Abstract
Saposhnikoviae Radix (SR) is a commonly used crude drug that is obtained from the root and rhizome of Saposhnikovia divaricata which is distributed throughout China, Korea, Mongolia, and Russia. To evaluate the quality of Mongolian S. divaricata, metabolomic profiling of 43 plant specimens from different regions of Mongolia, as well as 8 SR samples and 2 plant specimens from China, were conducted by liquid chromatography–ion-trap–time-of-flight–mass spectrometer (LC–IT–TOF–MS). LC–MS profiles of the specimens showed uniformity and 30 compounds were tentatively identified, including 13 chromones and 17 coumarins. Among them, 16 compounds were isolated and unambiguously verified by comparing them with the spectroscopic data of standard compounds. Orthogonal partial least squares–discriminant analysis (OPLS–DA) based on LC–MS data from 7 Mongolian specimens and 8 Chinese SR samples as well as 2 plant specimens revealed that these 2 groups were clearly distinguishable and that Mongolian specimens were characterized by an abundance of prim-O-glucosylcimifugin (1). Moreover, the OPLS–DA of the Mongolian specimens showed that they can be discriminated by their growing regions based on the content of 8 chromones. The total content of dihydrofurochromones 1–3 was relatively higher in the specimens from Khalkhgol in the far eastern part of Mongolia, while contents of 10, 11, 15, and 16 were higher in those from Holonbuir in the eastern part. Based on this research, the roots of S. divaricata from Mongolia have potential as a new resource of SR in Kampo medicine.
Similar content being viewed by others
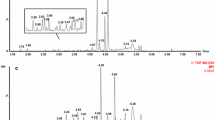
Explore related subjects
Discover the latest articles, news and stories from top researchers in related subjects.Avoid common mistakes on your manuscript.
Introduction
Mongolia is located in the hinterland of the eastern part of central Asia and surrounded by China and Russia. There is a vast floral resource which conforms to its extreme continental climate characterized by long, cold winters and short summers. Around 3000 species of vascular plants are distributed in the different geographical regions: 812 species are efficacious medicinal plants, and 200 species have been used as formulaic ingredients in Mongolian traditional medicine [1]. Mongolian medicinal plants are also attractive as sources of crude drugs used in traditional Chinese medicine as well as in Japanese traditional medicine (Kampo); for example, Glycyrrhiza uralensis Fischer is the source of Glycyrrhizae Radix; Ephedra sinica Stapf. is that of Ephedra Herba; and Astragalus mongholicus Bunge is that of Astragali Radix. Assessment of the qualities of these plants, previously conducted by our group, suggest regional variations [2-5]. Having addressed these species, we then turned out attention to Saposhnikovia divaricata (Turcz.) Schischk. of the family Umbelliferae (Apiaceae), because its root and rhizome, known as Saposhnikoviae Radix (SR), have been frequently used as ingredients of Kampo formulae, such as "Bofutsushosan" in Japan ("Fang-feng-tong-sheng-san" in China) for the treatment of obesity and metabolic syndrome [6]. In China, Japan, and Korea, SR has been widely applied for the treatment of pyrexia, rheumatism, headache, vertigo, generalized aching, and arthralgia in traditional medicine [7].
Previous phytochemical studies have revealed that the roots of S. divaricata contain chromones, coumarins, polyacetylenes, and polysaccharides, among which chromones have the most pharmacological relevance, including analgesic, anti-oxidant, anti-inflammatory, anti-proliferative, anti-allergic, and anti-cancer effects [7]. Chromones, the major compounds in SR, are classified into linear dihydrofurochromones and linear dihydropyranochromones. Two dihydrofurochromones, namely prim-O-glucosylcimifugin (1) and 4′-O-β-d-glucosyl-5-O-methylvisamminol (3), which have been reported to exhibit anti-inflammatory effects [8, 9], have been selected as marker compounds for SR quality control in the Chinese Pharmacopoeia (CP) [10], and the latter is used in the Japanese Pharmacopoeia (JP) [11]. Coumarins are abundant in SR, they are composed of linear dihydrofurocoumarins such as: deltoin which has anti-inflammatory activity [12]; linear furocoumarins, including psoralen, xanthatoxin, bergapten, and phellopterin, which have been reported to exhibit analgesic, anti-proliferative, and anti-convulsant effects [13-15]; khellactone-type coumarins, such as praeruptorin B (anomalin), with anti-inflammatory activity [16]; and simple coumarins, such as scopoletin, with anti-inflammatory activity [17].
In Mongolia, the root and rhizome of S. divaricata have not been used as medicines, but the aerial parts called "Derveger Jirgeruu" has been used for the treatment of stomach disorders for thousands of years [18]; therefore, the utilization of Derveger Jirgeruu have not disturbed the land by maintaining permafrost. In recent years, however, due to an increased demand for SR and its decreased production in China, Mongolian S. divaricata has been illegally collected by local inhabitants for export to their neighboring country. To sustain SR resources, cultivation is more efficient than the collection of naturally growing plants; however, to date, large scale cultivation has not been practiced in Mongolia.
