Abstract
Benthic ostracods are deemed as one of the most useful micro-fauna for biomonitoring in shallow marine ecosystem, which are sensitive to environmental changes that can be reflected by their diversity and abundances. The pollution leads to high dominance index and low abundance ostracod communities. Therefore, eighteen sediment samples were collected from Ras Gharib and Quseir sites, along the Red Sea coast, to assess the ostracods response toward the anthropogenic environmental disturbances. Jugosocythereis borchersi, Alocopocythere reticulata, and Loxocorniculum ghardaqensis are the most abundant ostracods in the study localities. To evaluate the linkage between ostracods and contaminated sediments, metal concentrations in the sediments were measured using inductively coupled plasma optical-emission spectrometry (ICP-OES). We observed a significant spatial difference in elemental distribution in sediment samples, and some elements are of higher contributions than the average marine sediment composition. The quantitative analysis of benthic ostracod associations was processed statistically with geochemical data and differentiated the study area into two environments, based on the degrees of contamination. Quseir is encompassing the most contaminated stations. It is characterized by sediments richer in heavy metals, higher silt, and higher organic matter contents. Contaminated environments favor the presence of pollution-tolerant species (e.g., J. borchersi, C. torosa, L. ghardaqensis, G. triebeli, M. striata, A. reticulata, and partly, C. dimorpha). Conversely, Ras Gharib is less polluted with metals and organic matter, and is characterized by pollution-sensitive species (X. rhomboidea). Noteworthy, a low density of ostracods was observed at the expense of the diversity around contaminated stations of the Quseir site.
Similar content being viewed by others
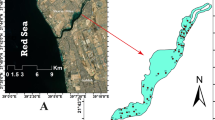
Explore related subjects
Discover the latest articles, news and stories from top researchers in related subjects.Avoid common mistakes on your manuscript.
Introduction
Worldwide seas are suffering from several anthropogenic influences such as organic pollution, heavy metals contamination, eutrophication, and oxygen consumption (Irizuki et al. 2015). The most impacted areas are usually coastal areas, and it is vital to determine the timing and reasons lead to such environmental deterioration. Several studies effectively used the paleontological approaches to infer environmental changes that occurred during the past hundreds of years (e.g., Frenzel and Boomer 2005; Yasuhara et al. 2012; Irizuki et al. 2015). The nearshore Red Sea area is frequently affected by human actions, such as industrial and recreation activities, mining, tourism, and oil industry that could have initiated several contaminants into the marine ecosystem, which can produce severe effects on different trophic levels. Several researchers have focused on organisms that are useful for biomonitoring of anthropogenic disturbances (e.g., El-Sorogy et al. 2006; Youssef et al. 2017; El-Kahawy et al. 2018). For example, some general models were suggested concerning the status of the faunas relative to the degree of eutrophication and heavy metals (e.g., Orive et al. 2002; Youssef et al. 2017). Other specific studies have assessed the impact of attributes of eutrophication on ecosystem functioning (e.g., hypoxia; Diaz and Rosenberg 1995).
Ostracods are a well-documented group of crustaceans that are worth studying due to their wealth of characteristic features with a strongly calcified and tiny carapace, and their easy fossilization (e.g., Mohammed et al. 2012). They dwell in marine, brackish, and fresh aquatic environments. Ostracods are a group of meiofauna, which can be used as bio-indicators for stressed settings in a variety of environments (e.g., Anadon et al. 2002; Boomer and Eisenhauer 2002). They have long been used as bio-indicators for salinity, pH, and temperature (e.g., Chivas et al. 1986; Kemp et al. 2012). Recent studies have drawn the attention to environmental threats (e.g., influence of sewage discharge, agricultural waste, industrial wastewater, heavy metals enrichment, etc.) by analyzing ostracods shells (e.g. Ruiz et al. 1997; Eager 1999). Yasuhara et al. (2003) described ostracods as a sensitive group to anthropogenic effects. Hence, living ostracods may respond by migration to the unpolluted areas (Samir 2000). In chiefly unpolluted stations, ostracods respond as lowering faunal diversity and density of population (Ruiz et al. 2004) and may disappear from the heavily polluted stations (Bergin et al. 2006). A negative correlation was noticed for the ostracod density and diversity due to oil seepage (e.g., Steichen et al. 1996). On the other hand, no significant change in the ostracod community at heavily oiled sites (e.g., Moore et al. 1997). It is noteworthy that the environmental stress may not be only owing to anthropogenic impacts, but also due to natural stressful conditions such as very high salinity (e.g., Pint and Frenzel 2017). Some taxonomic studies on recent ostracods have been focused on the Red Sea, relating species to their environment (e.g., Hartmann 1964; Bonaduce et al. 1983; Helal and Abd El-Wahab 2012; Mohammed et al. 2012). However, they lack the usage of ostracods to monitor the Red Sea coastal pollution. Therefore, this is the first study that focusing on ostracoda as a bio-indicator for different kinds of contaminations in the Egyptian Red Sea coast.
Several alarming spots along the Red Sea coast of Egypt delineate the importance to execute shortly spaced biomonitoring studies to convoy the changes in the marine environment. Hazards can be noticed nowadays due to elevated pollutants such as phosphates mining (Quseir city), oil industry (Ras Gharib city), sewages, and landfill leachates during the past decades (e.g., El-Taher and Madkour 2014; El-Kahawy et al. 2018).
In this regard, the main objectives of the present study are to:
-
1.
study the taxonomy of modern benthic ostracods and their distribution in the Red Sea coast.
-
2.
investigate the effects of anthropogenic activities on ostracods species, their abundances, and diversity.
-
3.
build statistical processing to distinguish the elemental geochemical anomalies and highlight the alarming spots within the study area.
Study area
The study area encompasses two sites (Ras Gharib and Quseir) along the Red Sea coast (Fig. 1). The Ras Gharib site is affected by onshore and offshore hydrocarbon exploration activities, which are distributed nearby the port (Fig. 1). This area includes two jetties: one stands for prohibiting the pollution and another old one stands for fishing. A few boats were observed around the latter jetty during the field survey. Moreover, the effect of clastic terrigenous input caused by flash floods is not uncommon (e.g., Hegazy and Effat 2010). The beach is wide (~ 8 m), gravelly to sandy, and has some wood remains and solid wastes. The beach is followed by a narrow tidal flat zone composed of coarse sand with a gradient slope toward the east direction. The tidal zone is followed by a large back reef zone (~ 1-km width) to the reef edge. The bottom floor is inhabited with some spots of algae and coral reefs. Mollusca dominated the bottom macro fauna communities both in their species compositions and their intensities that were represented with 87.6% (El-Komi et al. 2002).
The Quseir site is bounded by the Red Sea Phosphate Company northward, and the Quseir old buildings southward. It includes two marinas: one stands for tourist boats and another stands for fishing boats. The beach is sandy, has a width of ~ 5 m, and inclined by an angle of ~ 4°. The tidal flat zone has a gentle slope to a maximum depth of 2 m and inhabited by some green algae and seagrasses spots. The tidal flat zone is followed by a wide back reef zone with an average depth of 5 m including coral colonies in good conditions, especially near the bottom of the fishermen Marina. Macroalgae and seagrasses are widely distributed. Macroalgae is found in scattered patterns at a depth of 50–60 cm. Creeping green algae such as Caulerpa racemosa is found in small aggregates, covering vast areas of the sandy substrate. Small quantities of the green algae Halimeda tuna were found within the branches of corals. Seagrass was found as spots-forming large meadows, growing in the sandy mud substrate. The seagrass Halophila stipulacea is the dominant species beside Halodule uninervi.
