Abstract
The compressive yield stress of Fe-26Al with additives Ti (0.5 to 4 at. pct), Cr (0.5 to 8 at. pct), Mo (0.5 to 4 at. pct), and V (0.5 to 8 at. pct) at 1073 K (800 °C) has been determined. The effect of the concentration of diverse solutes on the yield stress at 1073 K (800 °C) was compared, and the additivity of the effects of solutes was tested. The effects in iron aluminides with two solutes (V and Ti, Ti and Cr, V and Cr) are compared with those of a single solute V, Ti, and Cr. It is found that the additivity of yield stress increments is valid only for lower solute concentrations. When the amount of the solute atoms increases, the yield stress increment is substantially higher than the sum of the yield stress increments of single solutes. This behavior is related to the high-temperature order in iron aluminides.
Similar content being viewed by others
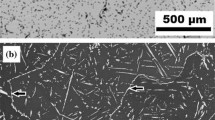
Avoid common mistakes on your manuscript.
1 Introduction
Alloys based on iron aluminides Fe3Al show potential for structural applications at high temperatures owing to their excellent oxidation and sulfidation resistance. They display lower density compared to other iron-based materials, and their low cost is advantageous as well. Unfortunately, they also show unfavorable lack of room temperature ductility and low high-temperature strength.[1,2,3,4,5]
During the last decades, research efforts have focused on enhancing the ductility, strength, and creep resistance of iron aluminides by alloying. Several approaches have been explored to improve the high-temperature mechanical properties of these alloys. Basically, solid solution hardening (SSH), strengthening by coherent and incoherent precipitates, and increasing the crystallographic order were considered for the strengthening of iron aluminides.[1,6–9] Elements such as Cr, Ti, Mn, Si, Mo, V, and Ni were added for SSH[2,10,11,12] and Palm[8] compared the SSH by Ti, V, Cr, and Mo at 873 K, 973 K, and 1073 K (600°C, 700°C, and 800 °C). Recently, Kratochvil et al.[12] described the SSH effect of vanadium in Fe3Al using the SSH theories for binary alloys of Fleischer[13] and Labusch.[14] It was reported that the SSH of Fe3Al by vanadium depends mostly on the elastic modulus misfit, and the atom size misfit plays a minor role.
In this work, we first describe the SSH of Fe3Al by Ti, Cr, V, and Mo solutes. This is followed by the study of the combined effect of two solutes in Fe3Al.
2 Experimental
Iron aluminide alloys were produced by vacuum induction melting. The cooling took place under argon. Samples were prepared from the alloys by electrical discharge machining (EDM). The compressive yield stress was evaluated using a digitally controlled testing machine (INSTRON 1186R). Parallelepipeds with dimensions of 6 × 6 × 10 mm3 were cut by EDM. The deformation rate was 8 × 10−5 s−1. The temperature of 1073 K (800 °C) was chosen because this temperature is well above the yield strength anomaly, which is an unusual increase of the strength with increasing temperature, typically observed for Fe-Al-based alloys with the maximum in the 773 to 873 K (500 to 600 °C) range.[15] Additionally, 1073 K (800 °C) is high enough for the vacancy concentration to be in thermal equilibrium. This is noteworthy because at lower temperatures the strength of Fe-Al-based alloys is markedly influenced by quenched-in thermal vacancies,[16] i.e., the strength depends on the processing of the alloys. The investigated alloys are B2-ordered at 1073 K (800 °C), allowing direct comparison of their yield strengths.
To reveal the structure of the investigated materials at elevated temperatures, X-ray diffraction measurements at high temperatures were performed. Powdered samples were placed into an AlN crucible and heated up to 1073 K (800 °C) using a radiant heater in vacuum. A high-temperature chamber MRI TC-Basics was placed onto a goniometer of the Panalytical X’Pert MPD diffractometer in the Bragg-Brentano geometry. CuKα radiation was used, and diffracted rays were detected using a gas-filled proportional detector equipped with a graphite monochromator. A constant irradiated area of 3 × 5 mm2 was held during the measurements using automatic divergence slits.
The X-ray study of the microstructure was complemented by transmission electron microscope (TEM) observations. Slices about 0.5 mm thick were cut from the bulk material (as-cast state). These were further ground to the thickness of about 0.1 mm and electro-polished under 15 V at −30 °C (243 K) in 20 pct solution of nitric acid (HNO3) in methyl alcohol (CH3OH) to prepare TEM specimens. TEM observations were performed on JEOL 2000FX working at 200 kV.
3 Results and Discussion
3.1 Experiments with Single Solutes
The compositions of the tested alloys were determined by wet chemical analysis (Table I). These alloys show comparable Al content (25.5 ± 0.3 at. pct) and are denoted FA. The C content (0.03 at. pct) is so low that it has no noticeable effect on the yield strength. All alloys are single-phase at room temperature and exhibit a polygonal grain structure with large grains, with sizes between 150 and 500 μm.
