Abstract
Human activities, particularly in large cities, can lead to pollution caused by micropollutants such as pesticides in water bodies, which have been recognized as serious threats to the environment and human health. The pollution level of six organophosphorus pesticides, three herbicides, and one bactericide in groundwater and the Wenyu River, and their fates in three sewage treatment plants (STPs) and a hospital were investigated in this study. The concentrations of the ten detected pesticides ranged from not detected (ND) to 323.44 ng L−1 in different water samples from Beijing; metalaxyl was detected to have the highest concentration (89.58 ng L−1), and the detection frequencies of atrazine and metalaxyl were 100%. The maximum concentrations of pesticides in the Wenyu River, STPs, and the hospital were 1–2 orders of magnitude higher than those in the groundwater. Good removal efficiencies by the treatment processes were observed for ametryn (100%), while the removal efficiencies for atrazine and omethoate were the lowest in the three STPs (− 9.6% and 12.67%, respectively). Finally, risk quotient (RQ) values of each contaminant were estimated from the maximum values determined for typical urban to assess the ecology and health effects. In the case of environmental toxicity, the highest RQ values (> 1) were obtained for dichlorvos and omethoate. In the case of health toxicity, the RQ values show that the pesticides found in groundwater pose no potential health risks to humans at current concentrations.
Similar content being viewed by others
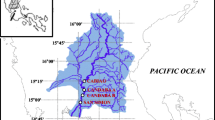
Explore related subjects
Discover the latest articles, news and stories from top researchers in related subjects.Avoid common mistakes on your manuscript.
Introduction
Organophosphorus pesticides (OPs) are one of the earliest synthetic pesticides produced by humans and are still widely used as efficient insecticides and plant growth regulators in agricultural production at home and abroad (Tsygankov 2019). OPs have the advantages of high efficacy, low cost, low residual toxicity, and easy degradation. Compared with organochlorine and pyrethroid pesticides, insect resistance to OPs has gradually increased. In 1991, 1994, and 1996, the sales of OPs reached 3.317 × 109, 3.017 × 109, and 3.31 × 109 US dollars, accounting for 39%, 38%, and 37% of the total sales of pesticides, respectively (Zhang and Sun 1999). In 1986, China produced 13,460 t of pesticides, of which 11,030 t was OPs, accounting for more than half of the total pesticides produced (Hua and Shan 1999). According to the statistics of China’s Ministry of Chemical Industry, in 1997, the output of OPs in China was approximately 200,000 t, accounting for ~ 57% of the total pesticides produced, while organophosphate insecticides accounted for ~ 50% of the total pesticides (Tang et al. 2004). Since the total pesticide output of China exceeded 1 × 106 t per year in 2005, China’s annual output of pesticides has ranked first in the world and its usage area ranks second in the world (Xu et al. 2019). Over 500 × 106 lb. of herbicides, insecticides, and fungicides was used annually from 1992 to 2011 (Stone et al. 2014). The widespread use of OPs has greatly contributed to the development of agriculture. However, it has also caused OPs to become common environmental pollutants; thus, OPs seriously affect food, environmental, and ecological security, and are a threat to human health (Berman et al. 2011). In particular, highly toxic OPs are more likely to pollute agricultural products and poison the consumers. The World Health Organization statistics on the use of pesticides in 19 countries show that around 500,000 acute poisoning incidents caused by pesticides occur worldwide each year, of which approximately 40,000 people die. Thus, with the recognition of the major environmental problems caused by organic pollution, increasing attention is being paid to organic micro-pollutants in surface, underground, and coastal watersheds (Affum et al. 2018; Chen et al. 2016).
Metropolises like Beijing have particularly high population densities, which may lead to a high probability of pesticide exposure to citizens. According to the statistics, at the end of 2016, the permanent population of Beijing was 21.73 × 106 and the water consumption was 3.95 × 109 m3, of which surface water and groundwater accounted for 9.1% and 42%, respectively (Beijing Statistical Yearbook 2018). Therefore, for a densely populated and water-short city like Beijing, OPs are likely to become a potential health risk. However, most of the studies on OPs in China concentrated on rivers and lakes; only a few focused on water bodies in densely populated urban areas with a large number of daily living and production activities. Based on this, the concentrations of OPs in typical urban water bodies of Beijing were investigated. The occurrence of six OPs, three herbicides, and one bactericide was characterized in this study. In addition, an ecological risk assessment on the Wenyu River, STPs, and groundwater, and a health risk assessment on groundwater were conducted to obtain a good overview of the water pollution risks associated with pesticides in Beijing. The results will be helpful to understand the pollution level of pesticides in the typical urban water of international modern metropolises, promote the construction of a security system to mitigate the impact of pesticides on the environment and human health, and provide support data for the future management and control of OPs.