The climate of the Red Sea is mainly controlled by the NW to NE wind, which creates NE-SW oriented water waves (Mansour 1995). The tide is semidiurnal, maximum peak every 12 h with a mean tidal range of about 0.5 m. The water depth during the high tide is ~ 0.9 m and during the low tide is ~ 0.3 m. The rainfall over the Red Sea area is negligible. It is mostly in the form of short duration winter showers (10–15 mm/year; Morcos 1970). A complete mixing of the water column occurs, which causes higher waves and drives alongshore current in the summer southward (Mansour 1995). The currents redistribute terrigenous deposits derived into the sea with no density stratification in the water column. This can be mirrored from the temperature and salinity measurements, which demonstrate a non-significant difference between surface and bottom waters (e.g., Piller and Pervesler 1989).
Material and methods
Sampling
Eighteen sediment samples were collected using a plastic coring tube (10 cm long and 10-cm diameter). Nine samples were collected from each site along three transects from the beach toward the open sea (Fig. 1). Few milliliters of 70% ethanol were added in the field to preserve the sediment samples from microbial activities, which would increase the organic carbon content as an artifact. Some samples were collected using SCUBA (Self Contained Underwater Breathing Apparatus) diving in the offshore waters of the study sites. The uppermost centimeters of the samples were assumed to be impacted by industrial and municipal activities (Fig. 2); therefore, we focused on this horizon. Salinity, pH, and water temperature of the seawater were measured for each sampling station using a Hydrolab Surveyor-4 Instrument in July 2014 (Table 1). Water depth at each station was determined using an echo-sounder instrument except the shallowest stations (< 1 m) were measured using the water depth meter. The water depth in the two sites ranged from 0.1 to 7.0 m.
Geochemical analyses of sediments
Chemical analysis was carried out on sediment samples in Acme Labs, Bureau, Canada. Aliquots of 0.5 g of fine sediments (63 μm) were analyzed using Inductively coupled plasma-emission spectrometry (ICP-OES) (Table 2). The samples were digested with a modified aqua regia solution (0.5 ml H2O, 0.6 ml concentrated HNO3, and 1.8 ml concentrated HCl) for 2 h at 95 °C. Samples were cooled down, diluted to 10 ml with de-ionized water. Samples were then analyzed using a Perkin-Elmer OPTIMA 3000 Radial ICP for the element suite. For quality control (QC), replicates (~ 25% of the total number of samples) were analyzed under the same procedures. A series of geochemical reference standards (USGS 1995) were used as controls to ensure the quality control and accuracy of the analysis. A standard matrix and a blank were running every 13 samples. The detection limits of the measured metals are Cu (1 ppm), Zn (1 ppm), Mn (2 ppm), Cd (0.5 ppm), As (2 ppm), Pb (3 ppm), Cr (1 ppm), Ni (1 ppm), Mo (1 ppm), P (0.001 ppm), Fe (0.01 ppm), and Co (1 ppm).
The total carbonate content was measured by an acid treatment weight loss technique (Gross 1971). Dried sediment was treated with a 2.5 N hydrochloric acid solution and the residue was collected on a 0.45-μm filter. After drying the filter, the measured weight loss was transformed into the percent of carbonate (Table 1). The determination of organic matter was made by the sequential weight loss at 550 °C (Dean 1974).
About 20 g were used for grain size analysis using standard sieves sedimentation technique after Folk and Ward (1957). The finest fractions were analyzed using the Pipette method (Suguio 1973).
Ostracod analysis
A constant weight (200 g) was taken from the top of undisturbed sediment. In the laboratory, the samples were washed with distilled water and sieved through 63 μm to remove salts. The samples were treated with hydrogen peroxide (H2O2), dried at 60 °C, and kept in small bottles before the analysis. The samples were washed and dried, then split into aliquots using a micro-splitter. The picking of ostracods was done on > 125-μm fractions. The picked specimens (disconnected valves and the articulated carapaces) were counted; a single valve was regarded as 0.5 specimen and the carapace as one specimen. This method of counting was used by several authors (e.g., Maddocks 1985; Mette and Roozbahani 2012; Helal and Abd El-Wahab 2012).
The ostracod assemblages were identified according to Moore (1961) and Hartmann (1964). The identified ostracod taxa were photographed by scanning electron microscope at the Egyptian Mineral Resources Authority (EMRA) using the machine JSM 6063LA.
Statistical analysis
The cluster analysis was accomplished using the SPSS Program (V. 20). A sample-by-sample (Q-mode) cluster analysis was applied here to a dendrogram classification of the studied samples. R-mode cluster analysis was conducted as well to construct a dendrogram classification of the dominant species and their species associations. Due to the scarcity of the living ostracod individuals, we used the dead individuals only in the statistical analysis. About 300 adult individuals were recovered from each sample, which was considered as a representative number of statistical treatments. In the studied samples, a species with occurrence of more than 2% relative abundance of the ostracod assemblages in at least one sample were involved in the data matrix in the cluster analysis. The counted data of the dominant species were standardized using z score transformation and with absolute values before performing the cluster analysis. The hierarchical cluster analysis was performed with squared Euclidean distances for the variables by using Ward’s method on the standardized data matrix.
Detrended correspondence analysis (DCA) was performed on the dominant taxa of more than 2% relative abundance of the total samples, to choose either applying unimodal method (canonical correspondences analysis, CCA) or linear method (redundancy analysis, RDA). The length of the gradient is 0.9 SD units (lower than 4), which means that we can apply the linear mode. Thus, linear analysis (RDA) will be more desirable and recommended than unimodal analysis (Leps and Smilauer 2003). Redundancy analysis (RDA) was conducted here using CANOCO software (version 5.12) to investigate the community’s relation to abiotic parameters at the studied stations (e.g., Elshanawany 2010). To classify and identify the environmental variables, the Monte Carlo permutation test (500 permutation, P < 0.05) is used in RDA analysis. The arrows radiating from the center of the RDA chart indicate the direction of maximum variation. The arrows’ length reveals the relative significance of each environmental variable. The angle between the environmental arrows is inversely proportional to the strength of their relationship.
A multivariate analysis (factor analysis) was performed using SPSS software via the dimension reduction category to decipher inter-elemental relationships. The factor analysis (FA) permits the projection of a large set of variables (samples and variables) into a reduced form (Gabrié and Montaggioni 1982). This analysis was done by the Varimax method and z standardization. The data matrix comprises 18 samples and 19 variables (Fig. 6). Several spatial bubble plot maps were constructed based on the graduated symbol method using Arc GIS software (v. 10.3.3).
Diversity indices
The computational program utilized in the present study is the Paleontological Statistics Program (PAST), version 3.20 (e.g., Hammer et al. 2009). The following concepts and formulas are used.
-
1.
Density describes how many individuals occur per unit area.
-
2.
Abundance is the number of individuals per sample.
-
3.
Dominance index:
D = ∑ (ni/n) 2, where ni is the number of individuals of taxon i and n the total number of individuals of all species. Its significance varies from 0 (all taxa are equally present) to 1 (only one taxon dominates the community).
-
1.
Diversity index (Shannon index) considers the number of individuals as well as the number of taxa. It varies from 0 (single taxon communities) to high values for several taxa communities (Shannon and Weaver 1948).
Results
Environmental variables
Substrate
Grain size data of the studied sites (Fig. 3) indicated a seabed sediment type with a high sand fraction (over 90% medium to fine sand grains) of sub-rounded morphologies. The samples from the deepest water showed the highest value of very fine sands with silt and/or clay fractions (Fig. 3). This is a common feature mirroring the hydrodynamic condition. The silt and clay fractions are of higher contribution in Quseir than in Ras Gharib site (Fig. 3). Ras Gharib demonstrated a high percentage of coarse sediments close to the beach (such as stations G.1 and G.4, excluding G.3). These stations revealed < 95% of coarse sand (Fig. 3). Nevertheless, the highest percentage of the fine particles was observed around stations G.5 and G.7 with values of 7.4% and 6.2% for silt plus clays, respectively (Fig. 3). The fine sediment-enriched stations are Q.2, Q.4, Q.5, and Q.6 (5%, 9.3%, 8.1%, and 5.1%, respectively; Fig. 3), which are located in central and southern part of the Quseir area (Fig. 3). Conversely, the coarse sand-enriched stations are Q.1, Q.3, Q.7, and Q.9 (98.8%, 98.3%, 99.5%, and 99.1%, respectively; Fig. 3).
