Abstract
Inflammatory bowel diseases (IBDs) are the result of pathological immune responses due to environmental factors or microbial antigens into a genetically predisposed individual. Mainly due to their trophic properties, a mounting interest is focused on the use of human mesenchymal stem/stromal cells (hMSCs) to treat IBD disease in animal models. The aim of the study is to test whether the secreted molecules, derived from a specific population of second trimester amniotic fluid mesenchymal stem/stromal cells, the spindle-shaped MSCs (SS-AF-MSCs), could be utilized as a novel therapeutic, cell free approach for IBD therapy. Induction of colitis was achieved by oral administration of dextran sulphate sodium (DSS) (3 % w/v in tap water), for 5 days, to 8-week-old NOD/SCID mice. The progression of colitis was assessed on a daily basis through recording the body weight, stool consistency and bleeding. Conditioned media (CM) derived from SS-AF-MSCs were collected, concentrated and then delivered intraperitoneally into DSS treated mice. To evaluate and determine the inflammatory cytokine levels, histopathological approach was applied. Administration of CM derived from SS-AF-MSCs cells reduced the severity of colitis in mice. More importantly, TGFb1 protein levels were increased in the mice received CM, while TNFa and MMP2 protein levels were decreased, respectively. Accordingly, IL-10 was significantly increased in mice received CM, whereas TNFa and IL-1b were decreased at mRNA level. Our results demonstrated that CM derived from SS-AF-MSCs cells is able to ameliorate DSS-induced colitis in immunodeficient colitis mouse model, and thus, it has a potential for use in IBD therapy.
Similar content being viewed by others
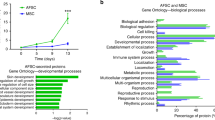
Avoid common mistakes on your manuscript.
Introduction
The two main entities of inflammatory bowel disease (IBD) are Crohn’s disease (CD) and Ulcerative Colitis (UC) and both are characterised as being chronic, relapsing, inflammatory conditions of the gastrointestinal tract. Although the underlying aetiology is not completely understood, it is widely accepted that in genetically predisposed patients a combination of local immune reactions and environmental factors that have an effect on the initialisation and progression of the disease are taking place [1]. Treating IBD has a basic goal to achieve long-term remission (limited symptoms and mucosal lesions healing) [2], reduce long-term disability and maintain good quality of life [3].
The current treatment for IBD begins with 5-ASA agents and antibiotics, followed by corticosteroids, immunomodulators and biologic agents. When all fails, the patients are leaded to surgery [4]. Today, concerning the anti-TNFa biologic agents, the rates of remission still remain low [5]. Certain patients do not respond to anti-TNFa biologic agents, and some patients even if initially achieve an initial response to induction therapy, they lose then this response over time with maintenance treatment [6]. Furthermore, treatments for inflammatory bowel disease are generally noted to have a number of side effects related to the GI tract and liver [6]. Despite the advancements in medical therapy, gastroenterologists still find it difficult to optimize treatment and maintain remission in a large group of patients, and for a significant number of patients, surgery is often the last option [5].
Thus, it is necessary to search for new treatment options for IBD, such as using T lymphocytes, tolerogenic dendritic cells, stem cells [hematopoietic (HSCs), and mesenchymal stem cells (MSCs) in cell therapy [7–9]. More specifically, cell-based treatments for IBD are focused on the use of MSCs derived from bone-marrow [10] or adipose tissue [11, 12]. MSCs are known to supress the immune system by a wide range of mechanisms which inhibit immune cell activation, proliferation and function, and by protecting damaged tissues through the release of anti-inflammatory and anti-apoptotic agents [13]. Furthermore, MSCs can mediate tissue repair by their multilineage differentiation potential and/or via producing growth factors, cytokines, and antioxidants, giving the foundation for their ability to control an immune response [11]. Regarding CD, MSCs therapy of intralesional infusions presented local healing of fistula, whereas the effectiveness of MSCs therapy in refractory IBD patients given systemic infusions seems uncertain and patients who presented clinical response post-treatment were few in number [14]. Basic limitations of this therapy is that transplantation requires a great number of cells, the ensuring that MSCs reach damaged tissue, the donor variance and senescence before culture expansion, as well as immunogenicity induced during culture and cryopreservation [15–17].
Recently, fetal MSCs represent a novel tool for cell-based therapies [18, 19]. Roubelakis et al. [20, 21] isolated MSCs from the human second trimester amniotic fluid (AF-MSCs), attained after informed consent during routine amniocentesis for prenatal diagnosis, from the excess volume of sample that is usually discarded. AF-MSCs exhibit an inherently low immunogenic profile, which decreases the potential for cell rejection and graft-versus-host-disease, making them suitable for allogenic transplantation. In our previous studies, we have shown that at least two morphologically distinct MSC subsets can be isolated from human amniotic fluid [20]. In particular, spindle shaped (SS)-AF-MSCs exhibit higher proliferation rate and enhanced differentiation potential, compared to round shaped (RS)-AF-MSCs and BMSCs [20, 22]. Secretome studies on SS-AF-MSCs, based on proteomics and proteome array analyses, suggested the presence of a number of anti-inflammatory molecules such as interleukins IL-10, IL-1ra, IL-13 and IL-27 [22, 23] and angiogenic factors such as angiopoietin-1, PD-ECGF, uPA and endostatin/collagen XVIII [22, 24].
