Abstract
Acrolein, which is an irritating and off-flavor compound formed during heating of vegetable oils, was estimated by the gas–liquid chromatography (GLC). Several vegetable oils such as high-oleic sunflower, perilla, rapeseed, rice bran, and soybean oils were heated at 180 °C for 480 min and then the concentration of acrolein in the head space gas was determined by GLC. The formation of acrolein was greatest in perilla oil among the tested oils, while it was much lower in rice bran oil and high-oleic sunflower oil. There was a good correlation between the level of acrolein and linolenate (18:3n-3) in the vegetable oils. To investigate the formation of acrolein from linolenate, methyl oleate, methyl linoleate, and methyl linolenate were also heated at 180 °C, and the amounts of acrolein formed from them were determined by GLC. The level of acrolein was the greatest in methyl linolenate. Acrolein was also formed from methyl linoleate, but not from methyl oleate. Acrolein in vegetable oils may be formed from polyunsaturated fatty acids, especially linolenic acid but not from glycerol backbone in triacylglycerols.
Similar content being viewed by others
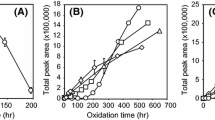
Explore related subjects
Discover the latest articles, news and stories from top researchers in related subjects.Avoid common mistakes on your manuscript.
Introduction
Vegetable oils such as olive, rapeseed, and soybean, are often used for heat cooking as well as for mayonnaise and salad dressing. Heat cooking such as deep- and pan-frying with vegetable oils contributes both desirable and undesirable flavors to foods. The formation of desirable flavors is necessary for the fried foods to acquire their unique sensory characteristics. However, extensive heating causes oxidative degradation of vegetable oils, which will not only reduce the sensory quality of fried foods but may also diminish their nutritional value. Moreover, some volatile compounds such as acrolein produced during prolonged frying, may have a harmful influence on the health of cooks, because the LD50 (oral) and odor threshold of acrolein are 82 mg/kg and 3.6 ppb, respectively.
The thermal oxidation of unsaturated fatty acids mainly contributes to thermal degradation of vegetable oils among constituent fatty acids. At high temperature, hydroperoxides, the primary oxidation products, do not accumulate in the frying oil because of their instability, although the formation of hydroperoxides from unsaturated fatty acids is accelerated. Hydroperoxides are spontaneously decomposed to form volatile compounds as well as polymers. These volatile compounds contribute to desirable and undesirable flavors. Volatile compounds produced by thermal oxidation include aldehydes, ketones, alcohols, acids, esters, and hydrocarbons. Gas chromatographic analyses of fats and oils after frying revealed that 2,4-hepatadienal derived from linolenate and hexanal derived from linoleate may cause undesirable rancidity among some volatile compounds [1].
Acrolein (2-propenal), one of unsaturated aldehydes, may give undesirable and irritated odors, besides toxicity [2, 3]. It is well known that acrolein vapor may cause eye, nasal, and respiratory tract irritations. There are several reviews of the toxicity of acrolein. Acrolein may induce epithelial cell injury and inhibition of macrophage [4, 5]. It can also cause membrane damage and mitochondrial dysfunction, and myelin disruption in the nervous system [6]. Moreover, acrolein and other α, β-unsaturated aldehydes endogenously formed during lipid peroxidation may have mutagenicity, because they may induce DNA and protein damages by forming DNA and cellular compound adducts [7–9].
It is well known that acrolein may be formed during frying with fats and oils. Fritsch [10] provided a diagram that summarized the route through which triglycerides degrade. Acrolein may be formed by the dehydration of the glycerol remaining after three fatty acids residues have hydrolyzed from the triglyceride molecule. However, there have been few reports on the quantitative analysis of acrolein during frying of vegetable oils. Ota et al. [11] measured volatile compounds including acrolein from soybean oil during heating at high temperature. Hirayama et al. [12] observed the formation of acrolein by autoxidation of unsaturated fatty acid methyl esters using a fluorescent method. Miyake and Shibamoto [13] observed the formation of acrolein from unsaturated lipids oxidized with Fenton’s reagent. However, they did not always directly determine the acrolein, and the quantitative data were inadequate. Moreover, the mechanism for the formation of acrolein from vegetable oils and unsaturated fatty acids during oxidation was not discussed. In this paper, we have tried to quantify acrolein formed during heating of vegetable oils at high temperature, and discuss the mechanism for the formation of acrolein in vegetable oils.
