybrids were obtained through manual pollination during the months of October and November, 2006. The 34 genotypes (24 F1’ s + 10 parents) of tomato were considered for the experiment and evaluated in two (field and glasshouse) environments during the months of October–February of 2007–2008. Twenty-one day-old seedlings were transplanted in a randomized complete block design (RCBD) with three replications in a well-prepared field. Each replication included 30 plants at a determined spacing of 45 cm (plant to plant) and 60 cm (row to row). The crop was raised with standard agrotechniques, without the application of any insecticide and fungicide. The recommended dosage and method of application of manures and fertilizers were used. Plants were examined for symptoms which appeared at 30, 60 and 90 days after transplanting (DATP). On the other hand, in glasshouse conditions (mass and cage inoculation) symptoms appeared at 15, 30, 45 and 60 DATP, as reported by Banerjee and Kalloo (1987) and Singh et al. (2010). In mass and cage inoculation condition, 24 F1’s and 10 parental tomato seedlings of each genotype were inoculated at two-week intervals using high pressure of whiteflies. These whiteflies used were collected from previous cultures already maintained in the glasshouse of IIVR, Varanasi. Plants were examined at weekly intervals for ToLCV symptom, expression and disease incidence was observed every 15th day interval which continued for up to 60 days after planting.
Measurement and statistical analysis
For data observation, five plants were randomly selected from each replication, avoiding the border row and were tagged before flowering to collect the data. The observations were recorded for only those traits which were affected by ToLCV disease e.g., coefficient of infection (CI%), plant height (PH) in cm, number of fruits per plant (NFPP), average fruit weight (AFW) in g and fruit yield per plant (FYPP) in kg.
The symptoms of disease was scored on 0–5 scale with some modifications (Banerjee and Kalloo 1987). The coefficient of infection was calculated by the following formulae: (PDI = (total number of diseased plants/total number of observed plants) × 100)
RV is categorized by scoring on 0 = 0, 1 = 0.12, 2 = 0.25, 3 = 0.50, 4 = 0.75 and 5 = 1.00. Where PDI, per cent disease incidence; CI, coefficient of infection; RV, response value. The mean data was subjected to combined ability analysis of variance. Combining ability analysis was carried out according to Singh and Chaudhary (1979) fixed effect model using the following formula:
where μ, general mean; G i, GCA effect of ith line (female parent); G j, GCA effect of jth tester (male parent); S ij, SCA effects of hybrids with the ith lines and jth tester; E ijk, error associated with the ith observation at the plot; i 1, 2 … (1) (number of lines), j = 1, 2…t (number of testers), k = 1, 2, 3…r (number of replications).
Results and discussion
A cluster analysis was carried out among 10 tomato accessions using SSR markers from different chromosomal loci (figure 1). Out of 50 SSR markers, 16 (32%) failed to amplify the expected PCR fragments, 23 (46%) amplified monomorphic banding patterns, while the remaining 11 (22%) generated polymorphic banding patterns (table 1). The effects of morphological plasticity cluster were different in SSR analysis (Frary et al. 2005), which is the capacity of the organisms with different genotypes to vary in varying phenotypic and genotypic conditions and parallel evolution. A total of 116 alleles were detected by these polymorphic markers. The polymorphic information content (PIC) of the markers range from 0.33 to 0.81 with an average 0.47. The SSR304 marker gave the highest PIC of 0.81, the SSR350 had the lowest PIC of 0.33. The low correlation identified is due to a large portion of the variation detected by molecular markers being nonadaptive. Therefore, it is not subjected to either natural or artificial selection as compared with the phenotypic characters, which are also directly influenced by the environment (Vieira et al. 2007). The coefficient range was 0.07–1.09. The dendogram was generated in two clusters of major groups, i.e., cluster ‘A’ and cluster ‘B’. Cluster ‘A’ consisted of cultivars (Punjab Chhuhara, H-24, H-86 and DVRT-2) having marketable yield quality, determinate plant height and susceptibility to ToLCV disease. Even cluster ‘B’ consisted of six wild species (EC-521080, EC-520061, EC-520049, EC-528372, WIR-3957 and WIR-5032) showing indeterminate plant growth and resistance to ToLCV disease. The Mantel test for association among the matrices derived from SSR and morphological data indicated a poor matrix correlation, showing that these methods discriminated very differently among the S. lycopersicum and wilds species (Chen et al. 2011).