Materials and methods
Sampling sites
The seven sampling sites of surface water in the Wenyu River, W1-W7, were as follows: Shahe floodgate, Mafang bridge, Xisishang village, Wenyu bridge, Yigezhuang bridge, Wenyu River bridge, and Beiguan floodgate. According to the water flow, five samples of groundwater were obtained from a site in Shunyi District, and three samples from STPs in Miyun District, Haidian District, and Chaoyang District (M, H, and C, respectively) including influent (M1, H1, and C1), secondary effluents (M2 and H2), and effluents (M3, H3, and C2). The groundwater was collected from five wells (G1, G2, G4, and G5 are 29 m deep; G3 is a multilevel monitoring well with depths of 6.79 m, 11.64 m, and 22.53 m) located in Shunyi, and the influent (Y1) and effluent (Y2) from a hospital in Haidian District were collected once to investigate the OP pollution in winter. Detailed information on the Wenyu River, wells, STPs, and hospital are provided in Fig. 1 and Tables S1, S2. The water samples were collected three bottles at each sampling point and were saved in the brown glass bottle at 4 °C before laboratory analysis, which were treated within 24 h after being transported to the laboratory to detect the concentration of OPs and general wastewater quality parameters.
Reagent
All solvents (HPLC-grade) were supplied from Beijing Chemical Reagents Company (Beijing, China). The pesticide standards of the ten OPs (isocarbophos, phosmet, parathion methyl, triazophos, fonofos, and phoxim) were obtained from ANPEL Laboratory Technologies Inc. (Shanghai, China).
Instruments and quantification
The analysis of the OPs was conducted using high-performance liquid chromatography coupled with electrospray ionization tandem mass spectrometry (HPLC-MS/MS 8040; Shimazu, Japan), operated in positive or negative mode and equipped with an Inert Suatain C18 column (150 mm × 4.6 mm, 5 μm, Tokyo, Japan). The column was maintained at 40 °C during the sample analysis. The mobile phase comprised eluent A (methanol, 80%) and eluent B (ultrapure water, 20%). Separation of the OPs was achieved with the following gradient program: 0–2 min, 10% B; 2–15 min, 10%–100% B; 15–17 min, 100% B; 17–17.5 min, 100%–10% B; 17.5–20 min, 10% B. Quantification with an external standard was used to determine the concentration of OPs. The calibration curves for the detection of OPs exhibited good linear relationships (R2 > 0.99). The limit of quantification, calculated with a signal/noise ratio of 10, was 0.04–0.52 ng L−1.
Sample preparation
A filtered water sample was transferred to the separatory funnel, and 50 mL of dichloromethane was added. The sample was extracted by shaking the funnel for 5 min with periodic venting to release excess pressure (for further details, refer to ‘Method 622: The Determination of Organophosphorus Pesticides in Municipal and Industrial Wastewater’ (Pressley 1982) and ‘Water quality--Determination of organic phosphorous pesticide in water (Water Quality GB 13192-1991).
General wastewater quality parameters
The parameters of DO, pH, and temperature were monitored by a YSI automatic water quality detector. The chemical oxygen demand (COD), total nitrogen (TN), total phosphorous (TP), NH3-N, and NO3-N were determined according to the Ministry of Environmental Protection: Standard methods for the examination of water and waste water, MEP of China (2002).
Risk assessment for pesticides
Ecological risk assessment
An ecological risk assessment of the pesticides was performed based on the risk quotient (RQ) index method. The RQ is established based on Eq. (1).
where MEC is the maximum measured environmental concentration, and PNEC of the pesticides was calculated by the no-observed-effect concentration (NOEC) value and the acute or chronic aquatic toxicity, dividing the lowest short-term L(E)C50 or long-term NOEC, respectively, by an assessment factor (AF). In particular, AF was obtained from Papadakis et al. (2015). Moreover, in this ecological risk assessment, three trophic levels (algae, aquatic invertebrates, and fish) and the most sensitive species were used to determine the PNEC. The ecotoxicological data were obtained from the USEPA ECOTOX database (Table S3).