Materials and Methods
Vegetable Oils
RBD rapeseed (The Nisshin OilliO Group, Japan), soybean (Riken Nosan Kako, Japan), perilla oils (Ohta Oil Mill, Japan) and high-oleic sunflower oils (Showa Sangyo, Japan) were purchased in a market. RBD rice bran oil was prepared by our company. Methyl oleate, methyl linoleate, and methyl linolenate (purity >99 %) were purchased from Sigma Aldrich Japan. Tridecanoylglycerol and acrolein (purity >99 %) were purchased from Sigma Aldrich Japan.
Methyl Linoleneate Monohydroperoxides
Methyl linolenate was auto-oxidized at 40 °C for 2 days, and the methyl linolenate monohydroperoxides were isolated with a silicic acid column chromatograph using a mixture of n-hexane and diethyl ether as eluents.
Heating Test of Vegetable Oils
Two grams of vegetable oils were put in a 20-mL glass vial and heated in an aluminum block bath at 180 °C for 0–480 min. Heating tests were also performed at 150 and 220 °C. Methyl esters of unsaturated fatty acids (0.5 g) or tridecanoylglycerol (0.5 g) supplemented with different amounts of methyl linolenate monohydroperoxides were put in a 20-mL glass vial, and were then heated at 180 °C for 20 min.
Collection of Acrolein
After heating, vegetable oils, methyl esters of unsaturated fatty acids, or tridecanoylglycerol supplemented with methyl linolenate monohydroperoxides in a 20-mL glass vial were sealed with a metal cap. The vial was heated at 55 °C for 10 min, and then 1.0 mL of head space gas was injected into the gas chromatograph.
Gas Chromatographic Analysis
A HERACLES II gas chromatograph (Alpha MOS, Japan) equipped with a FID detector was used. For the columns, 18 m × 0.18 mm i.d. DB-5 or WAX fused silica capillary columns were used. The injector temperature was 220 °C and the detector temperature was 250 °C. The column was held at 40 °C and then programmed to 250 °C at 3 °C/s after the injection of head space gas. Hydrogen gas was used as a carrier gas. When using the WAX capillary column, acrolein eluted at 21 s.
Quantification of Acrolein
Different amounts of acrolein were added to tridecanoylglycerol and heated at 55 °C under the same conditions as the vegetable oils to make a calibration curve. Amounts of acrolein in vegetable oils after heating was determined from the calibration curve. Experiments were performed in triplicate. All data described the average, because the relative standard deviation was below 10 %.
Results and Discussion
Figure 1 shows the gas chromatogram of the head space gas obtained from rapeseed and rice bran oils after heating at 180 °C for 120 min, when the WAX capillary column was used. The peak of acrolein was eluted at Rt. 21 s, and it was absolutely separated from those of propanal (Rt. 18 s) and other volatile compounds. They were also confirmed by GC–MS. Acrolein and propanal were detected as major volatile compounds on a gas chromatogram of rapeseed and rice bran oils whereas octane and ethanol were also detected at Rt. 15 and 26 s, respectively.
Figure 2 shows a calibration curve of acrolein. The concentration of acrolein correlated linearly with the peak area at the range between 10 and 3,000 ppb. The correlation coefficient (R 2) was 0.999. These results indicate this method is effective for quantification of acrolein in vegetable oils.
Figures 3, 4, 5, and 6 show the amounts of acrolein formed during heating of vegetable oils at 150, 180, and 220 °C. The amounts of acrolein increased with heating time in regardless of variety of vegetable oils at 150 °C (Fig. 3). Perilla oil showed the greatest level of acrolein during heating among tested oils. Acrolein level in perilla oil reached at 1,870 ppb after heating for 480 min. On the other hand, acrolein levels in rapeseed and soybean oil were 1,130 and 830 ppb, respectively. In particular, the levels of acrolein in rice bran and high-oleic sunflower oils were 300 and 210 ppb, respectively.
When vegetable oils were heated at 180 °C, all vegetable oils produced more acrolein than heating at 150 °C (Fig. 4). Among tested oils, perilla oil showed the greatest level of acrolein (3,440 ppb) after heating for 240 min, and then the level of acrolein in perilla oil decreased. Acrolein in rapeseed and soybean oils reached at the maximum (1,960 and 1,760 ppb, respectively) after heating for 240 min, and then the level of acrolein decreased. On the other hand, the levels of acrolein in rice bran and high-oleic sunflower were lower than other vegetable oils (700 and 340 ppb at maximum, respectively), although the level of acrolein at 180 °C was greater than heating at 150 °C.
