Abstract
The brown marmorated stink bug (BMSB), Halyomorpha halys Stål, has dispersed throughout the northern hemisphere, causing disturbances in agroecosystems and urban areas. Studies are, therefore, needed on the biology, physiology, and ecology of BMSB. This project characterized and partially correlated (via histology of plant tissues) the DC electropenetrography (EPG) waveforms related to non-probing (NP and Z) and both salivary sheath and cell rupture feeding. BMSB feeding behaviors were analyzed in comparison with the five other EPG waveform characterization papers published to date for heteropteran insects. Waveforms consisted of epidermal scanning (Hh1), pathway of stylets through leaf tissue (Hh2), X waves (Hh3), and sustained vascular ingestion (Hh4). In addition, a cell-rupturing phase in mesophyll (Hh2) and putative ingestion of resulting macerate (Hh5) were characterized. The most striking results were (1) the similarities of waveforms with other heteropterans, (2) performance of both sheath and cell rupture feeding strategies from all three plant cell types (xylem, likely phloem, and parenchyma macerate) on the same plant part, and (3) two different-appearing, dual-meaning X waves according to ingestion from xylem (Hh3a) or likely from phloem (Hh3b). This study provides a crucial framework for future, quantitative research on feeding behavior of this important invasive pest, to aid in the development of novel pest management tools.
Similar content being viewed by others
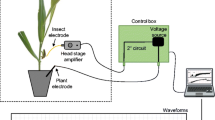
Avoid common mistakes on your manuscript.
Introduction
Halyomorpha halys Stål (Hemiptera: Heteroptera: Pentatomidae), the brown marmorated stink bug (BMSB), is native to Eastern Asia, and was first recorded outside Asia in the mid-1990s in the U.S.A. Since then, BMSB has spread widely throughout North America and is considered an invasive species. BMSB also was accidentally introduced into Europe in mid-2000’s (Wermelinger et al. 2008). BMSB is phytophagous, feeding on various plant organs with a preference for fruits (Rice et al. 2014). It is highly polyphagous in its native region, with most host plants also being present in Europe (Lee et al. 2013; Maistrello et al. 2016, Musolin et al. 2018).
Unlike most other hemipterans, pentatomids can adjust their feeding strategies according to the plant tissue. On seeds or immature plant tissues, they use a cell-rupturing strategy (previously termed lacerate-and-flush; Backus et al. 2005) to ingest from mesophyll/parenchyma or undeveloped tissue. Alternatively, pentatomids can also secrete a salivary sheath on leaves and stems to facilitate intracellular penetration/probing of the stylets, followed by ingestion from vascular cells (Backus 1988; Lucini et al. 2016). A type of electronic monitoring, unrelated to electropenetrography, was used for initial feeding studies of BMSB. That work identified that general probing activities on fruit varied with environmental conditions (Wiman et al. 2014). Electropenetrography, either DC (Tjallingii 1978) or AC-DC (Backus et al. 2019) types, a.k.a Electrical Penetration Graph (both names abbreviated EPG), represents a more rigorous method that is widely used in studies of sternorrynchans (aphids, whiteflies, and psyllids) and auchenorrhynchans (leaf- and planthoppers). EPG allows researchers to observe and analyze precise feeding behaviors, such as pathway, salivation, and phloem or xylem ingestion phases. Only a few EPG studies have been published with heteropterans (Backus et al. 2013; Lucini and Panizzi 2016; Lucini et al. 2016), while none have been published with BMSB yet. However, those preliminary studies have demonstrated strong similarities among waveform appearances for pentatomids and facilitated the development and application of EPG methods to other stink bug models (Lucini and Panizzi 2018) such as the present work with BMSB. Before any comparative, quantitative EPG studies can be performed using this new model, a description of waveforms must be detailed and related to biology of the pest. Therefore, the objective of this study was to identify and define the EPG waveforms of BMSB feeding on a preferred host plant, by electrically characterizing hierarchical waveform phases, families, and types, as well as observationally correlating stylet activities and plant locations with most of the characterized waveforms.
Materials and methods
Plants and insects
Brown marmorated stink bugs (BMSB) were provided by the Institute of Plant Protection, Chinese Academy of Agricultural Sciences, Beijing, where they were fed bean pods. Inside cages in Belgium (16 h light, 23 ± 2 °C), the insects were fed broad bean plants (Vicia faba L. cv. “Grosse Ordinaire”) and sunflower seeds (Helianthus annuus L. cv. unknown).
Prior to EPG recordings, 2-week-old broad beans with four fully developed leaves were individually transplanted into pots filled with loam (La Plaine Chassart, Belgium). Fifth-instar BMSB nymphs were isolated from rearing and starved (with ad libidum access to water) for 24 h prior to recording.