First, the influence of Mo, Ti, Cr, V solutes on the increase of the yield stress σ 0.2 at 1073 K (800 °C) was determined. The values for these ternary alloys are summarized in Table II. The dependencies can be described as functions of cn (Figure 1). In the case of Cr and Ti, n is equal to 1. For vanadium, n = ½ was obtained.[12]
3.2 Experiments with Two Solutes in Fe3Al
The investigation of a combined effect of two solutes on the hardening of Fe3Al (quaternary alloy) was the next step. The compositions were determined by wet chemical analysis of seven tested alloys and are presented in Table III. The structure of all alloys at 1073 K (800 °C)—the testing temperature—was determined by XRD of powder samples (Figure 2). The data for the 3 alloys investigated by Stein et al.[17] are added in Table V. Nominal compositions are listed for these 3 alloys in Table IV.
It is obvious that the combination of two solutes Ti and V, and as well of Cr and V, increases the transformation temperature D03→B2 to the temperatures higher than 1073 K (800 °C). In addition to X-ray diffraction, the structure of the Fe3Al-10V-10Cr alloy was also investigated by TEM (Figure 3). The TEM observations are in an agreement with the X-ray results and confirm that the structure is ordered, with a D03 symmetry (Figure 3(a)). Ordering in this material is also documented by the presence of both types of anti-phase boundaries (APB) (Figure 3(b)).
Then, the values of the yield stress σ 0.2 at 1073 K (800 °C) in the quaternary systems (Fe3Al-Cr-V and Fe3Al-Ti-V) are compared to the corresponding values of the ternary alloys, i.e., the yield stress σ 0.2 of quaternary Fe3Al-xTi-yV is compared to the sum of individual contributions of Ti and V to the yield stress σ 0.2 determined for ternary alloys Fe3Al-xTi and Fe3Al-yV.
The measured values of σ 0.2 for alloys with two solutes in Fe3Al at 1073 K (800 °C) are given in Table V (column 1). These values are compared to the sum of the measured yield stress σ 0.2 at 1073 K (800 °C) for Fe3Al-xTi and of the increment yield stress Δσ 0.2 at 1073 K (800 °C) for Fe3Al-xV. The increment of the yield stress Δσ 0.2 is equal to the value of the yield stress σ 0.2 for Fe3Al-xV lowered by the value of the yield stress σ 0.2 of the matrix Fe3Al that is approximately 23 MPa.[12] The data presented in the third and fourth columns of Table V as σ 0.2 exp and Δσ 0.2 exp, respectively, are interpolated or extrapolated from the dependencies shown in Figure 1 for the value of the respective concentration c.
It is found that for low values of x and y up to ~ 2 to 4 at. pct, the values σ 0.2 at 1073 K (800 °C) of the quaternary alloys Fe3Al-xTi-yV are the same as the sums of the respective σ 0.2 exp and Δσ 0.2 exp of the ternary alloys. This is also true for the quaternary system Fe-Al-Cr-V.
The value of σ 0.2 at 1073 K (800 °C) for Fe-26Al-4Ti-2V given in Reference 17 (~190 MPa) is similar to that measured in this work (compare line two in Table V). In the same paper,[17] the value of σ 0.2 at 1073 K (800 °C) for Fe-26Al-4Ti-2Cr is given as ~120 MPa. This is very close to the value of σ 0.2 at 1073 K (800 °C) for Fe-26Al-4Ti (see Table II and Figure 1) owing to the very small Δσ 0.2 contributed by Cr (for Fe3Al-2Cr it is 2 to 5 MPa).
This is true unless the concentration is increased over a certain, not yet determined limit. The structure of the Fe3Al-4 at. pct Ti-4 at. pct V and Fe3Al-8 at. pct Ti-8 at. pct V alloys is D03− (L21−) ordered at 1073 K (800 °C), as shown in Figure 2(a). The diffraction curves at 1123 K, 1223 K, and 1323 K (850 °C, 950 °C, and 1050 °C) are available for Fe3Al-8 at. pct Ti-8 at. pctV and show a D03 (L21) order, in agreement with the phase transformation at 1423 K (1150 °C) detected by DTA.[18]
The same situation is observed for the alloy Fe3Al-10 at. pct Cr-10 at. pct V. The additivity of the yield stresses 0.2 at 1073 K (800 °C) is no longer valid. The increased strengthening effect can also here be connected with D03 (L21) order (see Figure 2(b)). The ordering effect of each of V and Cr (i.e., the effect on increase of D03↔B2 temperature in iron aluminide Fe3Al), by 1 to 4 at. pct of both elements, was described by Anthony and Fultz.[19] The effect of Ti on D03 order was also studied in detail in References 20 and 21. Very recently, the Fe3Al iron aluminides substantially alloyed with Ti (generating coherent A2 + L21 microstructure) were used for near-net-shape processing technologies.[22]
4 Conclusion
The main task of describing the rules of the cooperative hardening of Fe3Al by two solutes can be divided into two parts:
-
1.