Roubelakis et al. showed that SS-AF-MSCs and their secreted molecules enhanced the production of vascular tubules in vivo and in vitro, being more effective than BMSCs [24]. Similarly, in 2012, the same group [23] showed that factors secreted by SS-AF-MSCs, such as IL-10, have a substantial advantage in treating acute hepatic failure in a mouse model, suggesting a paracrine anti-inflammatory effect of SS-AF-MSCs. Additionally, Watanabe et al. [25] examined a paracrine hypothesis for the function of rat MSCs against experimental colitis, and suggested that MSCs produced pleiotropic gut trophic factors with a therapeutic potential for treating IBD.
In this study, the efficiency of conditioned media (CM), derived from SS-AF-MSCs, were evaluated in a mouse model where dextran sulphate sodium (DSS) was utilized to induce experimental colitis as a new and innovative approach for IBD cell-based therapy.
Material and Methods
Culture of SS-AF-MSCs and Production of CM
SS-AF-MSCs were obtained and characterized, as previously described [23]. For CM preparation, 1.5 x 106 SS-AF-MSCs were cultured until 80 % confluency and further allowed to grow in DMEM (Sigma-Aldrich) media, containing 0.5 % FBS (Gibco-BRL) for 48 h at 37 °C in a 5 % (vol/vol) humidified CO2 chamber. At 48 h of culture, percentage of dead SS-AF-MSCs was estimated by trypan blue staining (Gibco-BRL) at 9.96 ± 1.29 %. Conditioned media were collected and the total number of living (0 %) or dead cells (0 %, trypan blue positive cells) was estimated. CM was then centrifuged at 1200 rpm for 10 min and the supernatants were then filtered. CM was concentrated (10–25 fold), of the original volume, using a suitable ultra-filtration device (Millipore, Bedford, Massachusetts, USA), as described previously [23]. The molecular cut off of the filters utilized was 3 kDa. The total protein amount contained in the injected dose of CM (200 μl) was 211.8 μg, as determined by Bradford assay (BioRad, Laboratories, Ltd., UK).
Induction of DSS Induced Colitis in NOD-SCID Mice
Forty NOD-SCID mice were housed and maintained at the Animal Facility of the Biomedical Research Foundation of the Academy of Athens. The institute guidelines for the maintenance and treatment of animals, which adhere to the guidelines of the Association for Assessment and Accreditation of Laboratory Animal Care, the recommendations of the Federation of European Laboratory Animal Science Associations and of the National Institute of Health, were strictly followed.
Induction of colitis was performed by oral administration of dextran sulphate sodium (DSS) (MP Biomedicals, Solon, OH) as previously described [26]. Briefly, NOD/SCID mice received DSS in sterilized tap water (3 % DSS w/v) for 5 days (Day1- Day 5). Mice were randomly assigned to 4 experimental groups: 3 % DSS treatment group (control group A, n = 19), 3 % DSS treated with 0.5 % FBS fresh medium (control group B n = 4), 3 % DSS treated with 20 % FBS fresh medium, (control group C n = 4), and 3 % DSS treated with SS-AF-MSC-CM treated group (n = 21). CM was administered by single intraperitoneal injection (200 μl dose). At day 5, after administration of CM, the solution of DSS was replaced by normal water until evaluation. On the 7th day of the experiment mice were sacrificed. Colon tissue, of approximately 2 cm, was excised.
Colitis Activity Evaluation
The progression of the colitis was monitored in daily basis by examination of the animals’ clinical symptoms (weight loss, diarrhoea occurrence and blood in stools) for 5 days following the DSS administration. Weight measurements were performed at Day 1 (before DSS treatment), at Day 2 (one day after DSS administration) and at Day 5, when mice were injected with CM.
Histopathological Analysis
Fresh colon tissue was excised, rinsed in saline solution, fixed in 4 % formalin, and embedded in paraffin wax. Fresh colon tissue was also collected and stored in −80 °C. Sections of tissue (5 μm thick) were retrieved and placed on coated slides before a haemotoylin-eosin staining was carried out. A blind evaluation of histopathologial scores was carried out on each mice separately, as stated in Zhang et al. [27] Histological damage score denoted the combination of damage in the epithelium and infiltration score, ranging from 0 (healthy) to 4 (severe colitis). Briefly, the criteria used to evaluate the severity of colitis were: Score 0: Unaffected, no evidence of inflammation; Score 1: very low level of lymphocyte infiltration of less than 10 % in a high-power field (hpf); Score 2: low lymphocyte infiltration of 10–25 % in hpf; Score 3: moderate level of lymphocyte infiltration (25–50 % in hpf) with high vascular density and thickening of the bowel wall; Score 4: high level of lymphocyte infiltration (> 50 % in hpf), high vascular density, crypt elongation with distortion, and transmural bowel wall thickening with ulceration.