Electropenetrographic recording
Each insect (n = 46) was recorded with a DC electropenetrograph (EPG), following the wiring methods of Lucini and Panizzi (2016). In brief, cuticular lipids were removed by immobilizing the insect on adhesive tape and gently brushing its mesonotum with fine-mesh sandpaper. Then, the mesonotum was glued to a copper nail by a 3-cm gold wire (Alfa Aesar, Kandel, Germany) (diameter: 0.1 mm) with a loop at its extremity. The insect stayed on the tape for at least 40 min, until the silver glue (4 g silver powder [Sigma-Aldrich, Darmstadt, Germany], 4 g paper glue in 4 mL water) used to affix the wire was dry. The nails were attached to the headstage amplifier of a Giga-8d basic DC-EPG system (EPG Systems, Wageningen, The Netherlands), with a fixed input resistance (Ri) of 109 Ohm, and DC applied signal of usually 50–100 mV. The recording was launched directly after the insect was put on the upper side of faba bean leaves. 6-h-long recording was considered sufficient for insects to acclimate to their new conditions and to start stylet probing.
The recorded waveform output was analyzed using the software Stylet+a (EPG Systems) using comparisons with previously published studies that characterized and interpreted waveforms for other Heteroptera, i.e., Backus et al. (2013) and Lucini and Panizzi (2016) for Blissidae and Pentatomidae, respectively. BMSB waveforms were characterized in terms of relative amplitude (in % of voltage drop at the start of the probe) and frequency (in Hz, for peaks and waves), measured on at least 12 events per waveform type from a minimum of four different insects.
Histology of plant leaves
To enhance the reliability of interpreting EPG waveforms, a few additional recordings were performed. The labium and stylets of the insects were carefully cut with dissection scissors when an interesting waveform occurred on the main vein. Precautions were taken to ensure that insects did not move their stylets just before or during the cutting, i.e., maintaining the continuity of waveforms while approaching the bug with scissors, and making a fast and neat cut. The insects were subsequently placed in the freezer to allow them to die. Thin, transverse slices of the leaf, including the stylets, were cut with a razor blade and bleached in sodium hypochlorite 50% (v:v) for a few minutes. The slices were rinsed in distilled water and then immersed in malachite green 0.1% (w:v) for 2 min. The excess stain was removed by rinsing the slices in methanol 70% (v:v). Finally, the slices were stained in Congo red 0.2% (w:v) for 1 min, and then mounted in glycerin water 20% (v:v) on glass slides (VWR, Radnor, PA, USA). All reagents were provided by Sigma-Aldrich, and mounted slices were observed with an Oxion optical microscope (Euromex, Arnhem, The Netherlands) using a × 10 ocular lens and a × 20 objective lens.
Results
General overview of BMSB stylet probing and waveform phases
Several stylet penetrations (a.k.a. stylet probes) with sustained ingestion events were usually recorded during each 6-h plant-access period. In total, 10 different waveforms were described in this study (summarized in Table 1): Np, Z, Hh1, Hh2, Hh3a-1, Hh3a-2, Hh3b, Hh4a, Hh4b, and Hh5. We followed precedent in the literature (see “Discussion” section) to group these EPG waveforms into five phases (Table 1), as follows: (1) non-probing (standing still or moving), (2) epidermal scanning (movements of labium on plant surface), (3) pathway (penetration of the stylets through plant tissues), (4) X wave (transition between pathway and ingestion), and (5) ingestion (xylem or putative phloem sap, or putative macerate). By combining the observed plant histology in the current study with waveform appearances from the published literature, we created reference figures for all main phases (Figs. 1, 2, 3, 4, 5, and 6). Because BMSB’s stylets are thick, the success rate of thin slices of leaves was very low. Therefore, we only validated the position of stylets during xylem ingestion and pathway phases (each n = 3).
Typical Halyomorpha halys (BMSB) electropenetrography waveforms for non-probing (Np) and test probe behaviors. Box A general view of non-probing and three test probes of varying durations. Box B enlarged view of the second test probe. Box C enlarged view of the pathway of the second test probe. Dashed line represents the baseline (0 V)
Typical Halyomorpha halys (BMSB) electropenetrography waveforms for X wave preceding sustained xylem ingestion. Box A general view of pathway, X wave, and sustained xylem ingestion. Box B enlarged view of a more regular pattern during pathway. Box C enlarged view of the transition between pathway and X wave. Box D enlarged view of X wave and the transition between X wave and sustained xylem ingestion
Typical Halyomorpha halys (BMSB) electropenetrography waveforms for X wave preceding putative sustained phloem ingestion. Box A general view of pathway, X wave, and putative phloem ingestion. Box B enlarged view of pathway and the transition between pathway and X wave. Box C enlarged view of the transition between X wave and putative phloem ingestion
Typical Halyomorpha halys (BMSB) electropenetrography waveforms for sustained xylem ingestion. Box A general view of non-probing, pathway, X wave, and sustained xylem ingestion. Box B enlarged view of the transition between pathway and X wave. Boxes C, D enlarged views of sustained xylem ingestion. Dashed line represents the baseline (0 V)
Typical Halyomorpha halys (BMSB) electropenetrography waveforms for putative sustained phloem ingestion. Box A general view of non-probing, pathway, X wave, putative phloem ingestion, and putative macerate ingestion. Boxes B, C, D enlarged views of putative phloem ingestion. Box E enlarged view of the end of putative phloem ingestion, pathway, and the beginning of putative macerate ingestion. Box F enlarged view of putative macerate ingestion. Dashed line represents the baselines (0 V)
Non-probing waveforms and epidermal scanning phases
Waveforms Np and Z
Non-probing phase was divided into two waveform types: Z, when the insect was standing still, and Np, when the insect was walking (Figs. 1 and 2). Z was a quasi-flat waveform on baseline, interrupted by events of irregular waveform with moderate-to-high-amplitude peaks (Np). The latter type occurred each time the insect walked on the leaf surface or when its labium touched the plant epidermis.