It is obvious that for dilute alloys, the addition of hardening by individual solutes describes the real properties of the alloys. This can be seen in the data presented in Table V for the first two and last three alloys—the addition of σ 0.2 exp and Δσ 0.2 exp.
-
2.
For a further increase of concentration of both solutes, the strength σ 0.2 exp of the alloys is greater than the sum of σ 0.2 exp and Δσ 0.2 exp. This increase is due to the structure of the tested alloys at 1073 K (800 °C), i.e., to the D03 (L21) order of alloys Fe3Al-4Ti-4V, Fe3Al-8Ti-8V, and Fe3Al-10V-10Cr.
In further research, the iron aluminide Fe3Al will be used for studies of a series of multicomponent alloys. These studies will aim to determine how far the good anticorrosion properties of iron aluminides can be maintained in Fe3Al containing high concentrations of two or more solutes.
References
Hardwick and G. Wallwork: Rev. High-Temp. Mater., 1978, vol. 4, pp. 47–74.
R.Prescott, M.J. Graham: Oxid. Met., 1992, vol. 38, pp. 73–87
P.F. Tortorelli, J.H. De Van: Mater. Sci. Eng. A, 1992, vol. 153, pp. 573–77
N.S. Stollof: Mater. Sci. Eng. A, 1998, vol. 258, pp. 1–14
V.K. Sikka, D. Wilkening, J. Libertau, B. Mackey: Mater. Sci. Eng. A, 1998, vol. 258, pp. 229–35
C.G. McKamey, in Physical Metallurgy and Processing of Intermetallic Compounds, N.S. Stoloff and V.K. Sikka, eds., Chapman & Hall, New York, 1996, p. 351.
D.G. Morris and M.A. Munoz-Morris: Adv. Eng. Mater., 2011, vol. 13(1-2), pp. 43–47.
M. Palm: Intermetallics, 2005, vol. 13(12), pp. 1286–95.
D.G. Morris: Intermetallics, 1998, vol. 6, pp. 753–58.
K. Vedula, in Intermetallic Compounds, Practice, J.H. Westbrook and R.L. Fleischer, eds., Wiley, Chichester, 1995, Vol. 2, p. 199
[11] M.G. Mendiratta, Mater. Res. Soc. Symp. Proc., 1987, vol. 81, pp. 393–404.
P. Kratochvil, J. Pešička, R. Král, M. Švec, M. Palm: Metall. Mater. Trans. A, 2015, vol. 46A (11), pp. 5091–94
R.L. Fleischer, Acta Metall., 1963, vol.11, pp. 203–09
R. Labusch: Phys. Status Solidi (B), 1970, vol. 41b, pp. 659–69.
D.G. Morris and M.A. Munoz-Morris: Intermetallics, 2005, vol. 13, pp. 1269–74.
G. Hasemann, J.H. Schneibel, and E.P. George: Intermetallics, 2012, vol. 21, pp. 56–61.
F.Stein, A. Schneider, G. Frommeyer: Intermetallics 2003, vol. 11, pp. 71–82.
F.Stein, MPIE Stuttgart, Germany, unpublished research, 2017.
L.Anthony, B. Fultz: Acta Metall. Mater. 1995, vol. 43, pp. 3885–91.
M. Palm, G. Inden, N. Thomas: J.Phase Equilib., 1995, vol. 16, pp. 209–22.
I. Ohnuma, C.G. Schön, R. Kainuma, G. Inden, K. Ishida: Acta Mater. 1998, vol.46, pp. 2083–93.
A. Michalcová, L. Senčeková, G. Rolink, A. Weisheit, J. Pešička, M. Stobik, M. Palm: Mater. Des., 2017, vol. 116, pp. 481–94.
Acknowledgments
Most of the alloys used in this work were kindly prepared by Frank Stein and Johannes Deges of the MPI für Eisenforschung in Düsseldorf, Germany. This help is acknowledged. The research is a part of the Project 16-05608S of the Czech Research Foundation.
Author information
Authors and Affiliations
Corresponding author
Additional information
Manuscript submitted June 3, 2017.
Rights and permissions
About this article
Cite this article
Kratochvíl, P., Daniš, S., Minárik, P. et al. Strengthening of Fe3Al Aluminides by One or Two Solute Elements. Metall Mater Trans A 48, 4135–4139 (2017). https://doi.org/10.1007/s11661-017-4211-x
Received:
Published:
Issue Date:
DOI: https://doi.org/10.1007/s11661-017-4211-x