Currently, a large amount of SR cultivated in China has become available in the Chinese and Japanese markets [19]. Although SR derived from cultivated S. divaricata should possess the same efficacy as SR from wild plants, it has been reported that the contents of main chromones in SR from the cultivated plants are lower than those from wild plants, while the yield of the extract in the former is twice as high as in the latter [20]. Therefore, sustainable utilization of SR resources should be implemented through high-performance cultivation by selecting suitable plant resources and cultivation areas as well as through the development of methods for cultivation, processing, etc. Mongolia was chosen for this study because of the large population of wild growing S. divaricata, especially in the eastern part and because of the attention paid by the local government to the efficient usage of medicinal plants. Moreover, no chemical studies of Mongolian S. divaricata have yet been undertaken. Thus, to identify sources of SR with superior genetic properties and areas suitable for the cultivation of S. divaricata, a field investigation was performed in Mongolia. A quality evaluation of S. divaricata roots from several regions was conducted by means of metabolomic profiling based on the liquid chromatography–ion-trap–time-of-flight–mass spectrometer (LC–IT–TOF–MS) method combined with multivariate statistical analysis.
Materials and methods
Plant specimens and crude drug samples
Forty-three plant specimens of S. divaricata were collected from eastern part of Mongolia, including Khentii and Dornod provinces (prov.) during the field investigation from 2015 to 2017 (Fig. 1a; Table 1A). Eight batches of SR samples and 2 plant specimens were obtained from China (Table 1B). All plant specimens were authenticated by Prof. Katsuko Komatsu (Institute of Natural Medicines, University of Toyama, Japan), and voucher specimens were deposited at the Museum of Materia Medica, Institute of Natural Medicine, University of Toyama, Japan (TMPW).
Information on plant specimens from Mongolia. a Collection location of plant specimens: the dotted line shows the route of the field investigation in 2017. b Shape of underground parts: (1) 2 newly formed roots on the old root; (2) a long root 75 cm in length; (3) 2 types of roots from non-flowering (M38) and flowering (M39) plants; c methods for division: (i) roots not greater than 30 cm in length were divided into two halves and were labeled upper and lower parts; (ii) roots greater than 30 cm in length were divided approximately every 10 cm and were labeled A, B, C, etc. from the base; (iii) 2 or more newly formed roots on the old root were labeled B1, B2, B3, etc.; (iv) roots branched at the lower part, 2 rootlets labeled R1 and R2, and upper part of the root were selected
The underground parts of plant specimens varied in shape and size depending on their growing conditions. To clarify the chemical variations concerned with aspects such as growing region, flowering or not, and root parts, all specimens as well as SR samples were divided into the following categories:
- 1.
Flowering or non-flowering variations: all specimens were divided into 2 groups, 17 flowering specimens and 17 non-flowering specimens, as well as 9 specimens without aerial parts (Fig. 1b).
- 2.
Root parts: as the shape and the length of roots were varied, the roots of all specimens and SR samples were cut into portions according to the following rules (Fig. 1c):
- (i)
Roots not greater than 30 cm in length: the root was divided into two halves and the parts were labeled upper and lower parts. Sometimes the root was divided into three equal lengths and the parts were labeled upper, middle, and lower parts. Half of each part was used for the preparation of LC–MS samples.
- (ii)
Roots greater than 30 cm in length: the root was divided approximately every 10 cm starting from the base, and the parts were labeled A, B, C, etc. Half of each part was used for the preparation of LC–MS samples.
- (iii)
2 or more new roots formed on the old root: new roots were separated from the old root and were labeled B1, B2, B3, etc. Half of each part was used for the preparation of LC–MS samples.
- (iv)
Roots that branch at the lower part: 2 rootlets labeled R1 and R2, and upper part of the root were selected. Whole part of the rootlets and upper part were used for the preparation of LC–MS samples.
- 3.
Regional variations: 6 different locations from central to eastern part of Mongolia, including 2 locations in Khentii prov. and 4 in Dornod prov. (Table 1A).
According to the above classification method, 131 individual samples were obtained from the roots of 43 Mongolian plant specimens, and 19 individual samples were obtained from 2 Chinese plant specimens and 8 batches of SR samples (3 batches composed of cut pieces were counted as 3 individual samples).
Chemicals and reagents
The following 16 reference compounds were isolated and purified from the SR sample C9 (see supplementary materials): prim-O-glucosylcimifugin (1) [21], cimifugin (2) [21], 4′-O-β-d-glucosyl-5-O-methylvisamminol (3) [21], 5-O-methylvisamminol (4) [21], isopimpinellin (5) [22], sec-O-glucosylhamaudol (6) [21], psoralen (7) [22], xanthatoxin (8) [22], bergapten (9) [22], hamaudol (10) [21], 3′-O-acetylhamaudol (11) [21], ledebouriellol (12) [21], phellopterin (13) [23], deltoin (14) [21], 3′-O-angeloylhamaudol (15) [21], and praeruptorin B (16) [24]. They were identified by spectroscopic methods as well as by comparison of their MS and 1H NMR data with values reported in the literatures (Fig. 2).
LC–MS grade acetonitrile, methanol, and formic acid were purchased from Fujifilm Wako Pure Chemical (Osaka, Japan). Pure water for extraction and LC–MS analysis was prepared with a Milli-Q system (Millipore, MA, USA). All other chemicals were analytical grade.