Waveform Hh1
We visually correlated epidermal scanning with the waveform Hh1 (Fig. 1). This behavior consisted of labial tip contact with the plant surface and moving of the labium from side to side. Waveform Hh1 always started with a strong voltage drop from baseline, followed by a few irregular peaks. The peaks were rapidly followed by a gradual rise in voltage to very low positive amplitude, until waveform Np returned (Fig. 1).
Pathway phase
As soon as the stylets started penetrating the leaf tissue, the voltage dropped below the baseline (Figs. 2a, b, 5, 6).
Waveform Hh2
The first waveform after the voltage drop at the start of a probe was named Hh2, marking the beginning of pathway phase. Hh2 had irregular-frequency peaks of low amplitude (Fig. 2c). A high number of short probes, consisting only of waveform Hh2, were observed during each recording, interrupting non-probing events. We defined such activity as test probes, during which the stylets penetrated throughout the plant cells without reaching vascular tissues (Fig. 7a). Test probes could be very short (less than 10 s) (Fig. 2), and never progressed to other waveform types. If Hh2 progressed longer than 10 or 20 s, it could become slightly more regular with the addition of frequent peaks (Fig. 3b). Thus, waveform appearances during pathway phase were complex (compare Figs. 2c, 3b). The longest probes usually presented evolving Hh2 waveforms that we did not further subdivide, but could be so divided in future work.
X wave phase
As a stylet probe lengthened, pathway phase (Hh2) gradually evolved into a transition phase (containing waveform family Hh3) that we termed X wave phase (see “Discussion” section). Two different types of X waves, termed Hh3a and Hh3b, were identified. Not only were the appearances of the two types different, but they also were distinguishable by the appearance of repetitive waveforms that followed them.
Waveform Hh3a
The first type of X wave, Hh3a (Fig. 3a, c, and d), started with non-descript humps with few details (Fig. 3c). Over time, a sequence of repeating, high-amplitude, and negative-voltage-level plateaus evolved, containing slight spikes on their otherwise flat tops (Fig. 3d). Each bout (or event) of plateaus (Hh3a-2) was separated by short events of lower-amplitude and negative-voltage-level, high-frequency peaks (Hh3a-1), which apparently evolved from the peaks of Hh2 by becoming larger and more regular (Fig. 3b). Ingestion phase almost always followed Hh3a, when the latter occurred. In a few cases, isolated Hh3a X waves were observed. They were followed by Hh2 waveform that evolved into either more Hh3a, ingestion phase, or probe interruption.
Waveform Hh3b
The second type of X wave, Hh3b, also following Hh2, was marked by a minimum voltage level (or trench) and a very small waveform amplitude (Fig. 4a, b). Thereafter, voltage level irregularly rose until the start of ingestion phase (Fig. 4a, c). Thus, Hh3b was an S-shaped modification of Hh2. Again, ingestion phase almost always followed Hh3b. Once again, isolated X waves (Hh3b) occurred, and were again followed by Hh2 that evolved as before.
Ingestion phase
Putative ingestion waveforms (see “Discussion”) were composed of events of variable-duration repeating, rounded plateaus (an event also being termed a wave; see “Discussion” for details) separated by positive- or negative-going peaks. Three types of ingestion waveforms were identified: Hh4a, Hh4b, and Hh5. As ingestion phase progressed with time, waveforms gradually rose in voltage level until they sometimes crossed the baseline (0 V) and became positive-going. This type of penetration with shifting negative-to-positive voltage levels is termed biphasic.
Waveform Hh4a
About 50% of ingestion events were composed of waveform Hh4a (Fig. 5a, c, d). This waveform usually followed Hh3a (Figs. 3, 5b) and, in fact, Hh4a strongly resembled Hh3a-2, but continued into a much longer event. Stable (i.e., appearance was no longer evolving) Hh4a usually presented negative-going peaks between the waves. Hh4a often was biphasic, starting at a negative voltage level and later rising strongly into a high positive level (Fig. 5a), without major waveform differences, while both voltage level and amplitude progressively rose. Waveform Hh4a was histologically correlated with the position of the stylets in xylem tissue (Fig. 7b). Fifty-four percent of Hh4a ingestion events were preceded by waveform Hh3a.