Hydrological parameters (salinity, pH, and temperature)
Ras Gharib stations showed a higher salinity range of 40.91–41.61 PSU, whereas Quseir stations showed a lower range of 40.3–40.9 PSU (Table 1 and Fig. 4). During the field survey, the water temperature was ranged from 20 to 26.6 °C, whereas the pH was slightly alkaline (7.4–8.3; Fig. 4). Detailed current information is lacking in the Red Sea area. This is because they are weak and variable (spatially and temporally) and are mainly governed by wind action (Rasul et al. 2015).
Total organic carbon content (TOC %)
The Ras Gharib site exhibited lower organic matter content than the Quseir site (Fig. 5). The TOC% increases toward the southern transect of the Quseir site; however, its values varied among all stations (Fig. 5). In the Ras Gharib area, the lowest TOC% value was measured in G.9 (~ 0.7%) and the highest value was noted in G.8 (2.7%; Fig. 5), averaging 2.1%. Quseir stations displayed high organic matter content (averaged 5%), especially Q.1 and Q.2 (Table 1, Fig. 5). Overall, station Q.2 showed the highest TOC value (12.1%) of the study two sites (Fig. 5), followed by Q.1 (10% TOC; Fig. 5).
Heavy metals in sediments
The geochemical analysis data for the analyzed heavy metals in the two sites are shown in Table 2.
Ras Gharib site
The Fe content revealed its lowest content around a shallow station G.4 (1500 ppm), whereas the highest content was measured around station G.7 (19,900 ppm). The Fe content increased, to some extent, away from the coastline in two stations (e.g., G.9; Table 2). The indicated contributions were much lower than that of the average marine sediment (32,000 ppm; Li 2000). On the other hand, the P concentration was valued higher than the average marine sediment (600 ppm; Li 2000) with a maximum content was observed in station G.9 (1600 ppm) and a minimum was observed in station G.4 (230 ppm), averaging 558 ppm (Table 2). The B concentration showed low values in the overall stations; however, the maximum concentration was found around station G.6 (47 ppm) and the minimum was found around station G.9 (22 ppm).
In the study of shallow sediment, Cu concentration was of a maximum contribution in station G.1 (186 ppm) and its minimum contribution was observed in station G.3 (3 ppm), averaging 41 ppm. Nonetheless, Cu has a content of 43 ppm in the average shallow marine sediment (Callender 2005). Moreover, the average Cu content is slightly above the effect range-low (ERL, 34 ppm), but less than the effect range-median (ERM, 270 ppm) indicated by Long et al. (1995). This indicates that Cu has a slightly higher contribution than normal shallow marine sediments as well as the biological guideline value for this metal, particularly in sandy and silty stations (e.g., G.1 and G.5; Table 2). The Ni concentration in the study bottom sediments reached its maximum at station G.9 (22 ppm), while the whole remaining concentrations have 1–3 ppm (Table 2). These values are, generally, lower than the average shallow marine sediment composition (44 ppm). Generally, Cr increased in the north direction and was of a maximum concentration at station G.9 (27 ppm), whereas the minimum concentration was measured around station G.4 (2 ppm; Table 2), which are negligible values compared to the average shallow marine sediment composition (79 ppm) and the ERL and ERM values as well (81 and 370 ppm, respectively). The Pb concentration in the study sediments ranged from 3 ppm in most of Ras Gharib stations to 15 ppm in station G.5 that is lower than its content in the average shallow marine sediment (23 ppm; Callender 2005). The Cd concentration increased in the shallow area around Ras Gharib harbor (0.8 ppm at G.4; Table 2) and decreased toward the deeper water to 0.1 ppm (G.6; Table 2), averaging 0.4 ppm. It is generally higher than the Cd content in the average shallow marine sediment (0.2 ppm), but still less than the ERL and ERM values (1.2 and 9.6 ppm, respectively). It is noteworthy that stations G.9 and, to some extent, G.6 contained high concentration of several elements, which could be revealed to their high clay content (Table 2).
Quseir site
The Fe content in the Quseir marine sediment ranged from 5500 ppm in station Q.5 to 12,500 ppm in station Q.2, averaging 8444.4 ppm (Table 2). Alike the Ras Gharib, these values are noticeably lower than the average marine sediments. Nevertheless, the P concentration was valued as the highest in station Q.5 (53,000 ppm), while the lowest concentration was recorded in station Q.3 (790 ppm), with an average of 13,265.5 ppm (Table 2). These contents are significantly of higher contribution compared to the average marine sediment value as well as the Ras Gharib P contents, which is, most likely, linked to phosphorite mining and exporting activities.
Cu showed a maximum observed content of 17 ppm in stations Q.1 and Q.2, whereas its lowest content (3 ppm) was observed in station Q.3, averaging 9.5 ppm. Unlike the Ras Gharib site, the Cu content is of lower contribution than the average shallow marine sediment as well as the ERL and ERM values of Cu (Table 2). The highest measured value of Pb was recorded in station Q.4 (29 ppm), averaging 12 ppm. Its content was, partly, higher than the average shallow marine sediment (e.g., Callender 2005; Table 2), but still lower than the ERL and ERM values (46.7 and 218 ppm, respectively) of Long et al. (1995). Furthermore, Cd exhibited expressively higher contributions than the average shallow marine sediment (0.2 ppm) with a highest measured value of 3.8 ppm (Q.5) and a lowermost contribution of 0.5 ppm in stations Q.3, Q.6, and Q.9, with an average of 1.6 ppm. The content is, in average, higher than the ERL value (1.2 ppm), but lower than the ERM value (9.6 ppm). Remarkably, stations Q.4 and Q.5 displayed a high, or may be the highest, concentration of several elements (As, Cd, Pb, Zn, P, and Mn), which could be revealed to their proximity to phosphate works (Table 2).
Correlation matrices were conducted for the study two sites, which showed various correlations among elements in the two sites (Table 3). This revealed different sources, migration, and behaviors from one another. The Ras Gharib site showed a few P-associating elements (Ni, Mn, and Cr), whereas the rest of elements were mostly related to anthropogenic sources (Table 3). On the other hand, the Quseir site showed a group of P-related elements (Pb, Cd, Mn, As, Zn, and B), which revealed that the phosphorite mining and exportation are the most effective anthropogenic source in the area (Table 3).
At the Ras Gharib, the first and second FA accounted for 41.99% and 19.4% of the total variance, respectively (Fig. 6a). Correlation and correspondence analyses of results from the Ras Gharib site indicate that Cr showed an intimate correlation with Mn (r = 0.97, p < 0.05) and forming a cluster, which supports the terrigenous input along with the low concentrations and their distribution pattern. At Quseir site, the first and second FA accounted for 48.08%, and 28.11% of the total variance, respectively (Fig. 6b). Phosphorus exhibited the strongest significant positive correlation with B (r = 0.99, p < 0.05). P also was found to be positively related to Mn (r = 0.98, p < 0.05), As (r = 0.93, p < 0.05), Cd (r = 0.86, p < 0.05), and Pb (r = 0.81, p < 0.05).
Benthic Ostracods
Both Quseir and Ras Gharib ostracod associations are reasonably similar in terms of the high abundance of the adult valves. Mostly, the recovered ostracods from the two sites were found dead; however, very few were perceived living. Moreover, the disarticulation, fragmentation, and abrasion processes were the most observed taphonomic features in the studied two sites. Aside, the most abundant taxa, in descending order, were the following: Jugosocythereis borchersi, Ghardaglaia triebeli, Loxocorniculum ghardaqensis, and Cyprideis torosa (respectively, Appendix 1).