Immunohistochemistry
Formalin-fixed paraffin-embedded (FFPE) sections were stained for TGFb1 (1:100; Acris antibodies, AP06350PU-N), MMP1 (1:150; Novus biologicals, NBP1–72,209), MMP2 (1:200; Acris antibodies, AM00257AF-N) and TNFa (1:30; Acris antibodies) antibodies. Slides were deparaffinised in xylene and rehydrated in a graded alcohol series. Three % H2O2 solution was used to block endogenous peroxidase activity. The immunohistochemical staining on tissue sections was performed using the ZytoChem Plus HRP Kit, Broad Spectrum (Zytomed Systems GmbH, Berlin, Germany), which is based on the streptavidin-biotin detection system, according to the manufacturer’s instructions. An overnight incubation of the tissue, at 4 °C with primary antibodies, was carried out. After being washed 3 times in 1X PBS, the sections were incubated with a biotinylated secondary antibody for 15 min at room temperature. A wash with 1X PBS was carried out before the tissue sections were incubated with horseradish peroxidase (HRP) labelled streptavidin for 15 min at room temperature. Staining was visualized using diaminobenzidine tetrahydrochloride (DAB) and a counterstain with hematoxylin was performed.
The expression of immunohistochemical parameters was classified by two independent blinded observers who used a modified semi-quantitative immunoreactivity score (IRS) according to the method of Remmele and Stegner [28].
Intensity of staining was scored as 1 (negative), 2 (weak), 3 (medium) and 4 (intensive), whereby additional scores of 1 (0 %), 2 (<10 %), 3 (10–50 %), 4 (51–80 %), and 5 (81–100 %) indicated the percentage of positive staining. Multiplication of the respective intensity score (1–4) and the respective additional score (1–5) resulted in the IRS, which ranges between 1 and 20. A Leica DM LB microscope equipped with a digital DFC 320 camera (Leica) and N-Plan objectives were used to examine the tissue slides and to assess intensity of staining. Photos were taken using a Leica Application Suite ver 2.2 preloaded with automatic (software) set conditions for image procurement.
RNA Extraction and Quantitative Real Time PCR
RNA ISO plus (Takara Bio, Otsu, Shiga, Japan) was used on fresh colon tissue to extract total RNA. For reverse transcription, 1 mg of total RNA was incubated for 1 h at 42 °C with 500 mg/ml of Oligo dT 12–18, 10 mM deoxyribonucleotide triphosphates, 5× first-strand buffer, 0.1 M dithiothreitol, and 200 U/ml MMLV reverse transcriptase (Invitrogen, Carlsbad, CA, USA). A DNase treatment was performed on all of the RNA samples before RT-PCR analysis to decrease the risk of the samples being contaminated with DNA.
Assessment of the TNFα, IL1β, IL10 mRNA levels was performed by employing the glyceraldehyde-3-phosphate dehydrogenase (GAPDH) expression levels as a reference gene. Quantitation was performed using SYBR-Green PCR master mix (Applied Biosystem, Foster City, CA, United States) on an ABI Prism 7700 apparatus (Applied Biosystem).
The thermal profile consisted of 95 °C for 2 min, 40 cycles of denaturation at 95 °C for 30 s, annealing at 64 °C for 1 min, and elongation with optics on for fluorescence monitoring at 72 °C for 1 min. For each individual reaction, a final melting curve analysis from 72 to 95 °C was performed to ensure the homogeneity of PCR products. The primers sequences were as follows: GAPDH-(Fw): 5′-TTCACCACCATGGAGAAGGC-3′, GAPDH-(Rv): 5′-GGCATGGACTGTGGTCATGA-3′, TNFα-(Fw): 5′-CACGTCGTAGCAAACCACCAAGTGG-3′, TNFα-(Rv):5’GATAGCAAATCGGCTGACGGTGTGG-3′, IL1β-(Fw): 5′ CAGGTCGCTC AGGGTCACA-3′, IL1β-(Rv): 5’CAGAGGCAAGGAGGAAACACA-3′, IL10-(Fw): 5’CACAAAGCAGCCTTGCAGAA-3′, IL10-(Rv): 5’AGAGCAGGCAGCATAGCAGTG-3′.
Western Blot Analysis
For protein extraction, cells were harvested following trypsinization and cell pellets were re-suspended in isoelectric focusing sample buffer containing 7 M Urea (ApliChem Inc., Missouri, USA), 2 M Thiurea (Fluka, Sigma Aldrich Co., St. Louis USA), 4 % w/v CHAPS (ApliChem Inc., Missouri, USA), 1 % w/v DTE, (Sigma Aldrich Co., St. Louis USA) and 2 % v/v IPG ampholytes (BioRad Laboratories Inc., CA, USA), followed by bath sonication for 10 min. The suspension was centrifuged at 13.000rcf for 10 min and the supernatants were collected. 3.6 % v/v protease inhibitors (Roche) were added to the extracts. Protein concentration was estimated by the use of Bradford reagent (BioRad Laboratories Inc., CA, USA).
CM was collected and centrifuged at 1200 rpm for 10 mins to remove debris and incubated with 7.5 % TCA (Trichloro Acetic Acid), 0.1 % NLS (NLauroyl Sarcosine) at −20 °C overnight. The samples were then centrifuged at 10.000 rcf for 10 min at 4 °C. The supernatant was discarded, and the pellet was washed in ice cold THF (Tetra Hydro Furan) and centrifuged again as described previously. The final pellet was-air dried and resuspended in Isoelectric Focusing (IEF) sample buffer (7 M urea, 2 M thiourea, 4 % CHAPS, 1 % DTE, 2 % IPG buffer and 3.6 % Protease inhibitors) by 10 mins bath sonication.
