Abstract
The lipase from Pseudomonas fluorescens (Lipase AK, AKL) was immobilized onto the magnetic Fe3O4 nanoparticles via hydrophobic interaction. Enzyme loading and immobilization yield were determined as 21.4 ± 0.5 mg/g and 49.2 ± 1.8 %, respectively. The immobilized AKL was successfully used for resolution of 2-octanol with vinyl acetate used as acyl donor. Effects of organic solvent, water activity, substrate ratio, and temperature were investigated. Under the optimum conditions, the preferred isomer for AKL is the (R)-2-octanol and the highest enantioselectivity (E = 71.5 ± 2.2) was obtained with a higher enzyme activity (0.197 ± 0.01 μmol/mg/min). The results also showed that the immobilized lipase could be easily separated from reaction media by the magnetic steel and remained 89 % of its initial activity as well as the nearly unchanged enantioselectivity after five consecutive cycles, indicating a high stability in practical operation.
Similar content being viewed by others
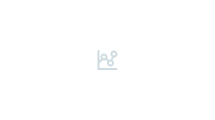
Explore related subjects
Discover the latest articles, news and stories from top researchers in related subjects.Avoid common mistakes on your manuscript.
Introduction
Enzymes have been widely applied in food industries and pharmaceuticals due to their high catalysis activity, strong specificity, wide sources, and little pollution. However, the further industrial applications of enzymes are seriously limited for their low stability and poor reusability [1–4]. Immobilization could reduce conformational change involved in enzyme inactivation and greatly increase the enzyme stability. Therefore, immobilizing enzymes on solid carriers is perhaps one of the most commonly used strategies to improve operational stability of biocatalysts, including better operation control, easier product recovery without catalyst contamination, and flexibility of reactor design [5–8]. Besides, lower inhibition of reaction products, wider selectivity towards non-natural substrates, and better functional properties make immobilization one of the most popular methods of enzyme improvement towards stabilization. Many reports have been published in this research field [7–12].
Although there exits some disadvantages for non-porous nanoparticles used as immobilization supports [13], magnetic nanoparticles have been showing great potential applications for enzyme immobilization and have attracted much attention [14–20] for the following reasons. Firstly, the large surface-to-volume ratios of magnetic nanoparticles lead to a high enzyme loading. For example, Cui Yanjun et al. [21] have used Fe3O4 nanoparticles to immobilize lipase B from C. antarctica (CaLB) and the loading amount was 43.6 mg/g. Secondly, compared with porous carrier materials, the nanoparticles are subjected to minimum diffusion limitation, thus, the stability and activity of enzymes are higher. Zheng Guo et al. [22] have reported that the thermal stability of Candia cylindracea lipase was enhanced by immobilizing the enzyme on hydrophobic nanoparticles. Jiang et al. [23] have immobilized laccase from Pycnoporus sanguineus using magnetic chitosin microspheres as supporter via adsorption-crossing method and the stability of the immobilized enzyme against acid, alkali, and heat was greatly improved. Dyal et al. [24] have also found that both the activity and stability of Candida rugosa lipase immobilized on γ-Fe304 magnetic nanoparticles were higher than those of free enzyme. Thirdly, in view of the magnetic nanoparticles' superparamagnetic properties, the immobilized enzyme could be easily recycled under a magnetic field which can reduce the operation cost [25, 26]. Despite of these advantages, there have been only few reports so far about the applications of enzymes immobilized onto magnetic nanoparticles in resolution of chiral compounds, and the demonstration of their utility remains an increasing concern.
In our present study, magnetic Fe3O4 nanoparticles with 10–20-nm particle diameter were prepared through thermal cracking method and dispersed with oleic acid. The prepared magnetic nanoparticles were used as supporter to immobilize the lipase from Pseudomonas fluorescens via the hydrophobic interaction between the oleic acid tails on the nanoparticle surface and the hydrophobic area of lipase. Besides, the immobilized AKL was used for resolution of (R,S)-2-octanol with vinyl acetate as acyl donor (Scheme 1) and the effects of organic solvent, water activity, substrate ratio, and temperature were also investigated.