In the studied shallow-water areas (0.1–7 m depth), twenty-three benthic ostracod species were identified, belonging to 20 genera and 13 families (Figs. 7 and 8, and Appendix 2–4). The family Trachylebrididae constituted the highest percentages among the ostracod fauna, representing 28.8% of the total ostracod associations in the study area (Appendix 2). The most abundant species was Jugosocythereis borchersi, constituting 18.5% of the total ostracod association. The family Cytheridea (Loxocorniculum ghardaqensis) was the second significant constituent and comprised 14% of the total assemblage in the study area. The third most abundant family was the Paracyprididae (Ghardaglaia triebeli) and comprised 13.7% of the total assemblage in the area of study (Appendix 2). The fourth dominant family was the Cytherideidae (12.6% of the total families) that is represented by the highest constituting species Cyprideis torosa.
The most abundant species (Jugosocythereis borchersi) exhibited a low number of abundances in coastal stations (G.4, G.5, G.7, Q.4, Q.5, and Q.7), which are closer to the pollution sources. This species was observed mostly in the silty and muddy sand facies, where sometimes the TOC% was the highest (e.g., Q.2; Fig. 7). Loxocorniculum ghardaqensis was the second dominant taxon in both sites. Its relative abundance varied allover stations. It had low values in Ras Gharib stations, whereas a high occurrence was observed in Quseir stations. This could be attributed to its preference to fine-grained substrate (e.g., G.8, Q.1, and Q.2; Fig. 7). A very high relative abundance of this species was recorded in the offshore and organic matter–enriched station (Q.2), and its abundance decreased in the polluted stations (e.g., Q.4 and Q.7; Fig. 7). Ghardaglaia triebeli was the third dominant taxon in the studied samples. The TOC-enriched stations were typified by its relative abundance on muddy substrate (e.g., G.8, Q.1, and Q.2; Fig. 7). The fourth most abundant species was Cyprideis torosa, which showed its highest abundance in sand-rich stations (e.g., Q.9, Q.8, and Q.1; Fig. 7).
The total standing yield abundance of benthic ostracods at the Quseir site was generally high and ranges from 1705 individuals/50 g (Q.2) to 629 individuals/50 g (Q.4). On the other hand, the abundances at the Ras Gharib site were moderate, ranging from 583 individual/50 g (G.8) to 304 individual/50 g (G.5).
The interrelationship between density and diversity was displayed by the diversity indices. Firstly, the Ras Gharib site displayed high diversity indices, especially the dominance index in comparison to the Quseir site. On the other hand, the Shannon index was high at Quseir rather than Ras Gharib. The highest values of the Shannon index were observed around station G.8 (2.26) in the Ras Gharib and Q.6 (2.57) in the Quseir sites. Aside, the Shannon index showed low values at stations G.7 (1.64) and Q.4 (2.23) of Ras Gharib and Quseir, respectively (Fig. 8). The highest value of the dominance index in the Ras Gharib was recorded around station G.7 (0.23), whereas the lowest value was found around station G.8 (0.12) (Fig. 8). On the Quseir site, the highest dominance index was observed at station Q.4 (0.13), whereas the lowest value was found at station Q.6 (0.09). Additionally, the species number of ostracods in Ras Gharib stations was low; the lowest station was noted around G.7 (7), whereas the highest was found around stations G.8 and G.9 (14). Accordantly, the species number of Quseir stations showed leaped behavior compared to Ras Gharib, where Q.6 was the highest and Q.4 was the lowest in species number of the Quseir site (Fig. 8).
Ostracod-environmental conditions using statistical analyses
Cluster analysis
The cluster analysis (CA) in the study area indicated individual clusters, characterizing different levels of contamination. Two main clusters (A and B) characterized the studied samples and taxa. Each cluster consisted of two sub-clusters A1, A2 (A2.1 and A2.2), B1, and B2 (Fig. 9). Cluster A was distinguished by the presence of Ras Gharib samples and heavy metal-enriched stations of the Quseir site (Q.4 and Q.5) (Pb, Zn, Fe, Ni, Cr, Cd, Mn, and B; see the “Redundancy analysis” section), fine-grained sediments, and less saline warm water. Sub-cluster A1 comprised the deep-water stations at the Ras Gharib (G.8, and G.9) and is characterized by the dominance of X. rhomboidea, C. dimorpha, and H. rubrimaris as evidenced in the R-mode analysis (Fig. 9). Sub-cluster A2 of the Q-mode, which was subdivided into two sub-clusters, comprised the rest of Ras Gharib stations as distinguished in the A2.1 sub-cluster and evidenced in R-mode analysis by dominance of X. ghardaqae. Two stations of the central transect of Quseir harbor (Q.4 and Q.5) were forming A2.2 sub-cluster and showed a sharp decrease in the abundance of A. reticulata and M. striata (Fig. 9).
Cluster B comprised Quseir stations Q.1–Q.9 (except Q.4 and Q.5; Fig. 9). These stations are relatively less polluted with metals and deeper (Fig. 9). The stations of sub-cluster B1 (Q.1, Q.7, Q.8, and Q.9) were typified by high sand content, and moderate TOC% and metal contents. These stations are fairly located away from the pollution effect and mainly dominated by J. borchersi (Fig. 9). The stations of sub-cluster B2 comprised stations Q.2, Q.3, and Q.6. They were of relatively low concentrations of some heavy metals and TOC% around stations Q.3 and Q.6, whereas, around station Q.2, the TOC% and some heavy metals were of high contents (e.g., Ni, Cr, Fe, Cu, and Zn). On the other hand, they were of high silty sand content, pH, and low salinity. They were dominated by Ghardaglaia triebeli, Cyprideis torosa, and Loxocorniculum ghardaqensis (Fig. 9).
Redundancy analysis
Based on the Detrended correspondence analysis (DCA), the data set was evaluated with Redundancy Analysis (RDA). The first and second RDA axes explained 52.5% and 11.92% of the total variance in the data set, respectively (Fig. 10). Other non-significant axes were not considered. The RDA revealed species and stations discrimination, based on their metal concentrations and other environmental factors (substrate, water depth, and TOC%). The environmental variables chosen were for the highest significant variations, whereas the neglected variables such as sand, clay, pH, and temperature showed less significant variations.
Axis 1 discriminated the stations into two groups based on the pollution level of the station (Fig. 10). On the negative side of axis 1, the first group included the shallow stations of Ras Gharib site (G.1–G.6 and G.8), which are less exposed to metal pollution other than Cu (Fig. 10). Comparatively low heavy metal concentrations, high Cu concentration, and water depth were regarded as the main controlling factors. On the positive side; however, the second group is located, which comprised most of Quseir stations that are more subjected to organic matter and heavy metal sources (Q.2–Q.8), and their dominating species (Fig. 10). This group was distinguished by high species diversity at the expenses of species density around the Quseir stations. Number of ostracods species are located on the right side, being occupied by Quseir stations. These species are J. borchersi, C. torosa, X. ghardaqae, Alocopocythere reticulata, Moosella striata, Hiltermannicythere rubrimaris, Cytheroma dimorpha, and L. ghardaqensis (Fig. 10).
Discussion
Almost all the ostracod specimens under investigation are dispersed in the studied marine sediment. The observed taphonomic features suggested a high energy marine ecosystem with appropriate and nearly constant pH and pCO2.