HPLC–IT–TOF–MS conditions
The HPLC analysis was performed with an HPLC system (Shimadzu, Tokyo, Japan) equipped with an SCL-10AVP system controller, LC-20AD binary pump, a DGU-20A degasser, an SIL-20AC auto-sampler, a CTO-20AC column oven, and an SPD-M20A photo-diode-array (PDA) detector. Chromatographic separations were carried out on an Atlantis T3 column (3 μm, 2.1 × 150 mm, Waters, MA, USA) at a column temperature of 30 °C. The mobile phase consisted of water containing 0.1% formic acid (v/v) (solvent A) and acetonitrile containing 0.1% formic acid (v/v) (solvent B) with elution by a linear gradient at a flow rate of 0.2 mL/min. For the first quality assessment comparing plant specimens from Mongolia with Chinese plant specimens and SR samples, a simple gradient elution system (I)—consisting of 0–4 min at 20% B, 4–69 min at 20–80% B, and 69–70 min at 80–100% B—was established. For qualitative comparisons of plant specimens from different regions of Mongolia, a different gradient elution system (II) of 0–5 min at 20% B, 5–15 min at 20–25% B, 15–30 min at 25% B, 30–40 min at 25–40% B, 40–90 min at 40–80% B, and 90–91 min at 80–100% B was established. Detection was by UV absorption at wavelengths of 200–400 nm, and the injection volume was 5 μL.
For MS analysis, a Shimadzu ion-trap–time-of-flight–mass spectrometer (IT–TOF–MS) equipped with an electrospray ionization interface was connected to the Shimadzu LC-20A HPLC system. A standard solution of trifluoroacetic acid sodium (TFA) was used to calibrate the TOF–MS to increase mass accuracy. The conditions of the ionization interface positive and negative were: source voltage, + 4.5 kV (for positive ionization modes) or − 3.5 kV (for negative ionization modes); 1.5 L/min nebulizer gas flow; 100 kPa dry gas pressure; 200 °C curved desolvation line (CDL) and heat block temperature. Molecular weight acquisition was performed from m/z 100–2000 for MS and MS/MS. The ion accumulation time was set at 30 ms, and the collision energy of collision-induced dissociation (CID) was set at 50%. The LCMS solution version 3.81 software package (Shimadzu, Tokyo, Japan) was used for data acquisition and processing.
Sample preparation
Plant specimens and SR samples were pulverized into fine powders of about 70 mesh, and 100 mg of each sample was extracted with 20 mL of 70% MeOH in an ultrasonic bath at room temperature for 30 min, and then centrifuged at 4000 rpm for 5 min. The supernatant solution was diluted with ultrapure water to 1:1 (v/v), and the resultant solution was filtered through a 0.2 μm membrane filter prior to use.
Method validation
Intra- and inter-day precisions were determined to evaluate the repeatability, stability, and reproducibility obtained with the gradient elution system II. Representative sample solutions of SR sample (C9) and upper and lower parts of plant specimen (M1) were prepared and injected in triplicate within a day and on 3 consecutive days, for intra- and inter-day precision assessments, respectively. The relative standard deviation (RSD) of the peak area was taken as the indicator for method validation.
Statistical and multivariate statistical analyses
The profiling solution (Shimadzu, Tokyo, Japan) was used to convert all raw data (tR 3–60 min for gradient system I or tR 3–85 min for gradient system II) from LC–MS profiles of plant specimens and SR samples into the data matrix for subsequent import to Microsoft Excel. Then, the data matrix was imported to SIMCA 14.0 (Umetrics, Umea, Sweden) for mean centering and Pareto scaling before multivariate analysis. Principal component analysis (PCA) and orthogonal partial least squares–discriminant analysis (OPLS–DA) were carried out.
Data of peak areas were expressed as means and differences between 2 independent groups were analyzed by means of the Student's t test. A value of p < 0.05 was regarded as statistically significant.
Results and discussion
Optimization of extraction and chromatographic condition
Several parameters, such as extraction solvent, extraction duration, and the amount of solvent in the sample preparation procedure, were considered on the basis of the previous study [20]. First, the extraction solvent was investigated with MeOH vs. 70% MeOH, with finding that 70% MeOH extraction provided yields 1.5–2.5% higher than the MeOH, in good agreement with the previous study [20]. Next, ultrasound-assisted extraction was chosen because of its convenience, as in the previous study [20]. Ultrasound-assisted extraction durations of 30 and 60 min were compared: ultrasonication in a water bath for 60 min did not exhibit greater extraction efficiency than 30 min, hence, ultrasonic extractions of 30 min were used to save time. Since yields from extraction performed with 20 vs. 25 mL of solvents were not significantly different, the lower volume was selected. On the basis of the above results, the following sample preparation method was established: 100 mg pulverized root was extracted with 20 mL of 70% MeOH in an ultrasonic bath at room temperature for 30 min. The yields of 70% MeOH extract of Mongolian plant specimen M1 and wild type of SR sample C9 were 18.2% and 23.8%, respectively, while those of cultivated type of SR samples C4 and C10 were 53.7% and 59.5%, respectively. The yields of 70% MeOH extracts of cultivated SR were more than twice as high as those of wild SR, agreeing well with the reported result [20].
Three analytical parameters, including gradient system, mobile phase modifier, and column oven temperature, were optimized to achieve good peak resolutions and desirable separations. Initially, the gradient system was optimized from the simple gradient elution method I to establish the combined isocratic and gradient method II. Peak of 6 was overlapped with a minor peak during linear gradient elution from 20–80% of solvent B; these overlapping peaks could be effectively separated by insertion of the isocratic 25% B step at 15–30 min in gradient system II, at the cost of an extra 27 min analysis. Next, the mobile phase modifiers formic acid vs. acetic acid were considered: 0.1% formic acid was selected, because it enhanced the intensity of adducted molecular ions [M+HCOO]− and protonated molecular ions [M+H]+ in the MS. Finally, the column temperature was optimized to achieve a desirable separation of each peak: 4 oven temperature, 25 °C, 30 °C, 35 °C, and 40 °C, and temperature of 30 °C was found to effectively improve the separation of peak of 6 and that of 14 from minor peaks (Fig. S1).