The risk ratios were classified into four risk levels: negligible risk (RQ < 0.01), low risk (0.01 < RQ < 0.1), medium risk (0.1 < RQ < 1), and high risk (RQ > 1) (Papadakis et al. 2015; Vryzas et al. 2009; Palma et al. 2014).
Health risk assessment
Health risk assessment is the process of predicting the probable effects of pollutants on human health over a specified period (Hu et al. 2011). As in previous studies (Derbalah et al. 2018; USEPA 2010; Papadakis et al. 2015), noncarcinogenic risks to humans were estimated using the hazard quotient (HQ). The HQ values were calculated assuming that surface water (as drinking water) is ingested directly (Hu et al. 2011; Papadakis et al. 2015).
To estimate the noncarcinogenic risk, the HQ was calculated using Eq. (3):
where RfD (mg kg−1 per day) is the acceptable daily intake of the contaminant via oral exposure. The values of the reference dose for the investigated pesticides were obtained from the USEPA Integrated Risk Information System (USEPA 2010), as in a previous publication.
The CDI for each pesticide was calculated using Eq. (4):
where C (mg L−1) is the measured concentration of a pesticide in water, IR is the water ingestion rate (0.87 L/day for a 6-year-old child, 1.41 L/day for a 70-year-old adult), EF is the exposure frequency (365 days/year), ED is the exposure duration (for children: ED = 6; for adults: ED = 70), BW is the body weight (20 kg for a child, 70 kg for an adult), and AT is the average lifespan (2190 days for a child; 25,550 days for an adult) (USEPA 2010).
HQ > 1 was considered to indicate that adverse effects could occur, while HQ < 1 indicates that adverse effects are improbable (Papadakis et al. 2015).
Statistical analysis
The correlation analysis of the OPs and environmental factors was conducted using SPSS 20.0. The correlation analysis of the OPs and environmental factors was evaluated by Pearson, which was considered significant at p < 0.05.
Results and discussion
Occurrence of pesticides in different waters of Beijing
Seven pesticides were detected in different water sources of Beijing among the ten targets, while the other 3 OPs were lower than the detection limits because of either their easily decomposed or lower usage amounts. The concentrations of the seven pesticides in different water samples from Beijing are shown in Table 1. The levels of the screened pesticides ranged from undetected (ND) to 323.44 ng L−1 in the different water sources from Beijing. The maximum concentrations of detected pesticides in groundwater were 1–2 orders of magnitude lower than those in the surface water of the Wenyu River, STPs, and the hospital. However, in cities with high population densities, the presence of pesticides in the groundwater, which is a source of drinking water, for a long time, can impact human health directly. Although different pesticides have different distributions, the majority of studies have shown a predominant presence of omethoate, metalaxyl, and atrazine in most water bodies (Meftaul et al. 2019). Overall, the results showed that metalaxyl and atrazine were the predominant pesticides with a detection frequency of 100% and that the detection rate of omethoate was greater than 80%. This can be attributed to their high consumption, stable structure (Liu et al. 2018), and longer half-life (e.g. atrazine = 60–150 days, metalaxyl = 115 days (pH = 9), omethoate = 68 days (pH = 7)) in the aquatic environment (Glinski et al. 2018). In the hospital and STP samples, low pollution levels of dichlorvos and diazinon were observed, which might be related to their usage and type; dichlorvos decomposes easily in the environment and hydrolyses by 50% at 30 °C for 18 days, while diazinon has high solubility and mobility in water (Cruz-Alcalde et al. 2018).