Waveform Hh4b
Approximately 25% of sustained ingestion events were composed of waveform Hh4b. We were unable to histologically correlate Hh4b with any stylet tip location. Characteristics of waveform Hh4b are portrayed in Fig. 6. Hh4b was similar to Hh4a except that it generally had positive-going peaks between the waves instead of negative-going peaks. Also, the amplitude of Hh4b was usually shorter than Hh4a. The relative amplitude of Hh4b always rose progressively from the amplitude level of Hh3a to reach a rather short height (maximum of 15%) (Fig. 6a–d). Like Hh4a, Hh4b could also be biphasic, without major waveform differences; however, unlike Hh4a, Hh4b rose to a level barely above baseline (Fig. 6a). Hh3b X waves preceded 70% of Hh4b ingestion events.
Waveform Hh5
The remaining 25% of sustained ingestion events were composed of this waveform, termed Hh5. This waveform often followed Hh4a or Hh4b ingestion and a short-to-medium, irregular-appearing pathway phase (but never an X wave). We identified this waveform as a fifth type because it was very different in appearance from the Hh4 waveforms (Fig. 6f). Although spiky plateaus were visible, they did not take on the typical peak-and-wave appearance of the Hh4 ingestion waveforms. Hh5 was a high-frequency waveform consisting of short plateaus of varying amplitude with tiny, high-frequency spikes on the top of each plateau, presenting an almost-irregular appearance (Fig. 6a, e, and f), until the end of the stylet probe.
Probe termination
After ingestion phases ended but before the return to Np, a short pathway phase always occurred during the withdrawal of stylets out of the plant tissue.
Discussion
About our instrument
This electropenetrography study is the first describing waveforms for BMSB, using a Giga-8 DC-EPG device. This instrument’s obligate DC current, coupled with its fixed high Ri, has been documented to disturb large insects such as BMSB during recording, potentially reducing the duration and numbers of all waveforms events but especially ingestion events (Backus et al. 2018). Such instrument-based biases must be taken into account for future quantitative studies. However, DC applied signals do not diminish the reliability of our description of EPG waveforms because they do not change the waveforms/behaviors performed, only their durations (Backus et al. 2018, E.A.B. unpub. data). Due to its fixed Ri and applied signal type (thus, absence of other possible settings), our EPG instrument prevented us from establishing a complete library of BMSB waveforms at different Ri levels and on different plant structures, unlike several other heteropteran EPG studies using the AC-DC electropenetrograph (e.g., Backus et al. 2013). Nonetheless, we found strong similarities between our waveforms and the ones delineated by Backus et al. (2013) when they used a 109-Ohm resistance and applied a DC current using an AC-DC electropenetrograph for chinch bugs, with the output resembling that of our DC instrument. The similarity in appearances between our waveforms and analogous ones in the literature, derived from both DC and AC-DC instruments, was supported by calculation of frequencies and relative amplitudes, allowing us to hypothesize activities of BMSB related to each described waveform (Table 1).
Epidermal scanning, waveform Hh1
At the tip of a hemipteran’s labium, sensilla are involved in chemosensation during an exploration phase of the plant. Short contacts of the labium with plant surface, a behavior usually termed “labial dabbing,” are accompanied by gelling saliva secretion (Backus 1988), and can occur while the insect is either standing still or walking (Youn et al. 2012). This behavior has previously been observed with BMSB, but could not be distinguished from stylet probing behavior, due to the technology used (Wiman et al. 2014). In our case, such labium contacts could have happened during the very irregular waveforms of non-probing phase and would not be distinguished from walking. More interestingly, we have frequently observed that the labium touched the plant surface, and then was dragged from side to side, while the insects stood still. This behavior was therefore termed “epidermal scanning.” Because we observed a voltage drop, this phase probably started with a superficial penetration or scraping of the waxy cuticle and epidermal surface of the leaf, thus making greater (but still small) electrical contact than during labial dabbing, which typically does not break the plant cuticle. We suspect that a brief burst of salivation could be occurring at the start of epidermal scanning, due to the large voltage deflection. We hypothesize that epidermal scanning phase was involved in plant testing and probing site choice before stylet penetration.
Pathway phase, waveform Hh2
The start of BMSB stylet probing was always accompanied with a large voltage drop from baseline, followed by the irregular and negative-going waveform of pathway phase. Negative voltage level, termed “intracellular” in DC EPG, supports that the stylets penetrated the leaf intracellularly, directly through epidermis and mesophyll tissue, breaking cell walls on their way. Intracellular penetration is well known for heteropterans (Backus 1988).
On many occasions, pathway events were short, directly followed by waveform Np. We interpreted that behavior as test probing into the plant, with salivary sheath secretion, but without reaching vascular tissues. These frequent short (test) probes could be attributed to a phase of acceptance of the host plant. This process has been documented for Edessa meditabunda (F.) (Lucini and Panizzi 2016) and Lygus species (Cervantes et al. 2016).
According to our EPG results, BMSB mostly performed salivary sheath feeding when on plant leaves. That category was characterized by a long pathway phase with evolving and complex waveform structure that could be further defined in future work for a waveform library, when switching Ri levels can identify electrical origins (R vs emf) and show derivation of complex structures. During such pathway waveforms, the insect secretes gelling saliva facilitating the penetration of stylets to vascular tissue (Backus et al. 2013; Lucini and Panizzi 2016).