Method validation
A qualitative analysis method based on considerations of repeatability, stability, and reproducibility for LC–MS profiling of SR was validated. The validation was performed on representative samples (C9, M1) based on retention time and peak areas obtained with gradient elution system II. Intra- and inter-day precisions were determined by analyzing 3 replicates in one day and 3 consecutive days, respectively. The RSD values of intra- and inter-day analyses were 0.4–6.8% and 0.7–8.6%, respectively (Table S1). This result indicated that the precision was sufficient to proceed with multivariate statistical analysis.
Characterization and identification of compounds in SR
The SR sample C9 from China was used for LC–MS profiling to identify metabolites. Chopped roots (1450 g) were extracted 3 times with methanol (MeOH) at room temperature for 24 h to yield 360 g of MeOH extract. Then, a portion of MeOH extract (140 g) was suspended in water and subsequently partitioned with ethyl acetate (EtOAc) and n-butanol (1-BuOH) to give the EtOAc layer, 1-BuOH layer, and water residue with yields of 26.3, 11.3, and 97.1 g, respectively. The EtOAc layer contained more abundant and numerous constituents than the other fractions, whereas the n-BuOH layer contained abundant amounts of the major chromones 1 and 3 (Fig. S2). Therefore, we separated the EtOAc and n-BuOH layers by means of chromatography and successfully isolated 20 compounds (see supplementary materials). Thirty compounds from the EtOAc layer, including 13 chromones and 17 coumarins were characterized and tentatively identified by comparing precursor ion values, product ion values, and UV spectra with related literature findings [25-27] (Fig. 3; Table 2). Their chemical structures are shown in Fig. 2. Among them, 16 compounds were unambiguously identified by comparing LC–MS/MS and UV data of reference standard compounds (Table 2).
Total ion chromatogram (TIC) of SR sample in positive (upper) and negative ionization (lower) modes. The EtOAc layer of the MeOH extract (SR sample C9) was dissolved in 50% MeOH. The details of identified compounds (peaks P1–P30, 1–16) are summarized in Table 2. PB the peak also detected in the blank sample (only solvent)
Comparison of metabolomic profile between Mongolian S. divaricata roots and Chinese SR
For a comparison between Mongolian and Chinese S. divaricata roots, representative 1 or 2 plant specimens from 5 different locations of Mongolia were selected and analyzed together with 8 SR samples as well as 2 plant specimens from China by LC–MS with the gradient elution system I. Total ion chromatograms (TIC) of representative samples are shown in Fig. 4. All plant specimens and SR samples exhibited similarity in the composition of peaks in LC–MS profiles to each other. Sixteen compounds were identified in both MeOH extracts of Mongolian and Chinese S. divaricata roots (Table 2). When touching upon peak areas of each compound, some differences were shown between the wild and cultivated types of Chinese SR samples. The wild type of SR contained relatively larger amounts of constituents than the cultivated type. To compare the contents of main dihydrofurochromones 1–4 and dihydropyranochromones 6 and 15 in the Mongolian and Chinese S. divaricata, the peak areas in the extracted ion chromatogram (EIC) data of divided root parts for each specimen were averaged (Fig. 5). The peak area of 3 in Mongolian specimens was similar to those of Chinese specimens and SR samples (Fig. 5a). However, the peak areas of 1 and 15 were significantly higher in Mongolian plant specimens than in Chinese specimens and SR samples (p < 0.01 and p < 0.05, respectively; Fig. 5; Table S2). Obviously, the peak areas of 2 and 4 in Chinese SR samples, C3 and C5, were higher than others (Figs. 4, 5a). Subsequently, to clarify the differences between them and to identify the primary contributors, multivariate statistical analysis, PCA and OPLS–DA were carried out for LC–MS data of all specimens and SR samples. The PCA results were similar to those of OPLS–DA, and absolute separation into two main groups, Chinese and Mongolian, was achieved for the 89 observations. The overall goodness of fit (R2Y = 0.829) and overall cross validation coefficient (Q2Y = 0.754) demonstrated that the predictability of the model was good (Fig. 6a). As shown in Fig. 6b, the loading scatter plot of OPLS–DA indicated 2 and 4 as potential contributors to the Chinese group. On the other hand, 1, 3, 15, and an unidentified compound with m/z 525 (X) were contributed most to the Mongolian group. The isolation and identification of compound X were carried out, and it was speculated as a derivative of 6 based on the LC–MS/MS analysis (Fig. S3). The structure determination of compound X is now in progress. Recently, Yoshitomi et al. reported that the wild type of SR could be discriminated by glucose and oleic acid from the cultivated type of SR which were characterized by sucrose using 1H NMR-based metabolomics approach [28]. Since we focused on the detection of the compounds listed in Table 2 in our LC–MS condition, these compounds were not considered. Although many previous studies have been reported on the Chinese SR [19, 20, 25, 28, 29], there are very few regarding Mongolian S. divaricata. To clarify regional variation in chemical component of Mongolian S. divaricata in more detail, we examined 36 additional Mongolian specimens in flowering or non-flowering collected from different regions.