Materials and Methods
Materials
Lipase from P. fluorescens (Lipase AK, AKL) was a gift from Amano (Nagoya, Japan). Octadecylene of regent grade was purchased from Alfa (USA). Vinyl acetate, 2-octanol, FeCl3·6H2O, oleic acid, and other organic solvents of analytical grade were purchased from Shanghai Chemical Reagent Company (China) and used without further purification. (R)-2-octanol (99 %), (S)-2-octanol (99 %) and (R)-(+)-1-Phenylethyl isocyanate ((R)-(+)-PEIC, 97 %) was purchased from Fluka (USA).
Preparation of FeIII (Oleate)3
The solution of FeCl3-CH3OH (0.4 mol/L, 60 ml) and oleic acid (25.4 ml, 72 mmol) were mixed. Brown precipitate was formed by slowly adding NaOH-CH3OH (0.4 mol/L, 180 ml) to the above mixture under vigorous stirring. After being washed with methanol twice, the precipitate was dissolved in n-hexane and washed with deionized water for three times. The solution was dried under vacuum and the mud-like FeIII (oleate)3 was obtained.
Preparation of Monodispersion Magnetic Nanoparticles
FeIII(oleate)3 (2.32 mmol) and oleic acid (2.32 mmol) were added into a two-neck flask (containing 20 ml octadecylene) and stirred under argon. After vacuum pumping for 30 min at 120 °C, the reaction mixture was heated up to 200 °C for 30 min. After that, the mixture was continually heated to 314 °C and refluxed for 30 min. The solution of n-hexane and ethanol (1:1, v/v) was added to the above mixture after the mixture was cooled to room temperature.
The obtained mixture was centrifuged at 9,000 rpm for 30 min. After pouring the supernatant, the solution of trichloromethane and methanol (1:10, v/v) was added to the residues. Then the magnetic nanoparticles were obtained through re-centrifugation and being washed with deionized water for three times. The size and morphology of the magnetic nanoparticles were characterized by transmission electron microscopy (FEI, USA).
Immobilization of AKL
AKL powder (0.1 g) was dispersed in phosphate buffer (10 ml, pH 8.0, 0.1 M) at 4 °C under stirring for 2 h, and the insoluble impurity was removed by centrifugation (8,000 rpm, 5 min). The magnetic nanoparticles (1.0 g) were added into the above AKL solution and then the mixture was stirred at 4 °C for 4 h. After filtering the mixture, the obtained immobilized AKL was washed with phosphate buffer (10 ml, pH 8.0, 0.1 M) for three times and then dried overnight under vacuum.
The amount of AKL immobilized onto the magnetic nanoparticles was measured via Lowry method with BSA as a standard for protein concentration [27]. The hydrolytic activity of the immobilized lipase was determined by the modified cupric acetate method of Lee and Rhee [28]. One unit of hydrolytic activity was defined as the amount of enzyme which liberates 1 μmol equivalent of acid in 1 min at 30 °C.
Control of Water Activity
The water activity (a w) of all reaction mixtures was controlled via adding salts/salt hydrate pairs [29]. The reaction mixtures with different water activity (0.06(LiBr), 0.11(LiCl), 0.24(KCH3COO), 0.33(MgCl2), 0.53(Mg(NO3)2), 0.75(NaCl), and 0.97(K2SO4)) were pre-equilibrated at 25 °C overnight.
Resolution of (R,S)-2-Octanol Catalyzed by Immobilized AKL
The reaction was performed in a 50-ml flat-bottom flask containing 2-octanol (0.32 ml, 2 mmol), vinyl acetate (0.55 ml, 6 mmol), n-hexane (10 ml), water activity (a w = 0.53), and immobilized AKL (50 mg). The flat-bottom flask was incubated on a shaker at 50 °C and 200 rpm. The reaction was terminated via separating the immobilized AKL with magnetic steel. The initial activity was used as the enzyme activity in this study and was detected while the conversion was controlled in the range of the 15∼30 %. One unit of the immobilized enzyme activity (micromoles per milligram per minute) was defined as the amount (in micromoles) of ester produced per minute per milligram of the magnetic particle-enzyme. The experiments were performed triplicate and all data were obtained based on the average values.
Reusability of Immobilized AKL
After the reaction of each batch, the immobilized AKL was recovered via magnetic separation and washed with n-hexane for three times. Then the recycled enzyme was repeatedly used in the next reaction. The residue activity of the recycled enzyme was compared with the enzyme activity of the first reaction cycle (100 %).