Finally, pathway durations also could be irregular, and without evolving sub-types, resembling the cell rupture waveform of Lygus spp. (Cervantes et al. 2016). Therefore, we concluded that pathway waveform could occur during either sheath feeding or cell rupture feeding.
X wave phase
We observed two types of X waves similar to those seen with other sheath feeders. An X wave is defined as “a complex, multi-component, stereotypically repeating” waveform that “represents stylet penetration of the preferred ingestion cell type for a vascular-specializing species, either phloem sieve elements or xylem tracheary elements” (Backus et al. 2009). Importantly, the X wave represents a transition phase between pathway and sustained ingestion, in which the stylet tips move into and out of the preferred ingestion cell. Evidence from the literature supports that X wave behaviors include tasting and testing to facilitate cell acceptance for ingestion (Wayadande and Nault 1993; Backus et al. 2009; Backus 2016). Thus, X waves typically predict the eventual onset of sustained ingestion. Our study is the second time for hemipterans (Chuche et al. 2017), and the first time for heteropterans that two types of X waves were described for the same insect species. As in Chuche et al. (2017), each of the BMSB X waves preceded different-appearing, subsequent ingestion waveforms (but see the qualification for ingestion waveforms, below). This correlation allowed us to discriminate subsequent xylem ingestion from putative phloem ingestion, similar to the dual-X wave finding with grape leafhopper, Scaphoideus titanus Ball (Chuche et al. 2017).
The most frequent type of X wave (Hh3a) occurred before sustained ingestion correlated with stylet tips in the xylem (Hh4a); thus, we termed Hh3a as the xylem X wave. We found strong similarities between Hh3a and the X wave described and correlated in detail for obligate xylem-feeding sharpshooters (Auchenorrhyncha: Cicadellidae: Cicadellinae), which are vectors of the lethal bacterial phytopathogen Xylella fastidiosa (Backus et al. 2009; Backus 2016). Similarities in sub-type appearance for the BMSB xylem X wave (i.e., Hh3a-1 and -2) and the sharpshooter X wave (especially XB1s and XC1, respectively, during XN) support the hypothesis that these two xylem X waves represent similar stylet probing behaviors. Therefore, Hh3a-1 (like XB1s of sharpshooters) may represent a combination of egestion and salivation for tasting/testing the contents of the xylem cell; Hh3a-2 (like XC1) may represent brief events of cibarial pumping to test the mechanical strength of the salivary sheath connection into the xylem cell (Backus 2016). The observation of lone Hh3a-1 events during long pathway phases could imply that BMSB sampled and rejected certain xylem vessels, as has been found for sharpshooters (Backus et al. 2009).
BMSB is the first heteropteran documented to perform sharpshooter-like X waves; the grape leafhopper S. titanus (an auchenorrhynchan) also performs such X waves (Chuche et al. 2017). Because the sharpshooter X wave probably represents the X. fastidiosa inoculation behavior (Backus 2016; Backus et al. 2019), we hypothesize that BMSB might be capable of inoculating previously acquired, foregut-borne, xylem-limited phytopathogens like X. fastidiosa.
The second type of BMSB X wave (Hh3b) occurred before the second type of sustained ingestion, whose stylet tip location was not histologically verified. However, Hh3b was very similar in appearance to the one described for chinch bugs (Backus et al. 2013) and for the pentatomid E. meditabunda (Lucini and Panizzi 2016) prior to phloem ingestion. Both studies interpreted this X wave as representing stylet contact and subsequent penetration into phloem sieve elements, with likely watery salivation and/or egestion (similar to waveform E1 of aphids). Due to this similarity in X wave appearance among heteropterans, we hypothesize that Hh3b represents the putative phloem X wave.
An interesting difference between BMSB X waves and those of the comparison species (sharpshooters for Hh3a and chinch bugs/E. meditibunda for Hh3b) is that X waves did not always precede ingestion waveforms with BMSB, while 100% of ingestion waveforms for the comparison species were preceded by X waves (Backus et al. 2009). For BMSB, 54% of xylem ingestion events were preceded by the xylem X wave (Hh3a), while 70% of putative phloem ingestion events were preceded by the putative phloem X wave (Hh3b). Thus, not all sustained ingestion by BMSB required testing/tasting for cell acceptance prior to onset of cibarial pumping. To our knowledge, no other salivary sheath feeders in Hemiptera exhibit this facultative performance of X waves. However, recently the exclusively cell-rupturing feeder Lygus lineolaris (Palisot de Beauvoir) (Heteroptera: Miridae) was found to perform an X wave-like waveform T, hypothesized to represent tasting/testing to determine the degree of maceration/viscosity of ruptured tissues, prior to ingestion (Cervantes et al. 2016). Waveform T is usually (but not always) performed before an ingestion event, as a repetitive transition behavior. Therefore, we propose that X waves no longer need to be performed before 100% of ingestion events to be considered “true” X waves (Backus et al. 2009).