For western blot analysis, total protein amount of 10 μg derived from cell extracts or CM were separated by 10 % SDS-PAGE, transferred to Hybond-ECL NC membranes (Amersham Biosciences, Sweden) and incubated with the mouse anti-tubulin monoclonal antibody at a dilution 1:2000 (Sigma-Aldrich). The membranes were subsequently incubated with an anti-mouse HRP-conjugated secondary antibody (Santa Cruz Biotechnology Inc.) at a dilution 1:6.000 and developed using the ECL (Perkin-Elmer, MA, USA) detection system. Films were scanned (GS-800, BioRad scanner) and analyzed using Quantity One software (BioRad Laboratories Inc., CA, USA).
Statistics
In all experiments the mean values ± standard deviations (SD) were calculated. Experiments were performed in triplicate. The ANOVA test, and then Bonferroni’s post hoc test, was used for multiple comparison of results to find statistically significant differences. For these analyses, GraphPad Prism software (version 6.0) was used. Results with probability values less than 0.05 (p < 0.05) were classified as statistically significant.
Results
Establishment of DSS-Associated Colitis in NOD/SCID Immunosuppressed Mice
We aimed to investigate the potential therapeutic role of secreted soluble factors derived from SS-AF-MSCs in to the DSS-associated colitis. To achieve this goal, we examined whether the intraperitoneally administration of CM derived from SS-AF-MSCs could improve the pathological phenotype. Intraperitoneally injection was selected as the most optimal administration route, based on previous studies [25]. In our DSS-induced colitis model, mice showed initial, mild, and severe clinical signs of disease, once the 5-day treatment with DSS had been completed. The body weight loss was clearly observed as indicated in Fig. 1 (Day +1: 22.6 kg ± 2.6 vs Day +5: 16.1 kg ± 1.9, p < 0.0001). Additionally, the Disease Activity Index (DAI), including clinical parameters of inflammation (weight loss, diarrhoea and/or rectal bleeding) [29], were recorded. At day +5, 18 out of the 40 animals presented symptoms of diarrhoea and/or rectal bleeding. CM injections were performed at day +5, when the initial clinical signs were detected. No side effects due to the CM administration were detected.
CM Treatment Reduced the Extension and the Severity of the Inflammation Compared to Control DSS-Treated Mice
Histopathological examination of the colon sections in the distal and proximal tracts showed that CM treatment significantly decreased the extension and the severity of the inflammation in comparison to the DSS-treated mice control groups (Fig. 2). It is important to notice that fresh medium administration into DSS treated mice at both concentrations had no effect on inflammation and exhibited similar histology pattern to control A, DSS-treated mice group.
Histologic improvement after CM treatment in DSS colitis. Representative H&E-stained paraffin sections A: without DSS treatment; B: 5 days after DSS treatment; C: DSS group treated with 0.5 % FBS fresh medium, D: DSS group treated with 20 % FBS fresh medium and E: CM-treated group (Images are representative of three separate experiments at 10X magnification)
As stated in the Materials and Methods section, a quantitative assessment of histological damage was carried out. It was shown, through the histological score data that CM administration significantly reduced both inflammatory cell infiltration (macrophages and neutrophils), and epithelial injury compared to that of the control A DSS-treated mice. Moreover, CM-treated mice presented a lower histological score (p < 0.01, in comparison to control group A) (Fig. 3a). Western blot analysis was performed for contamination from intracellular proteins such as tubulin in order to evaluate CM quality. Tubulin protein levels were undermined in CM compared to cell extract (Fig. 3b).
Effect of CM treatment on histologic colonic severity. A. Mice fed with 3 % DSS and receiving CM therapy showed a significant lower histologic severity score (1.9 ± 1.04) compared to control group A (without CM treatment) (3 ± 1). [Values are mean ± SD (results are from three separate experiments). **p < 0.01]. B. CM quality control. (a-b) Western blot analysis for tubulin expression in SS-AF-MSC cell extract. (c-d) Western blot analysis for tubulin expression in CM. Equal loading of (i-iii) concentrated and (iv-vi) unconcentrated CM was confirmed by coomassie blue staining
Downregulation of Tissue Inflammation by CM –SS-AF-MSC Treatment
It has been reported previously, that SS-AF-MSC CM treatment exhibits an anti-inflammatory role in mouse models related to inflammatory diseases [23]. More specifically, recent evidence support, that CM treatment is related to the downregulation of pro-inflammatory and upregulation of anti-inflammatory cytokine levels [23, 25]. Similarly, in order to investigate the inflammatory response at the site of the injury, we examined the mRNA levels of pro-inflammatory (TNF-a and IL-1β) and anti-inflammatory (IL-10) cytokines in colon tissues derived from the mice studied. Notably, we found that CM treatment reduced the inflammatory responses, as indicated in Fig. 4, where the relative expression levels of IL-10 mRNA levels were significantly increased (2.13-fold expression ±0.22) in DSS treated mice received CM, compared with the control group A. Similarly, TNF-a (1.56-fold expression ±0.42) and IL-1b (1.81-fold expression ±0.21) relative expression levels were decreased at mRNA level compared to control group A (Fig. 4).