Compared with other pesticides in Wenyu River, dichlorvos had the highest concentration, ranging from not detected (ND) to 37 ng L−1, a little higher than the average level in the northern region (3–13 ng L−1) (Mijangos et al. 2018). Dichlorvos is one of the most widely used insecticides in developing countries, with concentrations ranging from 1.4 to 5630 ng L−1 in surface waters worldwide (Cruz-Alcalde et al. 2018). However, dichlorvos has a high vapour pressure; hence, it easily enters the atmosphere. The transportation and diffusion of dichlorvos mainly occur through the atmosphere and water. Furthermore, it has a greater water solubility and smaller logKow (1.47), implying that it can be easily decomposed and removed (Wu et al. 2015). The above results correspond to the occurrence of dichlorvos in the STPs of Chaoyang and Miyun Districts. Metalaxyl and atrazine have mean concentrations of 6.67 and 4.31 ng L−1, respectively, in the Wenyu River, which are higher than the OP concentrations. The solubility of metalaxyl in water was 0.71% (20 °C), and it was stable in acidic and neutral media. The chemical hydrolysis of atrazine in water is stronger than the biodegradation, and the pH value has a strong influence on the hydrolysis process; i.e. it is fast under acidic or alkaline conditions (Qu et al. 2020). The frequencies and concentrations of these pesticides were similar to those in most rivers or lakes in China, mostly because of their high chemical stability and consumption (Elfikrie et al. 2020). In the Wenyu River, the concentrations of omethoate, metalaxyl, and atrazine have no apparent spatial distribution characteristics. However, the concentration of propazine gradually decreases along the flow direction in the Wenyu River, from 10.586 to 0.507 ng L−1, and there is no propazine pollutant source in the lower part of the Wenyu River. This may indicate that propazine degrades naturally in the environment via photodegradation and biodegradation (Qu et al. 2011). The accumulative concentration in all the sites in the Wenyu River ranged from 14.85 to 56.54 ng L−1; the highest concentration was observed in W6 with dichlorvos contributing 64.58%, followed by W1 > W7 > W2 > W4 > W5 > W3. Many factors influence the spatial distribution of pesticide pollution in the sampling sites, such as the effects of degradation and dilution, which can lead to their low levels in surface water. Therefore, further investigation is required with regard to these factors.
The concentrations of pesticides in groundwater were 1–2 orders of magnitude lower than those in the Wenyu River. The migration of pesticides was hindered by the natural soil infiltration layer, which can remove most pesticides through rainfall, irrigation, and other human activities, and result in low residues. The highest concentration of omethoate was detected in groundwater, particularly in the G1, G3, and G5 samples, presenting an average concentration of 3.09 ng L−1, and accounting for approximately 61.90% of all the pesticides detected in groundwater. After entering the environment, omethoate cannot be easily degraded by ordinary microorganisms (Hu et al. 2011). Previous results have shown that there are two main ways for microorganisms to degrade pesticides: mineralization and co-metabolism (Lazarević-Pašti et al. 2016). Surprisingly, no omethoate was detected at the middle position, but it was detected at the low position, which may be related to the migration and transformation of pesticides in soil; the migration of pesticides may be hindered by the natural soil infiltration layer. Omethoate has a strong contact killing effect on pests and mites, particularly on some aphids, which are resistant to dimethoate. Additionally, it has high toxicity and can maintain a strong toxicity at low temperature, and enters the human body directly through drinking water, which could pose a potential threat to ecological environment and human health. The acceleration of the energy utilization process and unreasonable exploitation of groundwater are significant factors causing the accumulation of pesticide residues (Lee et al. 2019). In addition, the atmospheric precipitation and groundwater infiltration may be another cause of OP pollution in groundwater (Mauffret et al. 2017).
Atrazine, metalaxyl, and omethoate have high-frequency detection in the STPs and hospital. The dichlorvos influent concentrations of STPs in Chaoyang and Miyun Districts were 22.26 ng L−1 and 5.90 ng L−1 respectively, which are clearly higher than those of the other pesticides. However, it was not detected in the effluent, which illustrates that these STPs have a distinct removal efficiency for dichlorvos (> 99%). These results are similar to those from the STPs in Stonecutter Island and Shatin (Man et al. 2018) and Catalonia (Köck-Schulmeyer et al. 2013).
The above results indicate that the river surface is more vulnerable to pollution, not only because the Wenyu River has a wide range of input contaminants and extensive pollution sources compared with STPs and the hospital, such as surface runoff and wet-dry deposition which facilitate the transport of pesticides from the pollutant source to the waterway, but also because there is no natural barrier, such as the adsorption and filter interception of soil, compared with groundwater.