Distribution, diversity indices, and abundances of ostracoda
The two studied sites are shallow sheltered environments dominated by vital elements (Helal and Abd El-Wahab 2010). The recorded ostracods were represented by benthic, phytal, and shallow-water species. The families Trachylebrididae, Paracyprididae, Cytherideidae, and Cytheridea dominated the ostracod assemblage in the study area (Appendix 2). Species of these families live in association with the seagrasses, algae, and mangrove trees, which are widely distributed on the coastal part of the Red Sea (Helal and Abd El-Wahab 2012) and appear as they are slightly suffering from deterioration as well. The impact on corals and algae is dealt in a follow-up study by the co-authors (not published). These species, for instance, are J.borchersi, M. striata, G. triebeli, C. torosa, and L. ghardaqensis.
An important measurement for the environmental stress consequences on benthic micro-fauna is the species diversity (e.g., Ruiz 1994; Elshanawany 2010). Thus, the effects of different contamination sources generated significant changes in the density and diversity of ostracods (e.g., Ruiz et al. 2013). Nevertheless, some species such as C. torosa and C. dimorpha are resistant to organic contamination (Lim and Wong 1986) and consequently low species diversity typified contaminated environments (Alin et al. 1999). Numerous authors (e.g., Poquet et al. 2008; Elshanawany 2010; Ruiz et al. 2013) considered the variation in species diversity of micro-fauna as a contamination response. They conveyed that ostracods are usually very limited or absent in polluted waters close to industrial activities. Furthermore, Van der Merwe (2003) inferred that mining activities have similar effects on ostracod assemblages.
The present data reveal that the type and magnitude of pollution to the marine benthos are not severe to cause a complete disappearance of ostracod, as in the case of extremely polluted sites (e.g., Carman et al. 2000; Lenihan et al. 2003). However, the pollution leads to ostracod communities with a high dominance index and a low abundance with a limited number of opportunistic species (e.g., Millward et al. 2004). This relationship is apparent in the highest values of dominance index occurring in stations Q.4 and Q.5, which have elevated concentrations of heavy metals (Table 2). Additional aspect is the low to moderate diversity and high density in stations Q.1 and Q.2, which could be revealed to the high organic content and reduced oxygenation. Species diversity increases in the deep-water direction and could be related to environment stability, along with less stress conditions for a benthic ecosystem (e.g., Ruiz et al. 1997). The species diversity usually increases with water depth in a shelf environment (e.g., Bonaduce et al. 1983). The diversity declines toward shore (Fig. 7) that could be attributed to the increased salinity and pollutants as in stations G.1, G.4, and G.7 as well as Q.4, Q.5, and Q.7. In accordance with Samir (2000), this research shows the importance of faunal diversity and density as bio-indicators for evaluating the presence of polluted areas. The observation of a low ostracods’ density at the expense of their diversity, especially around the contaminated stations (Q.4, Q.5, G.5, and G.7) matches well with Liljenstroem et al. (1987).
Ostracods as a bio-monitor of pollution
Two major distinctive environments were noted for the ostracod community in this study:
-
Group I: This group comprises most of the dominant species, which links all of the Quseir stations that are characterized by elevated contributions of TOC, silt, and heavy metals specially Q.4, Q.5, and Q.2 stations, respectively. The tolerant species for the Ni, Cr, Zn, and TOC in the upper right quarter are the following: J. borchersi, C. torosa, L. ghardaqensis, G. triebeli, M. striata, A. reticulata, and to some extent C. dimorpha. On the other hand, the occurrences of X. ghardaqae and H. rubrimaris were lessened due to the adverse effect of Pb, P, As, and Cd enrichments, especially in Q.4, Q.5, and Q.7 stations. Accordingly, the diversity and abundance for these two species increased toward stations Q.2, Q.3, and Q.6 away from the direct effect of the heavy metals. The species A. reticulata was previously reported as dominant onto fine-grained substrate with elevated organic matter in the Red Sea (Helal and Abd El-Wahab 2004). Our study verifies that A. reticulata is tolerated in the environments of elevated contents of organic matter, Zn, and some other metals concentration. It is worth to mention that stations Q.4 and Q.5 are the most contaminated stations in the study sites, which is mirrored by decreased abundances and diversities. The sediment elemental geochemical data of ostracod assemblages reveal that this group is of moderate to high pollution level.
-
Group II: This group involves most of Ras Gharib stations (except, G.9) and comprises only X. rhomboidea of the ostracod species and away from significant factor (Cu). The deviation of such species from the positive side on the ordination chart displayed its heavy metal vulnerability. Thus, the high relative abundance of this species typifies stations with sandy sediment, low to moderate TOC%, elevated pH, and depleted heavy metal contribution. This group is distinguished based on the low faunal diversity and abundance of ostracods around stations (e.g., G.1, G.5, and G.7) and the geochemistry of sediments, which reveals that this group is of a low to moderate pollution level. The low diversity and abundance can be attributed to the coarser sandy bottom facies especially at the Ras Gharib beside the general observation that Cu, Cd, and Co attain higher concentrations in Ras Gharib samples than that of Quseir site (Table 2).
Ostracod abundance-environmental factors relation
The amount of organic matter present in the sediment can influence the abundance of meiofauna, including that of ostracods (Mazzola et al. 1999; Mirto et al. 2000; Lili et al. 2013; Irizuki et al. 2015). Mirto et al. (2000) inferred that ostracod abundance decreased after the installation of fish and mussel farms, because of the bio-deposition farming complexes and oxygen deficiency. However, they did not assess whether the response to organic matter is species-specific. Irizuki et al. (2015) used the relationship between the TOC% and the most abundant ostracod species to explore the organic matter influences on ostracod density. Lili et al. (2013) considers species-specific response and examined the correlation between TOC content and abundances of several ostracod species for environmental change biomonitoring. However, their studies included surface sediments from different sites, water depth, and substrates, which were influenced by different environmental factors and make it difficult to identify specific causal relationships.
The present study ostracods abundance and their faunal diversity decreased around stations of high organic matter content. We verified that the salinity and type of substrate are not of a degree of variability to be considered in the correlation-relationship. Besides, an environmental factor such as water temperature is likely to remain unchanged in each site throughout the study interval. Moreover, all the dominant ostracods found in the present study were shallow marine mud-dwellers, and all samples were composed of silty to muddy sand. This is apparent by the weak negative correlation of silt through the ordination analysis of RDA (Fig. 10). The results of ostracods identification suggest that the association can be considered as of autochthonous (the same zoogeography) species of the Red Sea domain; hence, they represent indigenous assemblages. Most of the recovered species were dead, mainly because of the environmental stress due to the aforementioned causes. Other lesser extent causes would have also affected such as sampling.
Heavy metal clusters
Various studies have discussed the linkage between ostracod abundance and heavy metal contamination (e.g., Bodergat and Ikeya 1988; Watzin and Roscigno 1997; Ruiz et al. 2000, 2004; Lee and Correa 2005; Bergin et al. 2006; Irizuki et al. 2011, 2015). They inferred that an increase in some metals (e.g., Cu and/or Zn) concentration mostly causes a decrease in the ostracod abundance. As a result, ostracods may migrate away or disappear in the highly contaminated sites. It is worth to mention that elements P, Fe, Pb, Zn, and Cu have significantly higher contents in the Quseir site than the Ras Gharib site (Table 2). Additionally, their contributions are noticeably higher than what was recorded by Mansour et al. (2000) and El-Sorogy et al. (2006) in the same study area as well as by Mansour et al. (2011) for only the Quseir site. This implies that the content of these elements in the Red Sea shallow marine sediment increases on a short-term scale. Cd is of a remarkable high contribution in the two studied sites, which is higher than that of the average shallow marine sediment and it could be of a hazardous effect. Furthermore, the Cu and Pb content of the study sites can be considered as a whistling alarm for a potential toxicity in Ras Gharib and Quseir areas, respectively. Similar trace metal contributions were delineated in a recent study (Nour and Nouh 2020).
Iron plays an important role in trace metal scavenging and accumulation into sediments (Badr et al. 2009). Trace metals are absorbed or precipitated in the form of Fe oxides, forming a thin layer at the sediment surface (Duchart et al. 1973). This process is most probably the reason for the noticeable contributions of trace metals in the sediment samples.