Determination of Substrate Conversion, Enantiomeric Excess, and E Value
The remaining 2-octanol was directly analyzed by gas chromatograph on a Shimadzu gas chromatograph (GC-14B, Shimadzu, Japan) equipped with a FID detector and a column (30 m × 0.25 mm × 0.25 μm, EC-1000, Alltech, China). Nitrogen was used as the carrier gas at a flow rate of 60 ml/min. The temperatures of the injector and the detector were 200 and 290 °C, respectively. Temperature programming ranging from 110 to 210 °C with the increment of 15 °C/min was used to determine the concentration of 2-octanol. The conversion (C) of 2-octanol was determined via the decrease of 2-octanol.
In order to determine the enantiomeric excess (ee) and the enantiomeric ratio (E value), 2-octanol was derived by (R)-(+)-PEIC. The distinction of R from S enantiomers was achieved via temperature programming between 110 and 222 °C with the increment of 10 °C/min. The retention time of the (S)-2-octanol and (R)-2-octanol was 16.34 and 16.81 min, respectively. The enantiomeric excess of the 2-octanol (ee s ) was determined by calculating the peak areas of the two derivatives via using Eq. (1). And the enantiomeric ratio (E value) was determined from C and ee s via using Eq. (2) [30]:
Here, S and R represent the peak area of the (S,R)-diastereomer and (R,R)-diastereomer obtained from GC, respectively.
Results and Discussion
Characterization of Magnetic Nanoparticles
The size of the magnetic nanoparticles was characterized by TEM using n-hexane as solvent. As shown in Fig. 1, the obtained magnetic nanoparticles were almost spherical with a mean particle size of 10–20 nm. They were small in size and exhibited excellent dispersibility which might improve the immobilization efficiency of AKL. In this study, the lipase from P. fluorescens (Lipase AK, AKL) was immobilized onto the magnetic Fe3O4 nanoparticles via hydrophobic interaction with enzyme loading of 21.4 mg/g (± 0.5 mg/g) and the immobilization yield up to 49.2 % (± 1.8 %). The specific activity of the immobilized lipase (0.57 ± 0.05 U/mg) increased about 3.2-fold when compared with those of the free lipase, which might well be due to the interfacial activation of the lipase on the hydrophobic surface of the nanoparticles.
Optimization of Reaction Conditions for Resolution of 2-Octanol
In order to optimize the reaction conditions, effects of organic solvent, water activity, substrate ratio, and temperature on the resolution of (R,S)-2-octanol were investigated.
Effects of Organic Solvents
It has been well reported in literature that the influence of reaction medium on the enzyme is prominent [31, 32]. In this study, six solvents with different log P were selected to study the effect of reaction medium on the enzyme-catalyzed resolution of 2-octanol (Table 1). Here, log P is the logarithm of the partition coefficient of a given solvent between n-octanol and water, which was widely used to describe the polarity or hydrophobicity of a solvent. The results showed that the enzyme activity of immobilized AKL decreased with the increasing polarity of the organic solvents. When n-heptane and n-hexane with weaker polarity were used as reaction medium, the enzyme activity was higher; the enantioselectivity was observed the highest when n-hexane was used as organic medium. This may ascribe to the following reasons: the polar or hydrophilic solvent might destroy the optimum catalytic conformation of enzyme via stripping off the essential water (which is necessary to preserve the correct conformation of enzyme) from the enzyme because of its higher affinity to water [33]. Besides, the interaction between the solvent and the enzyme molecular may influence the conformation of the enzyme active site and thus change the enzyme activity and the enantioselectivity [34]. As shown in Table 1, when n-hexane was used as the reaction medium, both the enzyme activity and the E value were higher. Therefore, n-hexane was selected as the optimum solvent for the resolution of 2-octanol.
Effect of Water Activity
When a reaction is performed in organic medium, the enzyme activity may be greatly influenced by the micro-water existing in the reaction system [32]. Water activity (a w) was often used to study the effect of micro-water in the organic solvents. As shown in Fig. 2, the enzyme activity exhibited a bell-shaped curve with the water activity increase and the highest enzyme activity was observed when a w = 0.53. Further increase or decrease of a w resulted in the decline of enzyme activity. It is known that the enzyme needs a certain amount of water to maintain its proper conformation [35]. When the water activity was low, the conformation of AKL was excessively rigid, which made the “induced-fit” process of AKL difficult and led to the low enzyme activity. However, high water activity may lead to an increase of the hydrolysis, which might result in the low transesterification activity of enzyme. Furthermore, the enzyme conformation in organic media with high water activity may be more flexible, which may also decrease the transesterification activity of enzyme [35]. When the effect of water activity on the enantioselectivity was considered, the results in this study indicated that the E value was almost unchanged with the variation of a w values.