TIC of MeOH extracts of representative SR samples and plant specimens from China in positive (upper) and negative (lower) ionization modes. MeOH extracts of upper parts of the roots were analyzed with gradient elution system I. a, b Plant specimens C1 and C2, respectively; c–f SR samples C4, C5, C7, and C9, respectively. Numerals indicate respective compounds in Fig. 2
The peak areas of main chromones a1–4 and b6 and 15 in 8 SR samples as well as 2 plant specimens from China and 7 flowering specimens from Mongolia. The peak areas are shown as the means of the divided root parts in extracted ion chromatogram (EIC) that analyzed with gradient elution system I (n = 3; *n = 1). W wild type of SR, C cultivated type of SR. Numerals indicate respective compounds in Fig. 2
Orthogonal partial least squares–discriminant analysis (OPLS–DA) for the SR samples and plant specimens from China and specimens from Mongolia. a Score scatter plot and b loading scatter plot. The LC–MS data set was same with Fig. 5. Numerals indicate respective compounds in Fig. 2. 1′ and 3′ indicate isotopic ion [M+H+1]+ of the respective compounds
1 TIC of 70% MeOH extracts of (u) upper (m) middle, and (l) lower parts of the root of representative plant specimen (M3) from Bayan-Ovoo, Khentii prov. in positive (upper) and negative (lower) ionization modes. Numerals indicate respective compounds in Fig. 2. 2 TIC of 70% MeOH extracts of upper parts of the roots of representative plant specimens from each region of Mongolia in positive (upper) and negative (lower) ionization modes. (a) M1 from Khalkhgol, Dornod prov.; (b) M6 from Holonbuir, Dornod prov.; (c) M9 from Bulgan, Dornod prov.; (d) M41 from Tamsagbulag, Dornod prov.; (e) M43 from Ondorkhaan, Khentii prov. Numerals indicate respective compounds in Fig. 2.
Metabolomic profiling of S. divaricata roots from Mongolia
For characterizing and comparing the chemical constituents in the S. divaricata roots from different regions of Mongolia, 70% MeOH extracts of 43 plant specimens were prepared by the aforementioned method and were chromatographically analyzed with gradient elution system II. LC–MS profiles of Mongolian specimens showed similarity in the composition of peaks, as well as those of upper, middle, and lower parts of the root (Fig. 7-1u, 1m, and 1l). Twelve common peaks (1–4, 6, X, 10–12, 14–16) were observed in the LC–MS chromatograms of all specimens except for 3 specimens from the Tamsagbulag (M39–M41), Dornod prov., which had the peak of an unidentified compound with m/z 358 at tR 53 min (Fig. 7-2d). Furthermore, the peak of compound X was frequently observed at tR 39 min in the specimens from Mongolia (Fig. 7-2a–2e). Peaks of 11, 12, 15, and 16 were higher in the specimen from Holonbuir, Dornod prov. (Fig. 7-2b). When comparing the peak areas between LC–MS profiles of root parts within one root, a larger amount of 3 was observed in the lower parts of the roots, while compound X tended to be higher in the upper parts of the roots (Figs. 7-1 and S4). In Fig. 8, the peak areas in EIC of main dihydrofurochromones 1–4 and dihydropyranochromones 6 and 15, which were shown as the means of the divided root parts, were compared to reveal that the peak area of each chromone was extremely variable and dependent on not only the growing region but also on whether flowering or not. Mongolian specimens generally contained a large amount of 1. The peak area of 15 was markedly higher in the specimens from Holonbuir, and those of 1 and 3 were tended to be higher in the specimens from Khalkhgol. While, the peak areas of 2 and 4 varied among all specimens, and this variation might be attributable to the growing stage rather than the growing region.
The peak areas of main chromones a1–4 and b6 and 15 in all plant specimens except for specimens obtained from local government and collector (H, Y, K, C) from different regions of Mongolia. The peak areas are shown as the means of the divided root parts in EIC that analyzed with gradient elution system II. F flowering plant specimens, NF non-flowering plant specimens. Numerals indicate respective compounds in Fig. 2
To date, no comparison has demonstrated that the root part affects the chemical profile in SR. To clarify whether differences in the part of the root affected the chemical profile, multivariate statistical analysis based on LC–MS data was performed. First, to determine variations between the root parts of plant specimens, OPLS–DA was carried out for LC–MS data of 40 specimens of upper root parts and 31 specimens of lower root parts (R2Y = 0.676, Q2Y = 0.533). The 2 parts of the roots tended to separate into 2 groups (Fig. 9a): the S-plot of OPLS–DA revealed that 3 had more influence on discrimination of the lower parts of the roots, while 12, 15, and compound X were likely to have more contribution for separation of the upper parts of the roots (Fig. 9b). This result was strongly consistent with the comparisons of the LC–MS profiles of different parts of the roots, where a higher amount of 3 was observed in the lower parts, while compound X was higher in the upper parts (Fig. S4).