Analysis of variances for combining ability (GCA and SCA) was found to be highly significant for all the characters in both field and glasshouse environments (table 2). Significant differences were observed in parents, parents vs crosses and ‘line × tester’ interactions among all the characters, indicating that both additive and nonadditive gene effects played significant roles for the expression of these characters (Saleem et al. 2009). Lines exhibited significant variation for CI, AFW, FYPP whereas, testers were significant for CI, PH, NFPP, AFW and FYPP in both field and glasshouse conditions. Within the GCA effects female parents exhibited nonsignificant variation for each trait, while the male parents exhibited high significant values for each trait except FYPP. This may be due to female parents belonging to the cultivars of S. lycopersicum which do not tolerate disease pressure and are unable to express their vegetative and fruit characters in glasshouse condition (Singh et al. 2010). The SCA variances were also significant in both the environments for CI, PH, NFPP and AFW at P ≤ 0.05, P ≤ 0.01 and P ≤ 0.001%, respectively. Similar results were shown by Hannan et al. (2007) in their experiments.
The value of σ 2GCA female was less than that of σ 2GCA male for all the characters (table 2), thus indicating that the male parent was dominant on female for all characters. The σ 2GCA female was less than σ 2SCA though σ 2SCA was less than σ 2GCA male for all characters except NFPP, indicating predominance of both types of additive and nonadditive gene effects for the characters. The σ 2GCA male showed significance in both field and glasshouse environments for all characters, except FYPP, which was nonsignificant in glasshouse condition but significant in field condition at P ≤ 0.05 level (table 2). However in case of σ 2SCA, all characters were significant in field condition, except NFPP and AFW, while in glasshouse only PH was nonsignificant and all the remaining characters were significant at <0.001. As per results, the ratio of σ 2GCA male/σ 2SCA was <1 (NFPP) and equal to 1 (FYPP), and the degree of dominance (σ 2D/σ 2A)1/2 was either equal to 1 or >1 (CI, PH and AFW), showing predominance for nonadditive gene effect, as recorded by Saleem et al. (2009). Contrary to this, the ratio of σ 2GCA male /σ 2SCA was >1 (CI, PH and AFW) and degree of dominance (σ 2 D/σ 2A)1/2 was <1 (NFPP and FYPP), suggesting predominance of additive type gene action, as followed by Hannan et al. (2007).
GCA and SCA variances with each parent play a significant role in the choice of parents. The GCA component is primarily a function of the additive genetic variance. A parent with higher positive significant GCA effects is considered as a good general combiner. In the present study, all the female and male parents displayed highly positive significant values for CI, except H-86, H-24 and EC-520061 (table 3). These parents were found to exhibit negative significance in both field and glasshouse conditions, proving to be a good combiner for disease resistance as negatively significant CI value showed high disease resistance capacity of parents (Singh et al. 2011). For the plant height (PH), the parents H-86, H-24 and EC-520061 expressed high and positive significant values in both environments, while the remaining parents displayed either negative significance or nonsignificance, and this is also concordant with Hannan et al. (2007). Similarly, for the number of fruit per plant (NFPP), only two parents EC-521080 and EC-520061 showed highly positive and significant values in both the environments while the remaining parents exhibited less or nonsignificant values. These findings were supported by the study of Hannan et al. (2007). The average fruit weight (AFW) displayed a negative or nonsignificant GCA value for all parents, except H-86 (significant in glasshouse) and EC-528372 (significant in field and glasshouse). This is in accordance with the good combiner for fruit weight studied by Saleem et al. (2009) and Hannan et al. (2007). For the fruit yield per plant (FYPP), none of the parents were found significant in both the environments, except WIR-5032. They expressed their significant value for GCA either in field or in glasshouse condition. The parents, DVRT-2 and EC-528372, were significant in field condition, while H-86 was significant in