When vegetable oils were heated at 220 °C, all vegetable oils had greater level of acrolein than heating at 150 and 180 °C (Fig. 5). Perilla oil showed the greatest level of acrolein (4,160 ppb) among tested oils after heating for 30 min, and then the level of acrolein decreased rapidly. The acrolein in rapeseed oil reached at the maximum (2,630 ppb) after heating for 90 min, while that in soybean seed oil reached at the maximum (2,230 ppb) after heating for 45 min. The acrolein in rice bran and high-oleic sunflower oils reached at the maximum (960 and 400 ppb, respectively) after heating for 90 min, although the level was lower than those in perilla, rapeseed, and soybean oils.
These results showed that the level of acrolein formed in vegetable oils depended on types of vegetable oils, and they did not agree with Fritsch’s hypothesis. Our results suggested that acrolein did not derive from a glycerol backbone in triacylglycerol. Moreover, the level of acrolein formed in vegetable oils had a limit, although the level of acrolein in vegetable oils increased with heating temperature. Probably, acrolein might decompose into malondialdehyde and other compounds during heating. Rapid decrease of acrolein at higher temperature may be due to the poor thermal stability or the decrease of linolenate as a source of acrolein.
Anyway, these observations suggest that cooking with vegetable oils at high temperature is undesirable for cooks because it may induce the formation of acrolein.
Table 1 shows the fatty acid composition of vegetable oils tested. The level of α-linolenic acid (18:3n-3) was the greatest in perilla oil (58 %), while those in rice bran and high-oleic sunflower oils were very low (below 1 %). The level of acrolein produced from vegetable oils during heating seemed to be correlated with the level of 18:3n-3 in them. Thus, the relationship between the level of acrolein and 18:3n-3 in vegetable oils was investigated. Figure 6 shows the relationship between the highest value of acrolein and the concentration of 18:3n-3 in vegetable oils. As shown in Fig. 6, good correlation coefficients (150 °C, R 2 = 0.859; 180 °C, R 2 = 0.916; 220 °C, R 2 = 0.939) were observed. These results suggested that acrolein might be formed from 18:3n-3 but not glycerol backbone during heating of vegetable oils at high temperature.
To confirm the contribution of 18:3n-3 to the formation of acrolein, we determined the level of acrolein in methyl esters of unsaturated fatty acid such as linolenic (18:3n-3), linoleic (18:2n-6), and oleic (18:1n-9) acids after heating. Table 2 shows the level of acrolein in methyl linolenate, methyl linoleate, and methyl oleate at 180 °C for 20 min. The level of acrolein was the greatest in methyl linolenate (2,790 ppb), followed by methyl linoleate (820 ppb), and methyl oleate (30 ppb). The relative ratios of acrolein formed from methyl linolenate, methyl linoleate, and methyl oleate were 93:27:1. The formation of acrolein from polyunsaturated fatty acid methyl esters was very faster than those of hydroperoxides during autoxidation of them [14]. This result demonstrates that acrolein could be selectively formed from polyunsaturated fatty acids such as α-linolenic (18:3n-3) and linoleic (18:2n-6) acids. Especially, it was suggested that 18:3n-3 containing in vegetable oil could contribute mainly to the formation of acrolein during heating at high temperature.
We assumed the mechanism of formation of acrolein from 18:3n-3 at high temperature, as shown in Fig. 7. It is well known that polyunsaturated fatty acids readily produce hydroperoxides during oxidation. They also produce cyclic hydroperoxides and dihydroperoxides as secondary oxidation products via monohydroperoxides [14]. In particular, 18:3n-3 produces more secondary oxidation products than 18:2n-6 due to its more double bonds. We conjectured that dihydroperoxides and hydroperoxy endoperoxides could be formed in thermal oxidation of 18:3n-3, although their presence could not be confirmed because of their instability at high temperature. Probably, acrolein might be formed by the cleavage of dihydroperoxides and hydroperoxy epidioxides via monohydroperoxides from 18:3n-3 (Fig. 7), while it might also derive from hydroperoxy epidioxides from 18:2n-6. Unfortunately, acrolein has not been found in thermal degradation of dihydroperoxides and hydroperoxy epidioxides of unsaturated fatty acids. However, acrolein seemed to be formed from secondary oxidation products via monohydroperoxides of polyunsaturated fatty acids, because 18:1n-9 which hardly gave secondary oxidation products did not almost produce acrolein. Indeed, the formation ratio of acrolein from polyunsaturated fatty acids was markedly greater than the predicted formation ratio of their hydroperoxides, as shown in Table 2. In a previous paper, we observed that acrolein was not almost formed in vegetable oils during heating at 180 °C in the absence of oxygen [15]. These results indicated that the thermal oxidation of polyunsaturated fatty acids could produce acrolein.