Relative mRNA expression of inflammatory mediators (IL-10, TNF-a, IL-1b). The colon tissue from CM-treated and from control mice was prepared on day 7, and real-time RT-PCR was performed as described in the Materials and Methods section. The results refer to the comparison of DSS-treated mice received CM vs the control group A mice. Values are mean ± SD
Additionally, immunohistochemical analysis revealed that protein expression of TGFb1, was significantly higher in the CM-treated mice (4.1 ± 1.29) compared to the control group A (2.05 ± 0.69) (p < 0.0001). In agreement with these findings, TNF-a and MMP2, both actively related in systemic inflammation, were found downregulated at protein levels in colon tissues derived from CM-treated mice compared to the control group A (2.65 ± 0.81 vs 5.75 ± 0.85; p < 0.000, and 1.55 ± 0.69 vs 2.65 ± 1.53; p < 0.01, respectively) (Fig. 5).
a Box plots demonstrating the IRS of TGFb1, TNF-a and MMP2 protein expression in colon tissue of CM-treated and control mice. The results are expressed as mean ± SD; **p < 0.01, ***p < 0.0001; b Representative Images of immunohistochemical staining of TGFb1, TNF-a and MMP2 protein in colonic tissue (Images are representative of three separate experiments at 20X magnification)
Discussion
Although the aetiology of IBD is unknown, it is acknowledged that epithelial cells are able to secrete and respond to a variety of immunological mediators, thus suggesting that these cells may play an important role in IBD pathogenesis [30]. It has been shown that MSC treatment is related to decreased expression of a wide range of inflammatory cytokines and chemokines that modulate the systemic immune response. Thus, many studies have been focused on the use of MSC therapy to treat IBD [31–33]. Nevertheless, the exact functional role of MSCs in the recipient tissue remains uclear [34]. It has been suggested that MSCs contribute either via engraftment and differentiation by replacing damaged tissue and/or as tropic suppliers by stimulating tissue repair through the secretion of paracrine factors [35]. Nevertheless, it has been recently shown that MSC therapeutic effects are not related to engraftment and differentiation, but instead, relies on the paracrine and/or endocrine factors the MSCs secrete favouring tissue repair [23, 25, 36].
Our previous studies suggested the secretion of numerous anti-inflammatory molecules such as interleukins IL-10, IL-1ra, IL-13 and IL-27 [22, 23] and angiogenic factors such as angiopoietin-1, PD-ECGF, uPA and endostatin/collagen XVIII by AF-MSCs [22, 24].
In the present study, we focused on the anti-inflammatory effects of the SS-AF-MSCs secretome in DSS colitis mouse model. Based on our previous studies SS-AF-MSCs represent an advantageous cell type due to their inherently low immunogenic profile and high proliferation rate compared to adult MSCs [20, 21, 23]. In this study, we show, for the first time, that a single intraperitoneal injection of CM at the onset of the DSS-colitis improved the clinical and histopathologic severity of colitis in this animal model. Data obtained by real-time RT-PCR analysis support that the CM-treatment significantly downregulated the expression of inflammation markers such as TNF-a, and IL-1b at mRNA level, while upregulated anti-inflammatory cytokines such as IL-10 at mRNA level. It is known that IBD is related to an increase in activation of immune cells in the intestine, whose overproduction of pro-inflammatory cytokines, such as TNF-a, and IL-1b, is involved in mediating the sustained inflammatory response [37].
Additionally, IL-10 acts on Th1 cells and macrophages mainly by an inhibitory mechanism, which in turn inhibits the production of pro-inflammatory cytokines, including IL-1, TNF-a, and IL-6 [38]. In agreement with previous studies, our data support that CM derived from SS-AF-MSCs might act through an anti-inflammatory mechanism, indicating in this way a potential therapeutic approach for IBD treatment [23, 33, 39]. Further functional in vivo and in vitro studies are needed to unveil the exact mechanism by which these molecules exhibit an anti-inflammatory effect on DSS induced colitis.
Immunohistochemical analyses exhibited an advantageous effect of CM-treatment in colon tissues of DSS-treated mice. More specifically, protein levels of TNF-a, and MMP2 were found notably elevated in colonic tissues of mice that did not received CM-treatment, reflecting ongoing inflammation and impaired healing [40], and were significantly decreased in the CM-treated group. Concerning MMP2, transcripts or protein levels of MMP-1, −2, −3, −7, −9, −10, −12, and −13 are demonstrated to be upregulated in inflamed IBD mucosa or serum of IBD patients, and MMP proteolytic activity was increased in cells from inflamed IBD epithelium. In agreement to our findings, MMP2 was also shown to be upregulated in a rat TNBS-induced colitis model and corresponded with the severity of the disease [41]. Beyond the extracellular matrix degradation and remodelling, in intestinal inflammation MMPs can activate or inhibit a wide range of cytokines, chemokines, receptors, adhesion molecules and signalling molecules in order to regulate local inflammation [42]. Thus, SS-AF-MSCs CM-treatment leads to MMP2 protein levels decrease which could be of benefit in treating IBD. We also observe elevated protein expression of TGFb1 in CM-treated mice. It is known that TGFb receptor signalling plays a basic part in controlling the progression and recovery of inflammation-related colitis [43] Recently, Liu et al. [44] maintained that MSCs transplantation substantially improves DSS-colitis through recruited macrophages increasing their TGFβ1 production. Collectively, in agreement with previous studies that used bone marrow MSCs, our results support that CM derived from SS-AF-MSCs, may also improve DSS-colitis by down-regulating of Th1-Th17-driven inflammatory responses (TNF-α, IL-1b), and through the up-regulation of Th2 activities (IL-10) [45, 46].