Removal efficiency of pesticides in STPs and hospital
The removal efficiencies of the detected pesticides in STPs and hospital were calculated according to the following:
The influent OP concentration was the OP concentration of sample point of M1, H1, C1, and Y1, respectively. The effluent OP concentration was the OP concentration of sample point of M2, M3; H2, H3; and C2 and Y2, respectively.
From the results, the removal efficiency of the detected pesticides ranged from − 2 to 8% in the hospital, and they are not the main contaminants in hospital wastewater; thus, the removal efficiency of pesticides by the existing hospital sewage treatment technology may be relatively poor. In addition, chlorination is the only wastewater treatment process in the hospital, which limits the removal efficiency for most pesticides in hospital wastewater treatment.
The detected pesticide elimination rates for different STPs varied significantly for different STPs, which depends on many factors, such as the different treatment processes, pollution load, or hydraulic residence time. From the Table 2, it is clear that the STP in Miyun District has a higher removal efficiency for detected pesticides (ametryn (80%), metalaxyl (36%), and dichlorvos (100%) compared with other STPs. However, it has a poor removal efficiency for omethoate, which explains why the sewage disposal system had no significant effect on the pollution caused by omethoate, and the omethoate may translate into its parent (Wang et al. 2018) in the process of wastewater treatment. Thus, these results provide good insights into the mechanism of the mutual transition between metabolites and the parents of some pesticides. Moreover, considerable amounts of ametryn and dichlorvos were removed in the STP in Chaoyang District, reflecting that these two pesticides are susceptible to hydrolysis, particularly under alkaline conditions (Mahesh and Manu 2019) or adsorption (abiotic) onto activated sludge.
Table 2 shows that the worst removal capabilities of the detected pesticides were found at the STP in Haidian District, where most detected pesticides had zero or negative values. This illustrates that although A/O+MBR technology has a stronger purification capacity for many other organic pollutants, such as antibiotics (Liu et al. 2019), it is influenced by the external environment, such as pollution load, environment temperature, and maintenance of treatment equipment, and the characteristics of the pollutants themselves; for example, unlike ametryn and dichlorvos, atrazine and propazine were not easily removed. The effluent concentrations of atrazine and propazine gradually increased with further treatment. Some studies have confirmed that atrazine is water-soluble and has strong stability in weak acid or base water (Hansen et al. 2019). Propazine is nonbiodegradable, which results in the secondary release of propazine from sludge to water due to changes in the external environment during the treatment process (Köck-Schulmeyer et al. 2013), demonstrating that the biological and chemical methods are not as effective for the removal of propazine as expected (Köck-Schulmeyer et al. 2013). Furthermore, previous works regarding the occurrence and removal of pesticides in STPs also indicate that concentrations in effluents are frequently higher than those in influents, which show a poor efficiency and high variability on pesticides (Campo et al. 2013). Compared with cyclic activated sludge technology (CAST) (STP in Chaoyang District) and A/O+MBR technology (STP in Haidian District), A2/O technology (STP in Miyun District) seems to be more effective for the removal of pesticides. The performance of the studied STP in Haidian District for the removal of atrazine was similar to that reported from an activated sludge plant in Northeastern Spain, where tertiary treatment in WWTP-A is based on coagulation, flocculation, chlorination, and microfiltration.
Correlation analysis between pesticides and physicochemical parameters
To study the influence of environmental factors on the content of pesticides, we conducted a correlation analysis between the pesticides and environmental factors, such as the TP, TN, NH4+-N, NO3−-N, and COD. The results are shown in Table 3.
As demonstrated, the occurrence concentration of ametryn and diazinon had the significant correlation with concentration of ammonia nitrogen, phosphorus, and organic matter in water (P < 0.05), while the occurrence of NO3−-N and TN did not affect the migration and transformation of ametryn and diazinon. Besides, metalaxyl, omethoate, and propazine also had the significant correlation with concentration of COD in typical water bodies. Furthermore, atrazine and dichlorvos have almost no correlation with the concentration of conventional environmental pollutants; according to Kumar et al. (2018), the degradation of them may be affected by pH as well as temperature, particularly regarding the time and intensity of illumination.