The field observations confirm that the main source of pollution in the Ras Gharib site is shipping activities and to a lesser extent oil exploration around the study area. The Quseir harbor; however, is suffering from city sewage and other anthropogenic activities in addition to phosphate mining (Fig. 1). These several types of pollution have a serious effect on the ostracod community. The correspondence analysis of the present work confirms the correlation analysis, where P forms a cluster representing all metals of anthropogenic sources of pollution at Quseir site (Table 2). This corresponds to stations Q.4 and Q.5, which display the lowest abundances of ostracods at the Quseir site and might be due to the eutrophication problem. Moreover, the correspondence analysis shows that all redox-sensitive metals such as Pb, Cd, As, Mn, and Fe come in association with P, especially at the Quseir site. No significant correlation was noticed for Cu with other metals, specifically at the Ras Gharib site. This infers a different behavior of Cu in the study areas and its deposition is influenced by either terrigenous supply (the Ras Gharib site) or anthropogenic input (the Quseir site).
The cross-plot between Cr content and ostracod abundance (Fig. 11) shows positive correlation coefficients with the highest abundant ostracod species in the two sites (Fig. 11). Ghardaglaia triebeli had a significant positive correlation with Cr (r = 0.74). The correlation coefficients of the selected ostracod species are the following: Alocopocythere reticulata (r = 0.67), Jugosocythereis borchersi (r = 0.64), Moosella striata (r = 0.59), Hiltermannicythere rubrimaris (r = 0.59), and Cyprideis torosa (r = 0.52), which reveals a moderate to strong correlations (Fig. 11). The limited data we have suggest that they are tolerant species and could be used as bio-indicators for heavy metal contamination.
Conclusions
Benthic ostracods are used here to assess the ecological settings of the marine benthic ostracods on the coastal environment of the Red Sea. Quantitative analysis of benthic ostracods was coupled with geochemical data and statistical processing to discriminate the study area into two distinctive segments accompanying their environmental character and pollution level. The Quseir area is characterized by sediments relatively enriched in heavy metals, high silt content, and elevated organic matter. It encompasses the most contaminated stations. The depleted faunal abundance pinpoints to environmental stress in this area. Pollution-tolerant species such as J. borchersi, C. torosa, L. ghardaqensis, G. triebeli, M. striata, A. reticulata, and to some extent, C. dimorpha are survivors in a contaminated system. On the other hand, the Ras Gharib site is less contaminated with metals, and the sediments are coarse-grained with low TOC%. Pollution-sensitive species such as X. rhomboidea are characterizing Ras Gharib area. The species composition of the polluted areas can be considered as a good proxy for environmental considerations.
Data availability
The datasets used and/or analyzed during the current study are included in the published article and its supplementary information files and are available from the corresponding author on reasonable request.
Change history
19 March 2021
A Correction to this paper has been published: https://doi.org/10.1007/s11356-021-13374-y
Abbreviations
- J. borchersi :
-
Jugosocythereis borchersi
- H. rubrimaris :
-
Hiltermannicythere rubrimaris
- L .ghard :
-
Loxocorniculum ghardaqensis
- M. striata :
-
Moosella striata
- G. triebeli :
-
Ghardaglaia triebeli
- X. rhomboidea :
-
Xestolebris rhomboidea
- X. ghardaqae :
-
Xestoleberis ghardaqae
- C. dimorpha :
-
Cytheroma dimorpha
- C. torosa :
-
Cyprideis torosa
- A. reticulata :
-
Alocopocythere reticulata.
References
Alin S, Cohen AS, Bills R, Mukwaya M, Michel M, Tiercelin K, Coveliers P, Keita S, West K, Soreghan M, Kimbadi S, Ntakimazi G (1999) Effects of landscape disturbance on animal communities in Lake Tanganika, East Africa. Conserv Biol 13:1017–1033
Anadon, P., Gliozzi, E., Mazini, I., 2002. Paleoenvironmental reconstruction of marginal marine environments from combined paleoecological and geochemical analysis on ostracods. In: Holmes, J.A., Chivas, A.R. (Eds.), the Ostracoda: Applications in Quaternary Research, Geophysical Monograph, 131. American Geophysical-Union, Washington, DC, pp. 227–247
Badr NBE, El-Fiky AA, Mostafa AR, Al-Mur BA (2009) Metal pollution records in core sediments of some Red Sea coastal areas, Kingdom of Saudi Arabia. Environmental Monitoring Assessment 155:509–526
Bergin F, Kucuksezgin F, Uluturhan E, Barut IF, Meric E, Avsar N, Nazik A (2006) The response of benthic foraminifera and ostracod to heavy metal pollution in Gulf of Izmir. Estuary, Coast. Shelf Science 66:368–386
Bodergat, A.M., Ikeya, N., 1988. Distribution of recent Ostracod in Ise and Mikawa Bays, Pacific coast of central Japan. In: Hanai, T., Ikeya, N., Ishizaki, K. (Eds.), Evolutionary biology of Ostracod- its fundamentals and applications. Kodansha, Tokyo, Elsevier, Amsterdam, pp. 413–428
Bonaduce, G., Ciliberto, B., Minichelli, G., Masoli, M., Pugliese, N., 1983. The Red Sea benthic ostracods and their geographical distribution. Proceedings of the 8th International Symposium on Ostracoda: Applications of Ostracoda, (R. F. Maddocks, Ed.) Univ. Houston Geoscience: 472–491
Boomer I, Eisenhauer G (2002) Ostracod faunas as palaeoenvironmental indicators in marginal marine environments. In: Holmes JA, Chivas AR (eds) The Ostracoda: applications in quaternary research, geophysical monograph, vol. 131. American Geophysical Union, Washington, DC, pp 135–149
Callender, E., 2005. Heavy metals in the environment-historical trends. In: Lollar, B. S. (Ed), Holland, H.D., Turekian, K.K. (Ex. Eds), Environmental Geochemistry. Treatise on Geochemistry, vol. 9, 612 p., Elsevier, Amsterdam
Carman KR, Fleeger JW, Pomarico SM (2000) Does historical exposure to hydrocarbon contamination alter the response of benthic communities to diesel contamination? Mar. Environ Res 49:255–278
Chivas AR, De Deckker P, Shelley JMG (1986) Magnesium and strontium in nonmarine ostracods shells as indicators of paleosalinity and paleotemperature. Hydrobiologia 143:135–142
Dean WE (1974) Determination of carbonate and organic matter in calcareous sediments and sedimentary rocks by loss in ignition: comparison with other methods. J Sediment Petrol 44:242–248
Diaz RJ, Rosenberg R (1995) Marine benthic hypoxia: a review of its ecological effects and the behavioral responses of benthic macrofauna. Oceanography Marine Biology Annual Review 33:245–303
Duchart P, Calvert S, Price N (1973) Distribution of trace metals in the pore waters of shallow marine sediments. Limnology and Oceanography 18:605–610
Eager SH (1999) Distribution of Ostracoda around a coastal sewer outfall: a case study from Wellington, New Zealand. Journal of Royal Society New Zealand 29:257–264
El-Kahawy R, El-Shafeiy M, Helal SA, Aboul-Ela N, El-Wahab MA (2018) Morphological deformities of benthic foraminifera in response to nearshore pollution of the Red Sea, Egypt. Environmental Monitoring and Assessment 190:312. https://doi.org/10.1007/s10661-018-6695-2
El-Komi, M. M., Emara A.M. and Mona, M.H., 2002. Hydrographical conditions and benthic assemblages in the Suez Gulf. Pakistan Journal of Marine Sciences, Vol. 11(1&2), 1–18, 2002
Elshanawany, R., 2010. Microfossil assemblages as proxies to reconstruct anthropogenic induced eutrophication of two marginal Eastern Mediterranean Basins. PhD thesis, University of Bremen, Germany