In conclusion, our findings suggest that paracrine effects and secreted molecules from SS-AF-MSCs may represent an attractive tool for cell-free therapy for IBD. However, integrated identification and validation of the key molecular determinants and mechanism (s) mediated by the CM derived from AF-MSCs are needed in order to prove if this strategy may represent one of the main challenges of stem cell applications in inflammatory bowel diseases.
References
Cho, J. H. (2008). The genetics and immunopathogenesis of inflammatory bowel disease. Nature Reviews Immunology, 8(6), 458–466. doi:10.1038/nri2340.
Rogler, G., Vavricka, S., Schoepfer, A., & Lakatos, P. L. (2013). Mucosal healing and deep remission: what does it mean? World Journal of Gastroenterology, 19(43), 7552–7560. doi:10.3748/wjg.v19.i43.7552.
Hommes, D., & Travis, S. (2012). Leading change in inflammatory bowel disease. Foreword. Journal of Crohn's & Colitis, 6(Suppl 2), S223. doi:10.1016/S1873-9946(12)60501-7.
Thomas, A., & Lodhia, N. (2014). Advanced therapy for inflammatory bowel disease: a guide for the primary care physician. Journal of the American Board of Family Medicine : JABFM, 27(3), 411–420. doi:10.3122/jabfm.2014.03.130224.
Peyrin-Biroulet, L., Loftus Jr., E. V., Colombel, J. F., & Sandborn, W. J. (2011). Long-term complications, extraintestinal manifestations, and mortality in adult Crohn’s disease in population-based cohorts. Inflammatory Bowel Diseases, 17(1), 471–478. doi:10.1002/ibd.21417.
Jneid, H., Anderson, J.L., Wright, R.S., Adams, C.D., Bridges, C.R., Casey, D.E., Jr., Ettinger, S.M., Fesmire, F.M., Ganiats, T.G,, Lincoff, A.M., Peterson, E.D., Philippides, G.J., Theroux, P., Wenger, N.K., Zidar, J.P. (2012) 2012 ACCF/AHA focused update of the guideline for the management of patients with unstable angina/non-ST-elevation myocardial infarction (updating the 2007 guideline and replacing the 2011 focused update): a report of the American College of Cardiology Foundation/American Heart Association task force on practice guidelines. Journal of the American College of Cardiology 60 (7), 645–681. doi:10.1016/j.jacc.2012.06.004
Desreumaux, P., Foussat, A., Allez, M., Beaugerie, L., Hebuterne, X., Bouhnik, Y., Nachury, M., Brun, V., Bastian, H., Belmonte, N., Ticchioni, M., Duchange, A., Morel-Mandrino, P., Neveu, V., Clerget-Chossat, N., Forte, M., Colombel, J.F., (2012) Safety and efficacy of antigen-specific regulatory T-cell therapy for patients with refractory Crohn’s disease. Gastroenterology 143 (5), 1207–1217–e1201–1202. doi:10.1053/j.gastro.2012.07.116
Thomson, A. W., & Robbins, P. D. (2008). Tolerogenic dendritic cells for autoimmune disease and transplantation. Annals of the Rheumatic Diseases, 67(Suppl 3), iii90–iii96. doi:10.1136/ard.2008.099176.
Irhimeh, M. R., & Cooney, J. (2016). Management of inflammatory bowel disease using stem cell therapy. Current Stem Cell Research & Therapy, 11, 72–77.
Park, J. S., Yi, T. G., Park, J. M., Han, Y. M., Kim, J. H., Shin, D. H., Tak, S. J., Lee, K., Lee, Y. S., Jeon, M. S., Hahm, K. B., Song, S. U., & Park, S. H. (2015). Therapeutic effects of mouse bone marrow-derived clonal mesenchymal stem cells in a mouse model of inflammatory bowel disease. Journal of Clinical Biochemistry and Nutrition, 57(3), 192–203. doi:10.3164/jcbn.15-56.
Forte, D., Ciciarello, M., Valerii, M. C., De Fazio, L., Cavazza, E., Giordano, R., Parazzi, V., Lazzari, L., Laureti, S., Rizzello, F., Cavo, M., Curti, A., Lemoli, R. M., Spisni, E., & Catani, L. (2015). Human cord blood-derived platelet lysate enhances the therapeutic activity of adipose-derived mesenchymal stromal cells isolated from Crohn’s disease patients in a mouse model of colitis. Stem Cell Research & Therapy, 6, 170. doi:10.1186/s13287-015-0166-2.
Perez-Merino, E. M., Uson-Casaus, J. M., Zaragoza-Bayle, C., Duque-Carrasco, J., Marinas-Pardo, L., Hermida-Prieto, M., Barrera-Chacon, R., & Gualtieri, M. (2015). Safety and efficacy of allogeneic adipose tissue-derived mesenchymal stem cells for treatment of dogs with inflammatory bowel disease: clinical and laboratory outcomes. Veterinary Journal. doi:10.1016/j.tvjl.2015.08.003.
Uccelli, A., Moretta, L., & Pistoia, V. (2006). Immunoregulatory function of mesenchymal stem cells. European Journal of Immunology, 36(10), 2566–2573. doi:10.1002/eji.200636416.
Duijvestein, M., Vos, A. C., Roelofs, H., Wildenberg, M. E., Wendrich, B. B., Verspaget, H. W., Kooy-Winkelaar, E. M., Koning, F., Zwaginga, J. J., Fidder, H. H., Verhaar, A. P., Fibbe, W. E., van den Brink, G. R., & Hommes, D. W. (2010). Autologous bone marrow-derived mesenchymal stromal cell treatment for refractory luminal Crohn’s disease: results of a phase I study. Gut, 59(12), 1662–1669. doi:10.1136/gut.2010.215152.