OPLS–DA for elucidating the difference among root parts (a score scatter plot, b S-plot) and that between flowering or non-flowering specimens (c score scatter plot, d S-plot). Numerals indicate respective compounds in Fig. 2. 1″ and 3″ indicate [M+HCOO]− of the respective compounds
Next, to investigate the differences between flowering and non-flowering specimens, 8 flowering specimens and 11 non-flowering specimens both growing in grassland were evaluated by OPLS–DA. As shown in Fig. 9c, the loading scatter plot of the OPLS–DA for the 2 groups showed good separation. The values of R2Y and Q2Y were calculated to be 0.983 and 0.763, respectively, indicating satisfactory predictability. Interestingly, the S-plot of the OPLS–DA indicated that 1 discriminated the specimens that were flowering, and the unidentified compound with m/z 358 was frequently found in non-flowering specimens (Fig. 9d). The similar result was obtained when all the specimens including those from cropland were applied to the OPLS–DA. However, as shown in Fig. 8a, markedly higher amount of 1 were observed in several non-bolting and non-flowering specimens from Khalkhgol (M29, M34, M36, M40). Since these specimens had single thick root or newly formed roots on the old root, they may have grown more than 2 years. Thus, it was indicated that whether the flowering or non-flowering at the mature stage has no relevance to the contents of constituents. In case of Mongolian wild plant, the aerial parts of the plant freeze and fall in the winter season and then, new roots grow on the old root in next spring. Probably in the first year, the plant will not be bolting (no flower), but the root becomes mature. For this reason, the contents of the constituents in the root can increase even in non-flowering plant. Our findings showed that one of the most important factors that influence the quality of SR might be the number of growing years. A detailed study on variation of chemical components associated with the growing year as well as season will be necessary.
Finally, to highlight the differences among growing regions of Mongolian specimens and to identify discriminatory markers, OPLS–DA was carried out for LC–MS data of 31 specimens collected in 2017 which have single or straight root. Most of the specimens from cropland (except M32) and 3 specimens from grassland (M24, M39, M41) that have new roots on the old root or branched roots were excluded. The score scatter plot of the OPLS–DA indicated that all specimens were characterized by their growing regions (R2Y = 0.821, Q2Y = 0.536, Fig. 10a) and the loading scatter plot of the OPLS–DA indicated that 1–3 were most relevant to the specimens from far eastern part of Mongolia, Khalkhgol and Tamsagbulag; 10, 11, 15, and 16 were most abundant in the specimens from the eastern part, Holonbuir; 10 was recognized as a marker compound for the specimens from Bulgan; compound X may be used as a discriminatory marker for the specimens from central eastern part of Mongolia, particularly Ondorkhaan and Bayan-Ovoo (Fig. 10). Since the specimens from Holonbuir and Bulgan were characterized by 10, 11, 15, and 16 besides compound X, they were discriminated from the specimens of Ondorkhaan and Bayan-Ovoo. The result of OPLS–DA was consistent with that of LC–MS profiles, where the specimens from Holonbuir contained 10–12, 15, and 16 abundantly (Fig. 7-2b); the specimen from Bulgan contained a relatively high amount of 10 (Fig. 7-2c); the specimens from Ondorkhaan, Bayan-Ovoo, and Holonbuir contained compound X markedly higher than others (Fig. 7-2e, 1u, 2b). Moreover, the specimens from Khalkhgol and Tamsagbulag contained high amounts of major chromones 1–3 (Fig. 7-2a, 2d). However, the variation among the specimens was observed, especially some specimens (M25, M31) collected in the cropland showed lower contents (Fig. 8a). The difference in the shape and hardness of the root were observed between specimens from cropland and those from grassland. The specimen from grassland had hard, straight, and single root, by contrast, the specimen from cropland had soft and curved root with newly formed roots on the top. In particular, the specimen M31 which contained less amount of chromones had more than 10 newly formed thin roots on the old root. Therefore, the specimens from grassland were thought to be suitable for the crude drug SR to avoid SR with low content of main chromones. From this viewpoint, the high-performance cultivation method including grassland cultivation in suitable areas, followed by the best harvest season determined on the basis of the experiment, sometimes combined with wild plant collection, should be developed.
OPLS–DA for the specimens from different regions of Mongolia. a Score scatter plot and b loading scatter plot. Numerals indicate respective compounds in Fig. 2. 1″ indicates [M+HCOO]− of 1
The present study evaluated S. divaricata from Mongolia through qualitative analysis. To verify this result, quantitative determination of characteristic compounds is in progress.
Conclusions
In the present study, we evaluated S. divaricata obtained from different regions of Mongolia by LC–IT–TOF–MS/MS profiling combined with multivariate statistical analysis. Forty-three plant specimens from Mongolia together with 8 SR samples and 2 plant specimens from China were investigated and characterized by the differences of growing region, root parts, and flowering or not. Among 30 compounds tentatively identified by LC–MS/MS, 8 chromones were considered to be discriminatory compounds for group separation in Mongolia. The results revealed that dihydrofurochromones 1–3 were most contributed to the specimens from Khalkhgol and Tamsagbulag; 10, 11, 15, and 16 were most relevant to the specimens from Holonbuir; and the compound X with m/z 525 speculated as a derivative of 6 was the discriminatory marker for the separation of specimens from central eastern part, particularly Ondorkhaan and Bayan-Ovoo. Quality evaluation of S. divaricata roots from different regions of Mongolia was investigated for the first time. Based on this research, S. divaricata roots from Mongolia have potential as new resources of SR used in Kampo formulae, particularly those from far eastern part of Mongolia were superior in the contents of the major chromones 1–3. Natural resources of S. divaricata have been greatly reduced due to recent illegal harvesting. Therefore, it is necessary to promote cultivation in suitable regions to secure resources of SR. In this viewpoint, Khalkhgol, far eastern part of Mongolia, could be a prospective region for the cultivation of S. divaricata.