Therefore, we added isolated methyl linoleneate monohydroperoxides into tridecanoylglycerol, and determined the level of acrolein after heating at 180 °C for 20 min, as a model experiment. As shown in Table 3, acrolein increased with addition of methyl linolenate monohydroperoxides. The yield of acrolein from methyl linolenate monohydroperoxides was 17 ppm, and it was much higher than that from methyl linolenate (2.8 ppm). This data demonstrated that acrolein could be formed from linolenate via monohydroperoxides and it assisted the conjectured mechanism for formation of acrolein during heating of vegetable oils, as showed in Fig. 7.
In this study, we observed that the level of acrolein formed during heating of vegetable oils at high temperature correlated with the concentration of 18:3n-3 in them. In a model experiment using unsaturated fatty acid methyl esters, 18:2n-6 could also form acrolein during heating as well as 18:3n-3. Vegetable oils contain considerable amounts of natural antioxidants such as tocopherols and tocotrienol, so that 18:3n-3 which is more susceptible to oxidation than 18:2n-6 could be a major target of thermal oxidation.
Many researchers have believed that acrolein may be derived from a glycerol backbone in triacylglycerol during frying with vegetable oils. However, our results demonstrated that acrolein was mainly derived from 18:3n-3 but not a glycerol backbone, because methyl linolenate formed considerable amounts of acrolein during heating at high temperature, and the amounts of acrolein in vegetable oil depended on the concentration of 18:3n-3 in them.
In the next study, we are trying to develop the method to inhibit the formation of acrolein from 18:3n-3 during heating of vegetable oils, in order to also clarify the detailed mechanism for the formation of acrolein during cooking with vegetable oils.
References
Takeoka G, Perrino C, Buttery R (1996) Volatile constituents of used frying oils. J Agric Food Chem 44:654–660
Gasee FR, Stenhuis WH, Groten JP, Freton VJ (1996) Toxicity of formaldehyde and acrolein mixtures: in vitro studies using nasal epithelial cells. Exp Toxic Pathol 48:481–483
Uchida K (1999) Current status of acrolein as a lipid peroxidation product. Trends Cardiovasc Med 9:109–113
Li L, Holian A (1998) Acrolein: a respiratory toxin that suppresses pulmonary host defense. Rev Environ Health 13:88–108
Faroon O, Roney N, Taylor J, Ashizawa A, Lumpkin MH, Plewak DJ (2008) Acrolein health effects. Toxicol Ind Health 24:447–490
Shi R, Rickwtt T, Sun W (2011) Acrolein-mediated injury in nervous system trauma and diseases. Mol Nutr Food Res 55:1320–1321
Liu XY, Zhu MX, Xie JP (2010) Mutagenicity of acrolein and acrolein-induced DNA adducts. Toxicol Mech Methods 20:36–44
Abraham K, Andres S, Palavinskas R, Berg K, Appel KE, Lampen A (2011) Toxicology and risk assessment of acrolein in food. Mol Nutr Food Res 55:1277–1290
Tang MS, Wang HT, Hu Y, Chen WS, Akao M, Feng Z, Hu W (2011) Acrolein induced DNA damage, mutagenicity and effect on DNA repair. Mol Nutr Food Res 55:1291–1300
Fritsch CW (1981) Measurements of frying fat deterioration: a brief review. J Am Oil Chem Soc 58:272–274
Ota S, Iwata N, Mukai A, Enei H (1963) Volatile products of edible oils by open air heating IV: volatile substances from soybean oil at different stages of thermal oxidation. J Jpn Oil Chem Soc 12:403–408 (Japanese)
Hirayama T, Yamaguchi M, Nakata T, Okumura M, Yamasaki T, Watanabe T, Fukui S (1989) Formation of acrolein by the autoxidation of unsaturated fatty acid methyl esters. Eisei Kagaku 35:303–306 (Japanese)
Miyake T, Shibamoto T (1996) Simultaneous determination of acrolein, malonaldehyde and 4-hydroxy-2-nonenal produced from lipids oxidized with Fenton’s reagent. Food Chem Toxicol 34:1009–1011
Frankel EN (1998) Lipid oxidation. Oily, Dundee
Fujisaki M, Endo Y, Fujimoto K (2002) Retardation of volatile aldehyde formation in the exhaust of frying oil by heating under low oxygen atmospheres. J Am Oil Chem Soc 79:909–914
Author information
Authors and Affiliations
Corresponding author
About this article
Cite this article
Endo, Y., Hayashi, C., Yamanaka, T. et al. Linolenic Acid as the Main Source of Acrolein Formed During Heating of Vegetable Oils. J Am Oil Chem Soc 90, 959–964 (2013). https://doi.org/10.1007/s11746-013-2242-z
Received:
Revised:
Accepted:
Published:
Issue Date:
DOI: https://doi.org/10.1007/s11746-013-2242-z