Degradation and the resulting reduced mobility of organophosphate pesticides in water are significant factors affecting their behaviours in nature. The removal of most organophosphate pesticides in the natural environment mainly depends on microbial degradation, in which they are degraded by microorganisms for their limiting nutrients, especially carbon source (Evangelista et al. 2010). Some researchers (Ali et al. 2019; Masís-Mora et al. 2019; Kumar et al. 2018; Liu et al. 2019) found that the carbon source and the concentrations of nitrogen and phosphorus impact microbial life activities, further affecting the removal efficiency of contaminants. Furthermore, at P-starved conditions, OPs can be used as an alternative phosphorus source for marine plankton and microbes (Liu et al. 2019). This may be an important reason for ametryn related with TP in the Wenyu River.
Ecological risk assessment
The ecological risk assessment for the pesticides detected was performed based on the RQ method (Kapsi et al. 2019). This approach provides an estimate of the contribution of the compound of interest to the total toxicity of the water sample analysed to a certain taxonomic group (Table S2). The Pesticide Properties Database (Lewis et al. 2016) was used for the toxicological endpoint due to its comprehensive data on algae, aquatic invertebrates, and fish toxicity, and because it covers an extensive number of compounds. The MEC and PNEC values are presented in Table S2 and the RQs corresponding to the maximum concentrations (RQmax) for every pesticide in the typical water bodies of Beijing are presented in Fig. 2.
The RQ values of ametryn, dichlorvos, and omethoate were higher than those of the other pesticides in this investigation, particularly in the Wenyu River. Dichlorvos poses a high risk (RQ > 1) in the Wenyu River, the STPs of Chaoyang and Miyun Districts. Similarly, omethoate poses a medium risk at all sampling points, while ametryn poses a medium risk in the Wenyu River (RQ > 0.1). However, omethoate poses no risk to the ecological environment as per the study by Triassi et al. (2019). Meanwhile, atrazine, diazinon, metalaxyl, and propazine all pose negligible risk (RQ < 0.01). Compared with coastal waters around Liaodong Peninsula, as an herbicide known to effect algae, atrazine poses a high risk (RQ > 1) to algae (Xie et al. 2019); the same was observed in Taihu Lake wetland, China (Qu et al. 2011).
Based on the above analysis, the pesticides in the Wenyu River have a greater impact on the ecological environment, while those in groundwater and the hospital have low impact on the surrounding ecological environment. Furthermore, hospitals are places with high population densities, more pathogens, and strong viral infectivity. The concentration of omethoate in hospital sewage discharge is high; thus, it is a potential hazard to the surrounding environment (Xu et al. 2019), such as Kunming lake and groundwater. Additionally, the source of pesticide pollution should be identified and the pesticide discharge should be controlled. Therefore, further investigation on the risk assessment of mixed pesticides to aquatic species and human health is urgently required.
Health risk assessment
In many rural areas of China, shallow groundwater serves as a direct drinking water source. With the development of the agricultural economy and the wider use of agricultural chemicals, the risk of pesticide pollution in groundwater is increasing (Yang et al. 2017). Hence, a health risk assessment for the groundwater of the contaminated sites is necessary. In this study, typical pesticides detected with high values in the sampling points were selected and used for the noncarcinogenic health risk assessment model. The noncarcinogenic risks of ametryn, atrazine, diazinon, dichlorvos, metalaxyl, omethoate, and propazine are shown in Table 4.
When the noncarcinogenic risk value is lower than 1, there is no apparent danger to human health (Yang et al. 2017). As shown in Table 4, the noncarcinogenic risk values of omethoate in groundwater to children and adults are 3.488E-03 and 2.20E-01, respectively, while the total risk was 0.2. Thus, the total risk is lower than 1, indicating that there is no apparent danger to human health. The overall noncarcinogenic risks to children and adults decreased in the following order: omethoate > atrazine > metalaxyl > propazine > ametryn of groundwater. The HQs for children ranged from 3.4888E-03 to 1.59E-07 and from 2.20E-01 to 1.004E-05 for adults. By definition, the noncarcinogenic risks for the individual pesticides were lower than the total noncarcinogenic risks. Therefore, each pesticide poses insignificant to no potential health risks to humans at the concentrations found in the assessed groundwater. The results also indicate that the noncarcinogenic risks of ametryn and propazine were much lower than those of atrazine and omethoate. Therefore, omethoate was the chief contaminant at this site and more attention should be paid to the remediation of omethoate in the future. Our results are in agreement with those of Xie et al. (2019) and Papadakis et al. (2015).