Effect of Substrate Ratio
In this study, vinyl acetate was used as acyl donor. It is generally believed that application of vinyl esters as the acylation reagent for transesterification could offer an effective solution to overcome equilibrium because the enol co-product could be immediately transformed irreversibly into acetaldehyde [36]. However, the concentration of substrate may have a great effect on the enzymatic reaction, so the effect of mole ratio of vinyl acetate to 2-octanol from 1:1 to 5:1 on the resolution of 2-octanol was investigated in detail (Fig. 3). It could be observed that the highest enzyme activity was obtained while the substrate ratio was 3:1. Further increase of the substrate ratio from 3:1 could not enhance the enzyme activity. This could be explained by the fact that the enzyme–substrate complex needed to dissociate to ensure the active sites of enzyme were free and then accommodated more substrates. It could also be found from Fig. 4 that the increase of substrate ratio almost had no obvious effect on the enantioselectivity of immobilized AKL.
Effect of Temperature
The effect of temperature from 20 to 70 °C on the enzyme activity and enantioselectivity of the immobilized AKL for resolution of (R,S)-2-octanol was examined. The results shown in Fig. 4 indicated that the enzyme activity was greatly improved when the temperature increased from 20 to 50 °C, and then followed with a decrease by further increasing the temperature. The enzyme activity at low temperature (below 50 °C) might not high [37], but the high temperature (above 50 °C) might lead to the denaturation of enzyme and thus slowed the reaction rate. Higher enantioselectivity was observed as the reaction temperature was lower, which was consistent with the results of Phillips [38] who reported that enzymes could show their highest enantioselectivity under low temperatures. Since the highest enzyme activity was observed at 50 °C with a higher E value (71.5 ± 2.2), 50 °C was chosen as the optimum temperature for the resolution of 2-octanol catalyzed by immobilized AKL.
Comparison Between the Aggregated Lipase and the Immobilized Lipase
Comparison on the resolution of (R,S)-2-octanol catalyzed by the immobilized AKL and the aggregated AKL was studied. It was found that the enzyme activity (0.197 ± 0.01 μmol/mg/min) was dramatically increased by immobilizing the lipase onto the magnetic nanoparticles when compared with that (0.027 ± 0.02 μmol/mg/min) of the aggregated lipase. The possible mechanism might be the interfacial activation induced by the interaction between the large hydrophobic area surrounding the active center of lipases and the hydrophobic solid surfaces of the nanoparticles. Thus, lipases recognized these surfaces similarly to those of their natural substrates and suffered interfacial activation during immobilization [39–41]. Besides, compared with the enantioselectivity (E = 68.8 ± 1.9) of the aggregated AKL, the E value (71.5 ± 2.2) of the immobilized AKL hardly changed. The possible reason was that the immobilization on the prepared nanoparticles hardly influenced the distortion of the lipase during the reaction [42].
Separation and Reusability of Immobilized AKL
The separation effect of immobilized AKL via magnetic steel has been investigated (photos not shown). It was found that the separation of immobilized AKL from the reaction mixture by using magnetic steel only required several seconds and the visual effect of the separation was satisfying for the separated solution was visibly clear.
The supports of the immobilized enzyme can be reused after desorbing the inactivated enzyme upon use and the final cost of the reaction can thus be reduced. The reusability of the immobilized enzyme can also reduce the costs. Therefore, the reusability of the immobilized AKL in resolution of 2-octanol was investigated (Fig. 5). The results showed that there was no significant change in both the activity and the enantioselectivity of the immobilized AKL with the increasing reaction cycles. After five cycles, the activity of the immobilized AKL remained 89 % and the E value was almost unchanged. The decrease of enzyme activity probably ascribed to the following two reasons. One might be the enzyme loss during the reaction cycles, the other probably was the enzyme inactivation caused by the produced enol during the transesterification. In order to overcome this disadvantage, immobilization of AKL onto magnetic nanoparticles via covalent attachment was studying at the moment and the results will be published in the near future.