Touboul, T., Hannan, N. R., Corbineau, S., Martinez, A., Martinet, C., Branchereau, S., Mainot, S., Strick-Marchand, H., Pedersen, R., Di Santo, J., Weber, A., & Vallier, L. (2010). Generation of functional hepatocytes from human embryonic stem cells under chemically defined conditions that recapitulate liver development. Hepatology, 51(5), 1754–1765. doi:10.1002/hep.23506.
Martinez-Montiel, M. P., Gomez-Gomez, G. J., & Flores, A. I. (2014). Therapy with stem cells in inflammatory bowel disease. World Journal of Gastroenterology, 20(5), 1211–1227. doi:10.3748/wjg.v20.i5.1211.
Kim, N., & Cho, S. G. (2015). New strategies for overcoming limitations of mesenchymal stem cell-based immune modulation. International Journal of stem cells, 8(1), 54–68. doi:10.15283/ijsc.2015.8.1.54.
Trohatou, O., Anagnou, N. P., & Roubelakis, M. G. (2013). Human amniotic fluid stem cells as an attractive tool for clinical applications. Current Stem Cell Research & Therapy, 8(2), 125–132.
Roubelakis, M. G. (2013). Therapeutic potential of fetal mesenchymal stem cells. Current Stem Cell Research & Therapy, 8(2), 115–116.
Roubelakis, M. G., Bitsika, V., Zagoura, D., Trohatou, O., Pappa, K. I., Makridakis, M., Antsaklis, A., Vlahou, A., & Anagnou, N. P. (2011). In vitro and in vivo properties of distinct populations of amniotic fluid mesenchymal progenitor cells. Journal of Cellular and Molecular Medicine, 15(9), 1896–1913. doi:10.1111/j.1582-4934.2010.01180.x.
Roubelakis, M. G., Pappa, K. I., Bitsika, V., Zagoura, D., Vlahou, A., Papadaki, H. A., Antsaklis, A., & Anagnou, N. P. (2007). Molecular and proteomic characterization of human mesenchymal stem cells derived from amniotic fluid: comparison to bone marrow mesenchymal stem cells. Stem Cells and Development, 16(6), 931–952. doi:10.1089/scd.2007.0036.
Zagoura, D. S., Trohatou, O., Bitsika, V., Makridakis, M., Pappa, K. I., Vlahou, A., Roubelakis, M. G., & Anagnou, N. P. (2013). AF-MSCs fate can be regulated by culture conditions. Cell Death & Disease, 4, e571. doi:10.1038/cddis.2013.93.
Zagoura, D. S., Roubelakis, M. G., Bitsika, V., Trohatou, O., Pappa, K. I., Kapelouzou, A., Antsaklis, A., & Anagnou, N. P. (2012). Therapeutic potential of a distinct population of human amniotic fluid mesenchymal stem cells and their secreted molecules in mice with acute hepatic failure. Gut, 61(6), 894–906. doi:10.1136/gutjnl-2011-300908.
Roubelakis, M. G., Tsaknakis, G., Pappa, K. I., Anagnou, N. P., & Watt, S. M. (2013). Spindle shaped human mesenchymal stem/stromal cells from amniotic fluid promote neovascularization. PloS One, 8(1), e54747. doi:10.1371/journal.pone.0054747.
Watanabe, S., Arimura, Y., Nagaishi, K., Isshiki, H., Onodera, K., Nasuno, M., Yamashita, K., Idogawa, M., Naishiro, Y., Murata, M., Adachi, Y., Fujimiya, M., Imai, K., & Shinomura, Y. (2014). Conditioned mesenchymal stem cells produce pleiotropic gut trophic factors. Journal of Gastroenterology, 49(2), 270–282. doi:10.1007/s00535-013-0901-3.
Whittem, C. G., Williams, A. D., & Williams, C. S. (2010). Murine colitis modeling using dextran sulfate sodium (DSS). Journal of visualized experiments : JoVE, 35. doi:10.3791/1652.
Zhang, H., Zhang, X., Ding, X., Cao, W., Qu, L., & Zhou, G. (2014). Effect of secondary lymphoid tissue chemokine suppression on experimental ulcerative colitis in mice. Genetics and molecular research : GMR, 13(2), 3337–3345. doi:10.4238/2014.April.29.12.
Remmele, W., & Stegner, H. E. (1987). Recommendation for uniform definition of an immunoreactive score (IRS) for immunohistochemical estrogen receptor detection (ER-ICA) in breast cancer tissue. Der Pathologe, 8(3), 138–140.
De Fazio, L., Cavazza, E., Spisni, E., Strillacci, A., Centanni, M., Candela, M., Pratico, C., Campieri, M., Ricci, C., & Valerii, M. C. (2014). Longitudinal analysis of inflammation and microbiota dynamics in a model of mild chronic dextran sulfate sodium-induced colitis in mice. World Journal of Gastroenterology, 20(8), 2051–2061. doi:10.3748/wjg.v20.i8.2051.
Pedersen, G. (2015). Development, validation and implementation of an in vitro model for the study of metabolic and immune function in normal and inflamed human colonic epithelium. Danish medical journal, 62(1), B4973.