El-Sorogy, A.S., Abd El-Wahab, M., Nour, H.E., Ziko, A., Shehata, W., 2006. Faunal assemblages and sedimnet chemistryof some lagoons along the Red Sea coast, Egypt.Egypt. J. Paleontol. (6):193–224
El-Taher A, Madkour HA (2014) Environmental and radioecologicalstudies on shallowmarine sediments from harbourareas along the Red Sea coast of Egypt for identification ofanthropogenic impacts. Isotopes in Environmental andHealth Studies 50(1):120–133
Folk RL, Ward WC (1957) Brazos River bar: a study in the significance of grain size parameters. J Sediment Res 27(1):3–26
Frenzel P, Boomer I (2005) The use of ostracods from marginal marine, brackish waters as bioindicators of modern and quaternary environmental change. Palaeogeography., Palaeoclimatology, Palaeoecology 225:68–92
Gabrié C, Montaggioni L (1982) Sedimentary facies from the modern coral reefs, Jordan gulf of Aqaba a, Red Sea. Coral Reefs 1(2):115–124
Gross MG (1971) Carbon determination. In: Carver RE (ed) Procedures in sedimentary petrology. John Wiley and Sons, New York, pp 573–596
Hammer Ø, Harper DAT, Ryan PD (2009) PAST- Palaeontological Statistics. In: version 1, vol 89. University of Oslo, Oslo, pp 1–31
Hartmann G (1964) Neontological and paleontological classification of Ostracoda. Ostracods as ecological and palaeoecological indicators. Pubbl Stn Zool Napoli 33:550–587
Hegazy MN, Effat HA (2010) Monitoring some environmental impacts of oil industry on coastal zone using different remotely sensed data. Egypt J Remote Sens Space Sci 13(1):63–74
Helal S, Abd El-Wahab M (2004) Recent Ostracods from marine sediments of Safaga Bay, Red Sea, Egypt. Egypt Jour Paleontology 4:75–93
Helal, S., Abd El-Wahab, M., 2010. Zoogeography and taxonomy of the recent marine ostracod in the Egyptian Red Sea mangrove protected areas. Fifth international conference on the Geology of the Tethys Realm, South Valey. UNI: 55–70
Helal S, Abd El-Wahab M (2012) Distribution of podocopid ostracods in mangrove ecosystems along the Egyptian Red Sea coast. Crustaceana 85(14):1669–1696
Irizuki T, Takimoto A, Sako M, Nomura R, Kakuno K, Wanishi A, Kawano S (2011) The influences of various anthropogenic sources of deterioration on meiobenthos (Ostracod) over the last 100 years in Suo-Nada in the Seto Inland Sea, southwest. Japanese Marine Pollution Bulletin 62:2030–2041
Irizuki T, Ito H, Sako M, Yoshioka K, Kawano S, Nomura R, Tanaka Y (2015) Anthropogenic impacts on meiobenthic Ostracod (Crustacea) in the moderately polluted Kasado Bay, Seto Inland Sea, Japan, over the past 70 years. Mar Pollut Bull 91(1):149–159
Kemp J, Radke CL, Olley J, Juggins S, Deckker P (2012) Holocene lake salinity changes in the Wimmera, southeastern Australia, provide evidence for millennial-scale climate variability. Quat Res 77:65–76
Lee MR, Correa JA (2005) Effects of copper mine tailings disposal on littoral meiofaunal assemblages in the Atacama region of northern Chile. Mar Environ Res 59:1–18
Lenihan HS, Peterson CH, Kim SL, Conlan KE, Fairey R, McDonald C, Grabowski JH, Oliver JS (2003) Variation in marine benthic community composition allows discrimination of multiple stressors. Mar Ecol Prog Ser 261:63–73
Leps J, Smilauer P (2003) Multivariate analysis of ecological data using CANOCO. Cambridge University Press, New York 283 pp
Li Y-H (2000) A compendium of geochemistry: from solar nebula to the human brain. Princeton University Press, Princeton 475 pp
Lili F, Irizuki T, Sampei Y (2013) Spatial distribution of recent ostracod assemblages and depositional environments in Jakarta Bay, Indonesia, with relation to environmental factors. Paleontology Research 16:267–281
Liljenstroem, S., Widbom, B., Mattson, J., 1987. Effects of two oil refinery effluents on benthic Meiofauna in Mesocosms. Swedish Environmental Research Institute. 38 pp.
Lim RP, Wong MC (1986) The effects of pesticides on the population dynamics and production of Stenocypris major Baird (Ostracoda) in rice fields. Archive Hydrobiology 106:421–427
Long ER, MacDonald DD, Smith SL, Calder FD (1995) Incidence of adverse biological effects within ranges of chemical concentrations in marine and estuarine sediments. Environ Manag 19(1):81–97
Maddocks, R. F. 1985. One hundred million years of predation on Ostracods: the fossil record in Texas. In Hanai, T, lkeya, N, lshizaki, K.(eds.).1988. evolutionary biology of ostracoda its fundamentals and applications. Proceedings of the Ninth International Symposium on Ostracoda, held in Shizuoka, Japan, 29 July-2 August 1985, KODANSHA, Tokyo & ELSEVIER Amsterdam oxford- New York-Tokyo.1988: 637–685
Mansour A (1995) Sedimentary facies and carbonate-siliciclastic transition of Sharm El Bahari and Sharm El Qibli, Red Sea, Egypt. Egypt J Geol 39(1):57–76
Mansour, A. M., Nawar, A. H. and Mohamed, A. W., 2000. Geochemistry of coastal marine sediments and their contaminant metals, Red Sea, Egypt: a legacy for the future and a tracer to modern sediment dynamics. Sedimen. of Egypt,8: 231–242
Mansour AM, Nawar AH, Madkour HA (2011) Metal pollution in marine sediments of selected harbours andindustrial areas along the Red Sea coast of Egypt. Ann. Naturhist. Mus. Wien, Serie A 113:225–244
Mazzola A, Mirto S, Danovaro R (1999) Initial fish-farm impact on meiofaunal assemblages in coastal sediments of western Mediterranean. Mar Pollut Bull 38:1126–1133
Mette W, Roozbahani P (2012) Late Permian (Changsingian) ostracods of the Bellerophon Formation at Seis (Siusi) (Dolomites, Italy). J Micropalaeontol 31:73–87
Millward RN, Carman KR, Fleeger JW, Gambrell RP, Portier R (2004) Mixtures of metals and hydrocarbon elicit complex responses by a benthic invertebrate community. J Exp Mar Biol Ecol 310:115–130
Mirto S, La Rosa T, Danovaro R, Mazzola A (2000) Microbial and meiofaunal response to intensive mussel-farm biodeposition in coastal sediments of the western Mediterranean. Mar Pollut Bull 40:244–252
Mohammed M, Al-Wosabi M, Keyser D, Al-Kadasi WM (2012) Distribution and taxonomy of shallow marine Ostracods from northern Socotra Island (Indian Ocean)-Yemen. Rev Micropaleontol 55(4):149–170
Moore, R. C., 1961. Treatise on invertebrate paleontology, part Arthropoda, (Ostracod). Geological Society American University Kansas Press, 422 pp
Moore C, Harries D, Ware F (1997) The impact of the SeaEmpress oil spill on the sandy shore meiofauna of south west Wales. CCW Sea Empress Contract Report 230
Morcos SA (1970) Physical and chemical oceanography of the Red Sea. Oceanogr Mar Biol Ann Rev 8(73):202
Nour HE, Nouh E (2020) Comprehensive pollution monitoring of the Egyptian Red Sea coast by using the environmental indicators. Environ Sci Pollut Res 27:28813–28828
Orive, E., Elliott, M., de Jorge, V.V., 2002. Nutrients and eutrophication in estuaries and coastal waters, developments in hydrobiology 164. Kluwer Academic Publishers
Piller WE, Pervesler P (1989) The Northern Bay of Safaga (Red Sea, Egypt): an actuopaleontological approchs. I: topography and bottom. Facies.Beitr.Palantologie, Osterr 15:103–147
Pint A, Frenzel P (2017) Ostracod fauna associated with Cyprideis torosa–an overview. Journal of Micropalaeontology 36(1):113–119
Poquet JM, Mezquita F, Rueda J, Miracle AR (2008) Loss of Ostracod biodiversity in Western Mediterranean wetlands. Aquatic Conservation Marine and Freshwater Ecosystem 18:280–296
Rasul, N., Stewart, I,. Nawab, Z., 2015. Introduction to the Red Sea; its origin, structure, and environment. The Red Sea, 1–28
Ruiz, F., 1994. Los ostracodos del litoral de la provincia de Huelva. PhD thesis, Huelva University, 275 pp.