References
Jamyandorj J, Ligaa U, Otgonbileg K, Saaral N (2011) Very rare, rare and important useful plants, cultivating in Khuduu-Aral of Kherlen. Ulaanbaatar Print, Mongolia, pp 242–243
Kitani Y, Zhu S, Omote T, Tanaka K, Batkhuu J, Sanchir C, Fushimi H, Mikage M, Komatsu K (2009) Molecular analysis and chemical evaluation of Ephedra plants in Mongolia. Biol Pharm Bull 32(7):1235–1243. https://doi.org/10.1248/bpb.32.1235
Kitani Y, Zhu S, Batkhuu J, Sanchir C, Komatsu K (2011) Genetic diversity of ephedra plants in Mongolia inferred from internal transcribed spacer sequence of nuclear ribosomal DNA. Biol Pharm Bull 34(5):717–726. https://doi.org/10.1248/bpb.34.717
Zhu S, Sugiyama R, Batkhuu J, Sanchir C, Zou K, Komatsu K (2009) Survey of Glycyrrhizae Radix resources in Mongolia: chemical assessment of the underground part of Glycyrrhiza uralensis and comparison with Chinese Glycyrrhizae Radix. J Nat Med 63(2):137–146. https://doi.org/10.1007/s11418-008-0303-7
Tanaka K, Tamura T, Fukuda S, Batkhuu J, Sanchir C, Komatsu K (2008) Quality evaluation of Astragali Radix using a multivariate statistical approach. Phytochemistry 69(10):2081–2087. https://doi.org/10.1016/j.phytochem.2008.04.016
Gao SL, Satsu H, Makino T (2018) Inhibitory effect of bofutsushosan (fang feng tong sheng san) on glucose transporter 5 function in vitro. J Nat Med 72(2):530–536. https://doi.org/10.1007/s11418-018-1183-0
Kreiner J, Pang E, Lenon GB, Yang AWH (2017) Saposhnikoviae divaricata: a phytochemical, pharmacological, and pharmacokinetic review. Chin J Nat Med 15:255–264
Chen N, Wu Q, Chi G, Soromou LW, Hou J, Deng Y, Feng H (2013) Prim-O-glucosylcimifugin attenuates lipopolysaccharide-induced acute lung injury in mice. Intern Immunopharmacology 16(2):139–147. https://doi.org/10.1016/j.intimp.2013.04.014
Chang CZ, Wu SC, Kwan AL, Lin CL (2015) 4'-O-beta-d-glucosyl-5-O-methylvisamminol, an active ingredient of Saposhnikoviadivaricata, attenuates high-mobility group box 1 and subarachnoid hemorrhage-induced vasospasm in a rat model. Behav Brain Funct 11(1):28. https://doi.org/10.1186/s12993-015-0074-8
The State Pharmacopoeia Commission of China (1997) Pharmacopoeia of People`s Republic of China, vol 1 (English Edition). The Chemical Industry Press, Beijing
The Ministry of Health, Labour, and Welfare (2016) The Japanese Pharmacopoeia, 17th edn (English version). The MHLW Ministerial Notification No. 64, Tokyo
Wang CC, Chen LG, Yang LL (1999) Inducible nitric oxide synthase inhibitor of the Chinese herb—I. Saposhnikovia divaricata (Turcz.) Schischk. Cancer Lett 145(1–2):151–157. https://doi.org/10.1016/S0304-3835(99)00248-7
Fujioka T, Furumi K, Fujii H, Okabe H, Mihashi K, Nakano Y, Mitsunaga H, Katano M, Mori M (1999) Antiproliferative constituents from umbelliferae plants. V. A new furanocoumarin and falcarindiol furanocoumarin ethers from the root of Angelica japonica. Chem Pharm Bull 47(1):96–100. https://doi.org/10.1248/cpb.47.96
Luszczki JJ, Andres-Mach M, Glensk M, Skalicka-Wozniak K (2010) Anticonvulsant effects of four linear furanocoumarins, bergapten, imperatorin, oxypeucedanin, and xanthotoxin, in the mouse maximal electroshock-induced seizure model: a comparative study. Pharmacol Rep 62(6):1231–1236. https://doi.org/10.1016/S1734-1140(10)70387-X
Okuyama E, Hasegawa T, Matsushita T, Fujimoto H, Ishibashi M, Yamazaki M, Hosokawa M, Hiraoka N, Anetai M, Masuda T, Takasugi M (1998) Analgesic components of Glehnia root (Glehnia littoralis). J Nat Med 52:491–501
Khan S, Shin EM, Choi RJ, Jung YH, Kim J, Tosun A, Kim YS (2011) Suppression of LPS-induced inflammatory and NF-kappa B responses by anomalin in RAW 264.7 macrophages. J Cell Biochem 112(8):2179–2188
Kamino T, Shimokura T, Morita Y, Tezuka Y, Nishizawa M, Tanaka K (2016) Comparative analysis of the constituents in Saposhnikoviae Radix and Glehniae Radix cum Rhizoma by monitoring inhibitory activity of nitric oxide production. J Nat Med 70(2):253–259. https://doi.org/10.1007/s11418-016-0969-1
Sanchir C, Batkhuu J, Boldsaikhan B, Komatsu K (2003) Illustrated guide of Mongolian useful plants. Ministry of Nature, Environment, and Tourism (MNET), Japan International Cooperation Agency (JICA), Ulaanbaatar, p 22