Conclusions
This paper reported the occurrence of pesticides in the surface water of the Wenyu River, groundwater, and hospital samples, as well as their fates in STPs in Beijing. An analysis showed that atrazine, dichlorvos, and metalaxyl were detected in all water body samples, typically ranging from a few ng/L to hundreds of ng/L. Compared with CAST technology (STP in Chaoyang District) and A/O technology (STP in Haidian District), A2/O technology seems to be more effective for the removal of detected pesticides, except omethoate. Furthermore, the ecological risk assessment revealed that dichlorvos and omethoate could pose a threat to the aquatic life, particularly dichlorvos in the three water bodies. Taking this into consideration, the sources of pesticides and the potential risk posed by mixed pesticides to the aquatic ecosystem and human health in densely populated cities require further investigation. The results of this study can provide some help for water resource managers and regulatory issues, such as monitoring, control, and management of organophosphorus pesticides in the future.
References
Affum AO, Acquaah SO, Osae SD, Kwaansa-Ansah EE (2018) Distribution and risk assessment of banned and other current-use pesticides in surface and groundwaters consumed in an agricultural catchment dominated by cocoa crops in the Ankobra Basin, Ghana. Sci Total Environ 633:630–640
Ali N, Khan S, Li Y, Zheng N, Yao H (2019) Influence of biochars on the accessibility of organochlorine pesticides and microbial community in contaminated soils. Sci Total Environ 647:551–560
Beijing Statistical Yearbook (2018) China Statistics Press
Berman T, Hochner-Celnikier D, Barr DB, Needham LL, Amitai Y, Wormser U, Richter E (2011) Pesticide exposure among pregnant women in Jerusalem, Israel: results of a pilot study. Environ Int 37(1):198–203
Campo J, Masiá A, Blasco C, Picó Y (2013) Occurrence and removal efficiency of pesticides in sewage treatment plants of four Mediterranean River basins. J Hazard Mater 263:146–157
Chen H, Zhu J, Li Z, Chen A, Zhang Q (2016) The occurrence and risk assessment of five organophosphorus pesticides in river water from Shangyu, China. Environ Monit Assess 188(11):614
Cruz-Alcalde A, Sans C, Esplugas S (2018) Priority pesticide dichlorvos removal from water by ozonation process: reactivity, transformation products and associated toxicity. Sep Purif Technol 192:123–129
Derbalah A, Chidya R, Jadoon W, Sakugawa H (2018) Temporal trends in organophosphorus pesticides use and concentrations in river water in Japan, and risk assessment. J Environ Sci 79:135–152
Elfikrie N, Ho YB, Zaidon SZ, Juahir H, Tan ESS (2020) Occurrence of pesticides in surface water, pesticides removal efficiency in drinking water treatment plant and potential health risk to consumers in Tengi River basin, Malaysia. Sci Total Environ 712:136540
Evangelista S, Cooper DG, Yargeau V (2010) The effect of structure and a secondary carbon source on the microbial degradation of chlorophenoxy acids. Chemosphere 79(11):1084–1088
Glinski DA, Purucker ST, Van Meter RJ, Black MC, Henderson WM (2018) Analysis of pesticides in surface water, stemflow, and throughfall in an agricultural area in South Georgia, USA. Chemosphere 209:496–507
Hansen SP, Messer TL, Mittelstet AR (2019) Mitigating the risk of atrazine exposure: identifying hot spots and hot times in surface waters across Nebraska, USA. J Environ Manag 250:109424
Hu Y, Qi S, Zhang J, Tan L, Zhang J, Wang Y, Yuan D (2011) Assessment of organochlorine pesticides contamination in underground rivers in Chongqing, Southwest China. J Geochem Explor 111(1–2):47–55
Hua XM, Shan ZJ (1999) Production, usage and environmental influence factors of pesticides in China. Sci Technol Anhui (in Chinese) 6:6–10
Kapsi M, Tsoutsi C, Paschalidou A, Albanis T (2019) Environmental monitoring and risk assessment of pesticide residues in surface waters of the Louros River (NW Greece). Sci Total Environ 650:2188–2198