Ruiz F, Gonzalez-Regalado ML, Borrego J, Morales JA (1997) The response of ostracod assemblages to recent pollution and sedimentary processes in the Huelva estuary, SW Spain. Sci Total Environ 207:91–103
Ruiz F, Gonzalez-Regalado ML, Baceta JI, Muoz JM (2000) Comparative ecological analysis of the ostracod faunas from low- and high-polluted southwestern Spanish estuaries: a multivariate approach. Mar Micropaleontol 40:345–376
Ruiz F, Gonzlez-Regalado ML, Borrego J, Abad M, Pendón JG (2004) Ostracoda and foraminifera as short-term tracers of environmental changes in very polluted areas: the Odiel Estuary (SW Spain). Environ Pollution 129:49–61
Ruiz F, Abad M, Bodergat AM, Carbonel P, Rodríguez-Lázaro J, González-Regalado ML, Toscano A, García EX, Prenda J (2013) Freshwater ostracods as environmental tracers. International Journal Environmental Science Technology 10:1115–1128
Samir AM (2000) The response of benthic foraminifera and ostracods to various pollution sources: a study from two lagoons in Egypt. J Foraminifer Res 30(2):83–98
Shannon, C. E., and Weaver, W., 1948. A mathematical theory of communication. Bell Syst. Tech. J. (27): 379–423 and 623–656
Steichen DJ, Holbrook SJ, Osenberg CW (1996) Distribution and abundance of benthic and demersal macrofauna within a natural hydrocarbon seep. Mar. Ecol. Prog Ser 138:71–82
Suguio K (1973) Introdução à Sedimentologia. Edgard Blucher, São Paulo 317p
USGS. 1995 Available from: http://minerals.cr.usgs.gov/geo_chem_stand/marine.html
Van der Merwe, C., 2003. The assessment of the influence of treated underground mine water on the benthic fauna in a portion of the Blesbokspruit Ramsar Site. Mini-thesis for Magister Artium in Environmental Management. Rand Afrikaans University, South Africa
Watzin MC, Roscigno PR (1997) The effects of zinc contamination on the recruitment and early survival of benthic invertebrates in an estuary. Mar Pollut Bull 34:443–455
Yasuhara M, Yamazaki H, Irizuki T, Yoshikawa S (2003) Temporal changes of ostracode assemblages and anthropogenic pollution during the last 100 years, in sediment cores from Hiroshima bay, Japan. Holocene 13:527–536
Yasuhara M, Hunt G, Breitburg D, Tsujimoto A, Katsuki K (2012) Human induced marine ecological degradation: micropaleontological perspectives. Ecology and Evolution 2:3242–3268
Youssef M, Madkour H, Mansour A, Alharbi W, El-Taher A (2017) Invertebrate shells (mollusca, foraminifera) as pollution indicators, Red Sea Coast, Egypt. J Afr Earth Sci 133:74–85
Acknowledgments
The authors express their gratitude to the Cairo University for funding the chemical analyses. We are grateful to the editor Dr. Sarma VVSS and the two anonymous reviewers for considering the manuscript and help evolving it.
Funding
Partial financial support was received from Cairo University for the geochemical analysis.
Author information
Authors and Affiliations
Contributions
Nabil Aboul-Ela, Sobhi Helal, and M. Abd El-Wahab proposed and designed the research study. Ramadan El-Kahawy contributed to the acquisition of data. R. El-Kahawy, M. El-Shafeiy, and Sobhi Helal analyzed the data and wrote the manuscript. All authors approved the final version of the manuscript.
Corresponding author
Ethics declarations
Ethical approval
Not applicable.
Consent to participate
Not applicable.
Consent to publish
Not applicable.
Competing interests
The authors declare that they have no competing interests.
Additional information
Responsible editor: Vedula VSS Sarma
Publisher’s note
Springer Nature remains neutral with regard to jurisdictional claims in published maps and institutional affiliations.
The original online version of this article was revised: The image of Figure 9, citations of Figures 7, 8, 9 and 10 and the captions of Figures 9 and 10 are modified in this paper.
Supplementary information
ESM 1
Species distribution chart showing the ostracods density at the two studied sites. (PNG 1806 kb)
ESM 2
Percentage of all species, genera, and families collected from the two sampling sites (Ras Gharib and Quseir). (PNG 1908 kb)
ESM 3
1–3: Jugosocythereis borchersi (Hartmann 1964), Sample no. Q.2; 4: Paranesidean. sp2. Bonaduce, Ciliberto, Minichelli, Masoli & Pugliese, 1983, Sample no. Q.5; 5–6: Paranesidea fracticorallicola Maddocks, 1969, Sample no. Q.2; 7–8: Neonesidea schulzi (Hartmann 1964), Sample no. Q.6; 9: Triebelina sertata Triebel, 1948, Sample no. G.8; 10: Cytherelloidea n. sp1. Bonaduce, Ciliberto, Minichelli, Masoli & Pugliese1983, Sample no. Q.3; 11: Hiltermannicythere rubrimaris (Hartmann 1964), Sample no. Q.7; 12-Moosella striata Hartmann 1964, Sample no. G.8; 13: Caudites levis Hartmann 1964, Sample no. Q.9; 14: Alocopocythere reticulata (Hartmann 1964), Sample no. Q.1; 15: Cyprideis torosa, (Jones, 1850), Sample no. Q.2 (PNG 1.05 mb)
ESM 4
1: Bishopina cf. spinulosa (Brady, 1868), Sample no. Q.3; 2: Loxoconcha gurneyi Bate & Gurney, 1981, Sample no. G.9; 3: Loxocorniculum ghardaqensis (Hartmann 1964), Sample no. Q.2; 4- Sclerochilus rectomarginatus Hartmann 1964, Sample no. Q.6; 5: Hemicytherura videns (Müller, 1894), Sample no. Q.5; 6: Chartocythere arenicola (Hartmann 1964), Sample no. G.8; 7: Ghardaglaia triebeli Hartmann 1964, Sample no. Q.2; 8: Cytheroma dimorpha Hartmann 1964, Sample no. Q.7; 9: Paradoxostoma breve Müller, 1894, Sample no. Q.6; 10: Paradoxostoma parabreve Hartmann 1964, Sample no. Q.9; 11: Xestoleberis ghardaqae. Hartmann 1964, Sample no. Q.2; 12: Xestoleberis rhomboidea Hartmann 1964, Sample no. Q.3 (PNG 802 kb)
Rights and permissions
About this article
Cite this article
El-Kahawy, R., El-Shafeiy, M., Helal, S. et al. Benthic ostracods (crustacean) as a nearshore pollution bio-monitor: examples from the Red Sea Coast of Egypt. Environ Sci Pollut Res 28, 31975–31993 (2021). https://doi.org/10.1007/s11356-020-12266-x
Received:
Accepted:
Published:
Issue Date:
DOI: https://doi.org/10.1007/s11356-020-12266-x