Liu Y, Li L, Xiao YQ, Yao JQ, Li PY, Chen L, Yu DR, Ma YL (2016) Rapid identification of the quality decoction pieces by partial least squares-based pattern recognition: grade classification of the decoction pieces of Saposhnikovia divaricata. Biomed Chromatogr 30(8):1240–1247. https://doi.org/10.1002/bmc.3673
Nishihara M, Nukui K, Osumi Y, Shiota H (2018) Quality evaluation of Saposhnikoviae Radix (differences between wild-type and cultivated products). Yakugaku Zasshi 138(4):571–579. https://doi.org/10.1248/yakushi.17-00208
Sasaki H, Taguchi H, Endo T, Yoshioka I (1982) The constituents of Ledebouriella seseloides Wolff. I. Structures of three new chromones. Chem Pharm Bull 30(10):3555–3562. https://doi.org/10.1248/cpb.30.3555
Sasaki H, Taguchi H, Enndo T, Yosioka I (1980) The constituents of Glehnia littoralis Fr Schmidt et Miq, structure of a new coumarin glycoside, ostenol-7-O-β-gentiobioside. Chem Pharm Bull 28:1847–1852. https://doi.org/10.1248/cpb.28.1847
Muller M, Byres M, Jaspars M, Kumarasamy Y, Middleton M, Nahar L, Shoeb M, Sarker S (2004) 2D NMR spectroscopic analyses of archangelicin from the seeds of Angelica archangelica. Acta Pharm 54:277–285
Lu M, Nicoletti M, Battinelli L, Mazzanti G (2001) Isolation of praeruptorins A and B from Peucedanum praeruptorum Dunn and their general pharmacological evaluation in comparison with extracts of the drug. Farmaco 56(5–7):417–420. https://doi.org/10.1016/S0014-827X(01)01053-9
Kang J, Sun JH, Zhou L, Ye M, Han J, Wang BR, Guo DA (2008) Characterization of compounds from the roots of Saposhnikovia divaricata by high-performance liquid chromatography coupled with electrospray ionization tandem mass spectrometry. Rapid Commun Mass Spectrom 22(12):1899–1911. https://doi.org/10.1002/rcm.3559
Li YY, Wang XX, Zhao L, Zhang H, Lv L, Zhou GC, Chai YF, Zhang GQ (2013) High-performance liquid chromatography-electrospray ionization time-of-flight mass spectrometry analysis of Radix Saposhnikoviae for metabolomic research. J Chromatogr Sci 51(2):99–106. https://doi.org/10.1093/chromsci/bms111
Chen LX, Chen XY, Su L, Jiang YY, Liu B (2018) Rapid characterisation and identification of compounds in Saposhnikoviae Radix by high-performance liquid chromatography coupled with electrospray ionisation quadrupole time-of-flight mass spectrometry. Nat Prod Res 32(8):898–901. https://doi.org/10.1080/14786419.2017.1366482
Yoshitomi T, Wakana D, Uchiyama N, Tsujimoto T, Kawano N, Yokokura T, Yamamoto Y, Fuchino H, Hakamatsuka T, Komatsu K, Kawahara N, Maruyama T (2019) 1H NMR-based metabolomic analysis coupled with reversed-phase solid-phase extraction for sample preparation of Saposhnikovia roots and related crude drugs. J Nat Med. https://doi.org/10.1007/s11418-019-01343-2
Kim MK, Yang DH, Jung M, Jung EH, Eom HY, Suh JH, Min JW, Kim U, Min H, Kim J, Han SB (2011) Simultaneous determination of chromones and coumarins in Radix Saposhnikoviae by high performance liquid chromatography with diode array and tandem mass detectors. J Chromatogr A 1218(37):6319–6330. https://doi.org/10.1016/j.chroma.2011.06.103
Acknowledgements
The authors would like to thank all the government staffs of Mongolia for allowing us the plant collection and Dr. Yumin He of College of Medical Sciences, China Three Gorges University and Dr. Shengli Wei of School of Chinese Pharmacy, Beijing University of Chinese Medicine for helping sample collection in China. This work was supported by JSPS KAKENHI Grant Numbers 15H05268, 24406005, 15K07993, and 18K06728, JSPS Core-to-Core Program, Asia-Africa Science Platforms, and by the Research on Development of New Drugs from Japan Agency for Medical Research and Development, AMED (Grant number JP17ak0101046h002).
Author information
Authors and Affiliations
Corresponding author
Ethics declarations
Conflict of interest
The authors declare that they have no conflict of interest.
Additional information
Publisher's Note
Springer Nature remains neutral with regard to jurisdictional claims in published maps and institutional affiliations.
Electronic supplementary material
Below is the link to the electronic supplementary material.
Rights and permissions
About this article
Cite this article
Batsukh, Z., Toume, K., Javzan, B. et al. Metabolomic profiling of Saposhnikoviae Radix from Mongolia by LC–IT–TOF–MS/MS and multivariate statistical analysis. J Nat Med 74, 170–188 (2020). https://doi.org/10.1007/s11418-019-01361-0
Received:
Accepted:
Published:
Issue Date:
DOI: https://doi.org/10.1007/s11418-019-01361-0