Conclusion
Lipase AK (AKL) was successfully immobilized onto the magnetic Fe3O4 nanoparticles via hydrophobic interaction and exhibited a higher enzyme activity and enantioselectivity in resolution of 2-octanol through transesterification. Under the optimum conditions, the highest enantioselectivity (E = 71.5) was obtained with a higher enzyme activity (0.197 μmol/mg/min). Although the enzyme activity and enantioselectivity were not higher when compared with those in our previous research [43–48], they were high enough to obtain (S)-2-octanol with high optical purity (99 %). Above all, the immobilized AKL was easy to be separated from the reaction mixture by magnetic steel with little activity loss after five repeated uses, indicating a good potential in further industrial application.
Abbreviations
- AKL:
-
Lipase from Pseudomonas fluorescens (Lipase AK AKL) was purchased from Amano Pharmaceutical Co. Ltd. (Japan)
- a w :
-
Water activity
- E value:
-
The enantiomeric ratio
- ee:
-
The enantiomeric excess
References
Schmid, R. D., & Verger, R. (1998). Angewandte Chemie International Edition, 37, 1608–1633.
Reetz, M. T. (2002). Current Opinion in Chemical Biology, 6, 145–150.
Tutar, H., Yilmaz, E., Pehlivan, E., & Yilmaz, M. (2009). International Journal of Biological Macromolecules, 45, 315–320.
Sheldon, R. A. (2007). Advanced Synthesis and Catalysis, 349, 1289–1370.
Yang, P., Teo, W. K., & Ting, Y. P. (2006). Bioresource Technology, 97, 39–46.
Noureddini, H., Gao, X., & Philkana, R. S. (2005). Bioresource Technology, 96, 769–777.
Mateo, C., Palomo, J. M., Fernandez, L. G., Guisan, J. M., & Fernandez, L. R. (2007). Enzyme and Microbial Technology, 40, 1451–1463.
Rodrigues, R. C., Berenguer, M. Á., & Fernandez, L. R. (2011). Advanced Synthesis and Catalysis, 353, 2216–2238.
Brady, D., & Jordaan, J. (2009). Biotechnology Letters, 31, 1639–1650.
Iyer, P. V., & Ananthanarayan, L. (2008). Process Biochemistry, 43, 1019–1032.
Fernandez, L. R. (2009). Enzyme and Microbial Technology, 45, 405–418.
Hernandez, K., & Fernandez, L. R. (2011). Enzyme and Microbial Technology, 48, 107–122.
Garcia, G. C., Berenguer, M. A., Fernandez, L. R., & Rodrigues, R. C. (2011). Advanced Synthesis and Catalysis, 353, 2885–2904.
Gupta, A. K., & Gupta, M. (2005). Biomaterials, 26, 3995–4021.
Li, X. H., & Sun, Z. H. (2003). Journal of Applied Polymer Science, 58, 1991–1997.
Lu, A. H., Salabas, E. L., & Schuth, F. (2007). Angewandte Chemie International Edition, 46, 1222–1244.
Pan, C. L., Hu, B., Li, W., Sun, Y., Ye, H., & Zeng, X. X. (2009). Journal of Molecular Catalysis B: Enzymatic, 61, 208–215.
Bai, S., Guo, Z., Liu, W., & Sun, Y. (2006). Food Chemistry, 96, 1–7.
Kuroiwa, T., Noguchi, Y., Nakajima, M., Sato, S., Mukataka, S., & Ichikawa, S. (2008). Process Biochemistry, 43, 62–69.
Yong, Y., Bai, Y. X., Li, Y. F., Lin, L., Cui, Y. J., & Xia, C. G. (2008). Process Biochemistry, 43, 1179–1185.
Cui, Y. J., Li, Y. F., Yang, Y., Liu, X., Lin, L., Zhou, L. C., & Pan, F. (2010). Journal of Biotechnology, 150, 171–174.
Zhao, G., Bai, S., & Sun, Y. (2003). Enzyme and Microbial Technology, 32, 776–782.
Jiang, D. S., Sheng, Y. L., Huang, J., Xiao, H. Y., & Zhou, J. Y. (2005). Biochemical Engineering Journal, 25, 15–23.
Dyal, A., Loos, K., Noto, M., Chang, S. W., Spagnoli, C., & Shafi, K. V. P. M. (2003). Journal of the American Chemical Society, 125, 1684–1685.