Ingestion phase
After more than 40 years of DC and AC-DC EPG studies, a consistent appearance of hemipteran vascular ingestion waveforms has emerged. Highly regular, rhythmic, and repeating peaks or plateaus are continuously performed for many minutes to hours at a time. Electromyographic recordings of cibarial muscles definitively associate such peaks and plateaus with rhythmic cibarial pumping (Tjallingii 1978; Dugravot et al. 2008), with each plateau caused by streaming potentials (emf components) representing one cycle of cibarial pumping (Dugravot et al. 2008). Therefore, despite a lack of stylet exudation or excretory droplet pH correlation for our BMSB ingestion waveforms, we cite strong literature precedent about waveform appearances to support that our Hh4a, Hh4b, and Hh5 waveforms represent sustained ingestion.
Xylem ingestion, Hh4a
Our histological correlation of stylet tip position for BMSB clearly identified waveform Hh4a as representing sustained ingestion from xylem cells. This finding is supported by biphasic voltage levels, in which voltage rises from intracellular (negative) to high intercellular (highly positive) levels at 109 Ohms Ri, similar to xylem ingestion in aphids (Tjallingii and Prado 2001), sharpshooters (Backus 2016), and chinch bugs (Backus et al. 2013). Furthermore, Hh4a is a high-frequency series of tall, rectangular-to-rounded plateaus (with tiny peaklets on top of the plateaus). Plateaus in a peak-and-wave structure of Hh4a match the typical appearance of xylem ingestion waveforms of heteropterans. Sharpshooter ingestion plateaus are invariant in amplitude over time (Backus 2016), while heteropteran plateaus characteristically vary in amplitude over time to form repetitive crescendos, or multi-plateau events (called waves) separated by large, positive- or negative-going peaks (e.g., Backus et al. 2013, Lucini and Panizzi 2018).
The biological meaning of plateaus is well established via sharpshooter studies, mentioned above. For both sharpshooters and heteropterans, high amplitude and frequency are characteristic of xylem ingestion to facilitate strong pumping of high-tension xylem sap (Dugravot et al. 2008, Lucini and Panizzi 2018). In contrast, the meaning of peaks is presently unknown, although the peak is a strong R component (Backus et al. 2013). Hypothesized meanings include closure of the precibarial valve to facilitate swallowing, or extreme uplift of the cibarial diaphragm to completely fill the cibarium, or both (Backus 2016). Heteropteran peak polarity (negative- or positive-going) varies by species recorded. Like BMSB, congeneric D. melacanthus and Dichelops furcatus (F.) show negative-going peaks, while three more distantly related species, E. meditabunda, Piezodorus guildinii Westwood, and Nezara viridula (L.) show positive-going peaks (Lucini and Panizzi 2018). Therefore, peak polarity, by itself, does not indicate xylem versus phloem ingestion.
Putative phloem ingestion, Hh4b
Although we were unable to histologically verify ingestion cell type for Hh4b, the appearance of the BMSB X wave (Hh3b) supports phloem sieve elements because it is similar to the X wave of chinch bugs prior to phloem sap ingestion (Backus et al. 2013). Also, the shorter amplitude and lower frequency of Hh4b, compared with Hh4a, supports phloem rather than xylem ingestion because phloem is under positive hydrostatic pressure and does not require strong pumping, unlike xylem ingestion. Finally, like the chinch bug phloem ingestion waveform, Hh3b is biphasic but rises to a level barely above baseline, not strongly above like xylem ingestion.
Despite the above evidence for Hh4b representing phloem ingestion, the appearance of Hh4b (peak-and-wave with positive-going peaks) is like no other heteropteran phloem ingestion waveform published. Typically, phloem ingestion (when it occurs) is represented by rhythmic, repetitive peaks of short, invariant amplitude, as with chinch bugs (Backus et al. 2013) or E. meditabunda (Lucini and Panizzi 2016, 2018). Interestingly, the phloem ingestion waveform of the leafhopper Dalbulus maidis (DeLong and Wolcott) (verified by excretory droplet pH and Spiroplasma acquisition from phloem) displays plateaus with amplitude variation over time that form a wave-like structure, but lacking intermittent peaks (Carpane et al. 2011); thus, wave-like appearance is possible for phloem ingestion, but previously undocumented for heteropterans. Nonetheless, without excretory droplet pH or histological verification, Hh4b should be considered putative phloem ingestion until further correlations (and/or a complete waveform library to ascertain electrical origins) can be performed.
Putative macerate ingestion, Hh5
We propose that waveform Hh5 represents culmination of a cell-rupturing process for the sustained ingestion of macerated mesophyll, due to the absence of a preceding X wave plus its general resemblance to the Dm3a waveform of another pentatomid, Dichelops melacanthus (Dallas). Dm3a was histologically correlated with slow movement of stylets in parenchyma/mesophyll, a hallmark of cell rupturing (Lucini and Panizzi 2017). The appearance of Hh5 (i.e., slightly varying amplitudes of tight plateaus with tiny peaks on the top but lacking a wave structure) also resembles combined salivation into and ingestion from phloem sieve elements (waveform E2) by aphids (Tjallingii and Prado 2001). Therefore, we hypothesize that Hh5 represents salivation/maceration of non-vascular tissues and simultaneous ingestion of macerate. However, lacking either histological or excretory droplet pH verification, our interpretation of Hh5, like Hh4b, is also putative.