Aggarwal, S., & Pittenger, M. F. (2005). Human mesenchymal stem cells modulate allogeneic immune cell responses. Blood, 105(4), 1815–1822. doi:10.1182/blood-2004-04-1559.
Prockop, D. J. (2009). Repair of tissues by adult stem/progenitor cells (MSCs): controversies, myths, and changing paradigms. Molecular therapy : the journal of the American Society of Gene Therapy, 17(6), 939–946. doi:10.1038/mt.2009.62.
He, X. W., He, X. S., Lian, L., Wu, X. J., & Lan, P. (2012). Systemic infusion of bone marrow-derived mesenchymal stem cells for treatment of experimental colitis in mice. Digestive Diseases and Sciences, 57(12), 3136–3144. doi:10.1007/s10620-012-2290-5.
Wei, Y., Nie, Y., Lai, J., Wan, Y. J., & Li, Y. (2009). Comparison of the population capacity of hematopoietic and mesenchymal stem cells in experimental colitis rat model. Transplantation, 88(1), 42–48. doi:10.1097/TP.0b013e3181a9f0a7.
Khalil, P. N., Weiler, V., Nelson, P. J., Khalil, M. N., Moosmann, S., Mutschler, W. E., Siebeck, M., & Huss, R. (2007). Nonmyeloablative stem cell therapy enhances microcirculation and tissue regeneration in murine inflammatory bowel disease. Gastroenterology, 132(3), 944–954. doi:10.1053/j.gastro.2006.12.029.
Uccelli, A., Laroni, A., Freedman, M.S., (2011) Mesenchymal stem cells for the treatment of multiple sclerosis and other neurological diseases. The Lancet Neurology 10 (7), 649–656. doi:10.1016/S1474-4422(11)70121-1
Dionne, S., Hiscott, J., D’Agata, I., Duhaime, A., & Seidman, E. G. (1997). Quantitative PCR analysis of TNF-alpha and IL-1 beta mRNA levels in pediatric IBD mucosal biopsies. Digestive Diseases and Sciences, 42(7), 1557–1566.
Kucharzik, T., Stoll, R., Lugering, N., & Domschke, W. (1995). Circulating antiinflammatory cytokine IL-10 in patients with inflammatory bowel disease (IBD). Clinical and Experimental Immunology, 100(3), 452–456.
Chen, Z., He, X., He, X., Chen, X., Lin, X., Zou, Y., Wu, X., & Lan, P. (2014). Bone marrow mesenchymal stem cells ameliorate colitis-associated tumorigenesis in mice. Biochemical and Biophysical Research Communications, 450(4), 1402–1408. doi:10.1016/j.bbrc.2014.07.002.
Telgenhoff, D., & Shroot, B. (2005). Cellular senescence mechanisms in chronic wound healing. Cell Death and Differentiation, 12(7), 695–698. doi:10.1038/sj.cdd.4401632.
Mao, J. W., Tang, H. Y., Tan, X. Y., & Wang, Y. D. (2012). Effect of Etiasa on the expression of matrix metalloproteinase-2 and tumor necrosis factor-alpha in a rat model of ulcerative colitis. Molecular Medicine Reports, 6(5), 996–1000. doi:10.3892/mmr.2012.1021.
O’Sullivan, S., Gilmer, J. F., & Medina, C. (2015). Matrix metalloproteinases in inflammatory bowel disease: an update. Mediators of Inflammation, 2015, 964131. doi:10.1155/2015/964131.
Del Zotto, B., Mumolo, G., Pronio, A. M., Montesani, C., Tersigni, R., & Boirivant, M. (2003). TGF-beta1 production in inflammatory bowel disease: differing production patterns in Crohn’s disease and ulcerative colitis. Clinical and Experimental Immunology, 134(1), 120–126.
Liu, W., Zhang, S., Gu, S., Sang, L., & Dai, C. (2015). Mesenchymal stem cells recruit macrophages to alleviate experimental colitis through TGFbeta1. Cellular Physiology and Biochemistry : International Journal of Experimental Cellular Physiology, Biochemistry, and Pharmacology, 35(3), 858–865. doi:10.1159/000369743.
Delcenserie, V., Martel, D., Lamoureux, M., Amiot, J., Boutin, Y., & Roy, D. (2008). Immunomodulatory effects of probiotics in the intestinal tract. Current Issues in Molecular Biology, 10(1–2), 37–54.
Chen, Q. Q., Yan, L., Wang, C. Z., Wang, W. H., Shi, H., Su, B. B., Zeng, Q. H., Du, H. T., & Wan, J. (2013). Mesenchymal stem cells alleviate TNBS-induced colitis by modulating inflammatory and autoimmune responses. World Journal of Gastroenterology, 19(29), 4702–4717. doi:10.3748/wjg.v19.i29.4702.
Author information
Authors and Affiliations
Corresponding author
Ethics declarations
Conflict of Interest
The author declares that there is no conflict of interest.
Rights and permissions
About this article
Cite this article
Legaki, E., Roubelakis, M.G., Theodoropoulos, G.E. et al. Therapeutic Potential of Secreted Molecules Derived from Human Amniotic Fluid Mesenchymal Stem/Stroma Cells in a Mice Model of Colitis. Stem Cell Rev and Rep 12, 604–612 (2016). https://doi.org/10.1007/s12015-016-9677-1
Published:
Issue Date:
DOI: https://doi.org/10.1007/s12015-016-9677-1