glasshouse condition (table 3) and thus, these parents may be good combiners for yield capacity. These results are in accordance with the studies of Saleem et al. (2009) and Hannan et al. (2007). Specific combining ability (SCA) is based on nonadditive gene effect. Normally, SCA effects do not contribute much to the improvement of self-pollinated crops. As per the results, most of the crosses showed SCA values were either nonsignificant or negative significant for various characters. In the present study, low and negative significant CI values were found in the crosses, i.e., PBC × EC-520049, PBC × EC-520061, H-24 × EC-521080, DVRT-2 × EC-528372 and H-86 × WIR-5032 during both field and glasshouse trials (table 4). These crosses may be good combiners for progeny selection against ToLCV disease resistance, similar to the results of Singh et al. (2011). On the other hand, with regard to the plant height (PH), no cross combination could show a significant value in both field and glasshouse environments. These crosses showed significant values either in field or in glasshouse like, PBC × EC-520061 (significant in field) followed by DVRT-2 × EC-520061 (significant in field) and H-24 × EC-520061 (significant in glasshouse). Similar results of best cross combiners for PH was reported by Ahmad et al. (2009) and Hannan et al.(2007). For FNPP, chronologically high and positive significant values were found in the crosses of PBC × EC-521080, H-24 × WIR-3957, H-86 × EC-520049, PBC × EC-520061 and DVRT-2 × WIR-5032 in both field and glasshouse environments. The fruit yield was high in most crosses, this may be due to the use of wild male parents since they bear more and unexpected number of fruits as reported by Ahmad et al. (2009). Among the 24 F1’s, AFW did not show any positive significant values in any cross combinations in both the environments, except PBC × WIR-3957 (significant in glasshouse). This result may be a guide to any breeder who avoids developing the AFW characters by using interspecific crosses. Poor average fruit weight was reported by many workers (Ahmad et al. 2009; Saleem et al. 2009). Besides disease resistance, fruit yield was also an interesting character for developing a good hybrid by tomato breeders. In the present study, the SCA effect was negative and nonsignificant in most of the crosses for yield traits. No cross combinations exhibited significant results on yield for SCA effects in glasshouse. Only one cross combination (DVRT-2 ×
WIR-5032) was found significant in both the environments, while the remaining crosses showed significant SCA in field condition only, e.g. PBC × EC-521080, DVRT-2 × EC-528372 and H-86 × EC-520049. The best cross combiners for fruit yield were reported in many studies (Hannan et al. 2007; Ahmad et al. 2009; Saleem et al. 2009).
Based on results, it was concluded that the following parents were good general combiners for various characters namely, H-86 (CI, PH, AFW, FYPP), H-24 (CI, PH), DVRT-2 (FYPP), EC-521080 (FNPP), EC-520061 (PH, CI, FNPP), EC-528372 (AFW, FYPP) and WIR-5032 (FYPP). In case of SCA most of the characters were found to be better in various crosses namely CI (PBC × EC-520049, H-24 × EC-521080, DVRT-2 × EC-528372 and PBC × EC-520061), PH (PBC × EC-520061, DVRT-2 × EC-520061 and H-24 × EC-520061), AFW (PBC × WIR-3957), NFPP (PBC × EC-521080, H-24 × WIR-3957 and H-86 × EC-520049) and FYPP (PBC × EC-521080, DVRT-2 × WIR-5032, DVRT-2 × EC-528372 and H-86 × EC-520049). These parents and hybrids could be utilized as good combiners and isolated to obtain desirable segregates for improving respective characters. This study was suggested for a breeding programme for which, the advantages of both types of variances, namely additive and nonadditive are chosen. Due to the presence of additive genetic variance, disease resistant capacity can be improved which may prove highly useful in yield improvement.
References
Ahmad S., Quamruzzaman A. K. M. and Nazimuddin M. 2009 Combining ability estimates of tomato (Solanum lycopersicum) in late summer. SAARC J. Agri. 7, 43–56.
Banerjee M. K. and Kalloo G. 1987 Sources and inheritance of resistant to leaf curl virus in Lycopersicon. Theor. Appl. Genet. 73, 707–710.
Chen H., Fu R. and Li X. 2011 Tomato yellow leaf virus (TYLCV): the structure, ecotypes and the resistance germplasm resources in tomato. Afr. J. Biotech. 10, 13361–13367.