Summary of ingestion findings
In summary, putative phloem versus xylem waveforms could be distinguished by their characteristics (higher amplitude and frequency plus negative-going peaks for Hh4a, xylem ingestion, whereas lower amplitude and frequency plus positive-going peaks for Hh4b, putative phloem ingestion), as well as the waveforms (i.e., X waves) that often preceded them (Backus et al. 2013; Lucini and Panizzi 2016). Putative salivation into and ingestion of macerate from cell-ruptured parenchyma/mesophyll, waveform Hh5, also occurred.
Conclusions
In this study, we documented for the first time for any heteropteran that, on the same plant structure, BMSB can utilize two different strategies of plant feeding (sheath feeding and cell rupture feeding), probably exploiting three different cell types (xylem cells, phloem sieve elements, and mesophyll/parenchyma). Some aspects of this plasticity have been documented before for heteropterans. For example, switching between two feeding strategies according to the feeding site (stems/leaves or pods) has been observed with other pentatomids (Lucini and Panizzi 2018). Previously, only D. melacanthus also applied both strategies on the same plant structure, namely maize stem (Lucini and Panizzi 2017), but it only ingested from xylem, not phloem. E. meditabunda on soybean stem ingested both phloem and xylem saps, favoring the latter; however, it did not perform cell rupturing (Lucini and Panizzi 2016). At the opposite extreme for sheath feeders, P. guildinii ingested exclusively from xylem when placed on soybean leaves (Lucini et al. 2016). The present comparison with the published literature enlightened us on the uniquely large plasticity of BMSB feeding strategies, which probably helps to explain the strong pest status of this highly polyphagous, invasive pest that can feed on many plant species and structures. Future EPG studies on BMSB, now allowed by our benchmark information, will better be able to investigate bug–host plant interactions for improved pest management tools.
References
Backus EA (1988) Sensory systems and behaviours which mediate hemipteran plant-feeding: a taxonomic overview. J Insect Physiol 34:151–165. https://doi.org/10.1016/0022-1910(88)90045-5
Backus EA (2016) Sharpshooter feeding behavior in relation to transmission of Xylella fastidiosa: a model for foregut-borne transmission mechanisms. In: Brown JK (ed), Vector-mediated transmission of plant pathogen. American Phytopathological Society, St. Paul, MN, 496 pp
Backus EA, Serrano MS, Ranger CM (2005) Mechanisms of hopperburn: an overview of insect taxonomy, behavior, and physiology. Annu Rev Entomol 50:125–151. https://doi.org/10.1146/annurev.ento.49.061802.123310
Backus EA, Holmes WJ, Schreiber F et al (2009) Sharpshooter X wave: correlation of an electrical penetration graph waveform with xylem penetration supports a hypothesized mechanism for Xylella fastidiosa inoculation. Ann Entomol Soc Am 102:847–867
Backus EA, Rangasamy M, Stamm M et al (2013) Waveform library for chinch bugs (Hemiptera: Heteroptera: Blissidae): characterization of electrical penetration graph waveforms at multiple input impedances. Ann Entomol Soc Am 106:524–539
Backus EA, Cervantes FA, Godfrey L et al (2018) Certain applied electrical signals during EPG cause negative effects on stylet probing behaviors by adult Lygus lineolaris (Hemiptera: Miridae). J Insect Physiol 105:64–75. https://doi.org/10.1016/j.jinsphys.2017.12.006
Backus EA, Cervantes FA, Guedes RNC et al (2019) AC–DC electropenetrography for in-depth studies of feeding and oviposition behaviors. Ann Entomol Soc Am 112:236–248. https://doi.org/10.1093/aesa/saz009
Carpane P, Wayadande A, Backus EA et al (2011) Characterization and correlation of new electrical penetration graph waveforms for the corn leafhopper (Hemiptera: Cicadellidae). Ann Entomol Soc Am 104:515–525. https://doi.org/10.1603/an10052
Cervantes FA, Backus EA, Godfrey L et al (2016) Characterization of an EPG waveform library for adult Lygus lineolaris and Lygus hesperus (Hemiptera: Miridae) feeding on cotton squares. Ann Entomol Soc Am 109:684–697. https://doi.org/10.1093/aesa/saw039
Chuche J, Backus EA, Thiéry D, Sauvion N (2017) First finding of a dual-meaning X wave for phloem and xylem fluid ingestion: characterization of Scaphoideus titanus (Hemiptera: Cicadellidae) EPG waveforms. J Insect Physiol 102:50–61. https://doi.org/10.1016/j.jinsphys.2017.01.013