Liu, X. Q., Guana, Y. P., Shen, R., & Liu, H. Z. (2005). Journal of Chromatography B, 822, 91–97.
Andradea, L. H., Rebelo, L. P., Netto, C. G. C. M., & Toma, H. E. (2010). Journal of Molecular Catalysis B: Enzymatic 66, 55–62.
McCabe, R. W., & Taylor, A. (2004). Tetrahedron, 60, 765–770.
Lee, S. Y., & Rhee, J. S. (1993). Enzyme and Microbial Technology, 15, 617–623.
Halling, P. J. (1992). Biotechnology Technology, 6, 271–276.
Chen, C. S., Fujimoto, Y., Girdaukas, G., & Charles, J. S. (1982). Journal of the American Chemical Society, 104, 1294–1299.
Paravidino, M., Sorgedrager, M. J., Romano, V. A. O., & Hanefeld, U. (2010). Chemistry, 16, 7596–7604.
Paul, A. F., & Alexander, M. K. (1991). Journal of the American Chemical Society, 113, 3166–3171.
Gorman, L. A. S., & Dordick, J. S. (1992). Biotechnology and Bioengineering, 39, 392–397.
Giacometti, J., & Giacometti, F. (2006). Chemical and Biochemical Engineering, 20, 269–274.
Halling, P. J. (1994). Enzyme and Microbial Technology, 16, 178–206.
Ganapati, D. Y., & Archana, H. T. (2003). Enzyme and Microbial Technology, 32, 783–789.
Bayramoglu, G., Yilmaz, M., & Arica, M. Y. (2004). Food Chemistry, 84, 591–599.
Phillips, R. S. (1996). Trends in Biotechnology, 14, 13–16.
Fernández-Lafuente, R., Armisén, P., Sabuquillo, P., Fernández-Lorente, G., & Guisán, J. M. (1998). Chemistry and Physics of Lipid, 93, 185–197.
Fernández-Lorente, G., Palomo, J. M., Cabrera, Z., Guisán, J. M., & Fernández-Lafuente, R. (2007). Enzyme and Microbial Technology, 41, 565–569.
Palomo, J. M., Segura, R. L., Fernández-Lorente, G., Dernas, M., Rua, M. C., Guisán, J. M., & Fernández-Lafuente, R. (2004). Biotechnology Process, 20, 630–635.
Palomo, J. M., Fernández-Lorente, G., Mateo, C., Ortiz, C., Fernández-Lafuente, R., & Guisán, J. M. (2002). Enzyme and Microbial Technology, 31, 775–783.
Yu, D. H., Chen, P., Wang, L., Cheng, Y. M., Wang, Z., & Cao, S. G. (2007). Process Biochemistry, 42, 1312–1318.
Yu, D. H., Wang, Z., Zhao, L. F., Cheng, Y. M., & Cao, S. G. (2007). Journal of Molecular Catalysis B: Enzymatic, 48, 64–69.
Yu, D. H., Wang, Z., Chen, P., Jin, L., Cheng, Y. M., Zhou, J. G., & Cao, S. G. (2007). Journal of Molecular Catalysis B: Enzymatic, 48, 51–57.
Yu, D. H., Ma, D. X., Wang, Z., Wang, Y., Pan, Y., & Fang, X. X. (2012). Process Biochemistry, 47, 479–484.
Zhao, L. F., & Zheng, L. Y. (2011). Biocatalysis and Biotransformation, 29, 47–53.
Du, C., Zhao, B., Li, C. Y., Wang, P., Wang, Z., Tang, J., & Wang, L. (2009). Biocatalysis and Biotransformation, 27, 340–347.
Acknowledgments
The authors are grateful for the financial supports from National Natural Science Foundation of China (nos. 31070708, 20772046, and 21172093) and the Natural Science Foundation of Jilin Province (no. 201115038).
Author information
Authors and Affiliations
Corresponding authors
Rights and permissions
About this article
Cite this article
Xun, En., Lv, Xl., Kang, W. et al. Immobilization of Pseudomonas fluorescens Lipase onto Magnetic Nanoparticles for Resolution of 2-Octanol. Appl Biochem Biotechnol 168, 697–707 (2012). https://doi.org/10.1007/s12010-012-9810-9
Received:
Accepted:
Published:
Issue Date:
DOI: https://doi.org/10.1007/s12010-012-9810-9