Chomdej O., Whankaew S., Chatchawankanpanich O., Kositratana W. and Chunwongse J. 2008 Resistance to tomato yellow leaf curl Thailand virus, TYLCTHV-[2] from Solanum habrochaites accession (L06112) in F1 and BC1 F 1 generations. Songklanakarin J. Sci. Technol. 30, 441–446.
Doyle J. J. and Doyle J. L. 1990 A rapid DNA isolation procedure from small quantity of fresh leaf material. Phytochem. Bull. 119, 11–15.
Frary A., Xu Y., Liu J., Mitchell S., Tedeschi E. and Tanksley S. D. 2005 Development of a set of PCR-based anchor markers encompassing the tomato genome and evaluation of their usefulness for genetics and breeding experiments. Theor. Appl. Genet. 111, 291–312.
Hannan M. M., Biswas M. K., Ahemed M. B., Hossain M. and Islam R. 2007 Combining ability analysis of yield and yield components in tomato (Lycopersicum esculentum Mill.). Turk. J. Bot. 31, 559–563.
Kalloo G. and Banerjee M. K. 1989 Transfer of tomato leaf curl virus resistance from Lycopersicon hirsutum f. glabraum to L. esculetum. Plant Breed. 105, 156–159.
Lioi L. and Galasso I. 2013 Development of genomic simple sequence repeat markers from an enriched genomic library of grass pea (Lathyrus sativus L.). Plant Breed. 132, 649–653.
Rohlf F. J. 1994 NTSYS-PC: numerical taxonomy and multivariate analysis system version 2.2. State University of New York, Stony Brook, NY, USA.
Saleem M. Y., Asghar M., Haq A., Rafique T., Kamran A. and Khan A. A. 2009 Genetic analysis to identify suitable parents for hybrid seed production in tomato (Lycopersicon esculentum Mill.). Pak. J. Bot. 41, 1107–1116.
Singh R. K. and Chaudhary B. D. (ed.) 1979 Biometrical methods in quantitative genetics analysis. Kalyani Publishers, New Delhi, India.
Singh R. K., Rai N. and Singh S. N. 2010 Response of tomato genotypes to tomato leaf curl virus. Ind. J. Agri. Sci. 80, 755–758.
Singh R. K., Rai N., Singh M., Singh A. K., Kumar P., Singh R. and Singh S. N. 2011 Molecular diversity in tomato genotypes and their exploitation of heterosis against tomato leaf curl virus (ToLCV) and yield traits. Abstract in National Symposium on Vegetable Biodiversity, pp. 47–48. Department of Horticulture, Jawaharlal Nehru Krishi Vishwa Vidyalaya, Jabalpur, India.
Sprague, G. F. (ed.) 1966 Plant breeding. Iowa State University Press, Ames, USA.
Vieira E., Carvalho F., Bertan I., Kopp M., Zimmer P., Benin G. et al. 2007 Association between genetic distances in wheat (Triticum aestivum L.) as estimated by AFLP and morphological markers. Gen. Mol. Biol. 30, 392–399.
Wu F., Eannetta N. T., Xu Y., Durrett R., Mazourek M., Jahn M. M. and Tanksley S. D. 2009 A COSII genetic map of the pepper genome provides a detailed picture of synteny with tomato and new insights into recent chromosome evolution in the genus Capsicum. Theor. Appl. Genet. 118, 1279–1293.
Author information
Authors and Affiliations
Corresponding author
Additional information
[Singh R. K., Rai N., Singh M., Singh S. N. and Srivastava K. 2014 Genetic analysis to identify good combiners for ToLCV resistance and yield components in tomato using interspecific hybridization. J. Genet. 93, xx–xx]
Rights and permissions
About this article
Cite this article
SINGH, R.K., RAI, N., SINGH, M. et al. Genetic analysis to identify good combiners for ToLCV resistance and yield components in tomato using interspecific hybridization. J Genet 93, 623–629 (2014). https://doi.org/10.1007/s12041-014-0433-5
Received:
Revised:
Accepted:
Published:
Issue Date:
DOI: https://doi.org/10.1007/s12041-014-0433-5