Dugravot S, Backus EA, Reardon BJ, Miller TA (2008) Correlations of cibarial muscle activities of Homalodisca spp. Sharpshooters (Hemiptera: Cicadellidae) with EPG ingestion waveform and excretion. J Insect Physiol 54:1467–1478. https://doi.org/10.1016/j.jinsphys.2008.05.008
Lee D-H, Short BD, Joseph SV et al (2013) Review of the biology, ecology, and management of Halyomorpha halys (Hemiptera: Pentatomidae) in China, Japan, and the Republic of Korea. Environ Entomol 42:627–641
Lucini T, Panizzi AR (2016) Waveform characterization of the soybean stem feeder Edessa meditabunda: overcoming the challenge of wiring pentatomids for EPG. Entomol Exp Appl 158:118–132. https://doi.org/10.1111/eea.12389
Lucini T, Panizzi AR (2017) Feeding behavior of the stink bug Dichelops melacanthus (Heteroptera: Pentatomidae) on maize seedlings: an EPG analysis at multiple input impedances and histology correlation. Ann Entomol Soc Am 110:160–171. https://doi.org/10.1093/aesa/saw070
Lucini T, Panizzi AR (2018) Electropenetrography (EPG): a breakthrough tool unveiling stink bug (Pentatomidae) feeding on plants. Neotrop Entomol 47:6–18. https://doi.org/10.1007/s13744-017-0574-3
Lucini T, Panizzi AR, Backus EA (2016) Characterization of an EPG waveform library for redbanded stink bug, Piezodorus guildinii (Hemiptera: Pentatomidae), on soybean plants. Ann Entomol Soc Am 109:198–210. https://doi.org/10.1093/aesa/sav156
Maistrello L, Dioli P, Bariselli M et al (2016) Citizen science and early detection of invasive species: phenology of first occurrences of Halyomorpha halys in Southern Europe. Biol Invasions 18:3109–3116. https://doi.org/10.1007/s10530-016-1217-z
Musolin DL, Konjević A, Karpun NN et al (2018) Invasive brown marmorated stink bug Halyomorpha halys (Stål) (Heteroptera: Pentatomidae) in Russia, Abkhazia, and Serbia: history of invasion, range expansion, early stages of establishment, and first records of damage to local crops. Arthropod Plant Interact 12:517–529. https://doi.org/10.1007/s11829-017-9583-8
Rice KB, Bergh CJ, Bergmann EJ et al (2014) Biology, ecology, and management of brown marmorated stink bug (Hemiptera: Pentatomidae). J Integr Pest Manag 5:1–13. https://doi.org/10.1603/IPM14002
Tjallingii WF (1978) Electronic recording of penetration behaviour by aphids. Entomol Exp Appl 24:521–530
Tjallingii WF, Prado E (2001) Analysis of circulative transmission by electrical penetration graphs. Virus Insect Plant Interact. https://doi.org/10.1016/b978-012327681-0/50007-8
Wayadande AC, Nault LR (1993) Leafhopper probing behavior associated with maize chlorotic dwarf virus transmission to maize. Phytochemistry 83:522–526
Wermelinger B, Wyniger D, Forster B (2008) First records of an invasive bug in Europe: Halyomorpha halys Stål (Heteroptera: Pentatomidae), a new pest on woody ornamentals and fruit trees? Mitteilungen der Schweizerischen Entomol Gesellschaft 81:1–8
Wiman NG, Walton VM, Shearer PW, Rondon SI (2014) Electronically monitored labial dabbing and stylet ‘probing’ behaviors of brown marmorated stink bug, Halyomorpha halys, in simulated environments. PLoS ONE 9:e113514. https://doi.org/10.1371/journal.pone.0113514
Youn Y, Backus EA, Serikawa RH, Stelinski LL (2012) Correlation of an Electrical Penetration Graph Waveform with Walking by Asian Citrus Psyllid, Diaphorina citri (Hemiptera: Psyllidae). Florida Entomol 94:1084–1087. https://doi.org/10.1653/024.094.0456
Author information
Authors and Affiliations
Contributions
LS, LP, and FF conceived and designed research. LS and LP conducted experiments. LS, LP, and EAB analyzed data. LS and EAB wrote the manuscript. All authors read and approved the manuscript.
Corresponding author
Ethics declarations
Conflict of interest
The authors declare that they have no conflict of interest.
Additional information
Handling Editor: Chen-Zhu Wang.
Publisher's Note
Springer Nature remains neutral with regard to jurisdictional claims in published maps and institutional affiliations.
Elaine A. Backus: Mention of trade names or commercial products in this publication is solely for the purpose of providing specific information and does not imply recommendation or endorsement by the U.S. Department of Agriculture. USDA is an equal opportunity provider and employer.
Rights and permissions
About this article
Cite this article
Serteyn, L., Ponnet, L., Backus, E.A. et al. Characterization of electropenetrography waveforms for the invasive heteropteran pest, Halyomorpha halys, on Vicia faba leaves. Arthropod-Plant Interactions 14, 113–126 (2020). https://doi.org/10.1007/s11829-019-09722-y
Received:
Accepted:
Published:
Issue Date:
DOI: https://doi.org/10.1007/s11829-019-09722-y