Köck-Schulmeyer M, Villagrasa M, de Alda ML, Céspedes-Sánchez R, Ventura F, Barceló D (2013) Occurrence and behavior of pesticides in wastewater treatment plants and their environmental impact. Sci Total Environ 458:466–476
Kumar S, Kaushik G, Dar MA, Nimesh S, Lopez-Chuken UJ, Villarreal-Chiu JF (2018) Microbial degradation of organophosphate pesticides: a review. Pedosphere 28(2):190–208
Lazarević-Pašti TD, Pašti IA, Jokić B, Babić BM, Vasić VM (2016) Heteroatom-doped mesoporous carbons as efficient adsorbents for removal of dimethoate and omethoate from water. RSC Adv 6(67):62128–62139
Lee HJ, Kim KY, Hamm SY, Kim M, Kim HK, Oh JE (2019) Occurrence and distribution of pharmaceutical and personal care products, artificial sweeteners, and pesticides in groundwater from an agricultural area in Korea. Sci Total Environ 659:168–176
Lewis KA, Tzilivakis J, Warner DJ, Green A (2016) An international database for pesticide risk assessments and management. Hum Ecol Risk Assess: An International Journal 22(4):1050–1064
Liu T, Xu S, Lu S, Qin P, Bi B, Ding H et al (2018) A review on removal of organophosphorus pesticides in constructed wetland: performance, mechanism and influencing factors. Sci Total Environ 651:2247–2268
Liu X, Zhang G, Liu Y, Lu S, Qin P, Guo X et al (2019) Occurrence and fate of antibiotics and antibiotic resistance genes in typical urban water of Beijing, China. Environ Pollut 246:163–173
Mahesh GB, Manu B (2019) Removal of ametryn and organic matter from wastewater using sequential anaerobic-aerobic batch reactor: a performance evaluation study. J Environ Manag 249:109390
Man YB, Chow KL, Cheng Z, Kang Y, Wong MH (2018) Profiles and removal efficiency of organochlorine pesticides with emphasis on DDTs and HCHs by two different sewage treatment works. Environ Technol Innov 9:220–231
Masís-Mora M, Lizano-Fallas V, Tortella G, Beita-Sandí W, Rodríguez-Rodríguez CE (2019) Removal of triazines, triazoles and organophophates in biomixtures and application of a biopurification system for the treatment of laboratory wastewaters. Chemosphere 233:733–743
Mauffret A, Baran N, Joulian C (2017) Effect of pesticides and metabolites on groundwater bacterial community. Sci Total Environ 576:879–887
Meftaul IM, Venkateswarlu K, Dharmarajan R, Annamalai P, Megharaj M (2019) Pesticides in the urban environment: a potential threat that knocks at the door. Sci Total Environ 711:134612
Mijangos L, Ziarrusta H, Ros O, Kortazar L, Fernández LA, Olivares M, Zuloaga O, Prieto A, Etxebarria N (2018) Occurrence of emerging pollutants in estuaries of the Basque Country: analysis of sources and distribution, and assessment of the environmental risk. Water Res 147:152–163
Palma P, Kockschulmeyer M, Alvarenga P, Ledo L, Barbosa IR, De Alda ML, Barcelo D (2014) Risk assessment of pesticides detected in surface water of the Alqueva reservoir (Guadiana basin, southern of Portugal). Sci Total Environ 488–489:208–219.
Papadakis EN, Tsaboula A, Kotopoulou A, Kintzikoglou K, Vryzas Z, Papadopoulou-Mourkidou E (2015) Pesticides in the surface waters of Lake Vistonis Basin, Greece: occurrence and environmental risk assessment. Sci Total Environ 536:793–802
Pressley T (1982) The Determination of organophosphorus pesticides in industrial and municipal wastewater: method 622. USEPA, Washington, DC
Qu CS, Chen W, Bi J, Huang L, Li FY (2011) Ecological risk assessment of pesticide residues in Taihu Lake wetland, China. Ecol Model 222(2):287–292
Qu M, Liu G, Zhao J, Li H, Liu W, Yan Y, Feng X, Zhu D (2020) Fate of atrazine and its relationship with environmental factors in distinctly different lake sediments associated with hydrophytes. Environ Pollut 256:113371
Stone WW, Gilliom RJ, Ryberg KR (2014) Pesticides in US streams and rivers: occurrence and trends during 1992–2011. Environ Sci Technol. https://doi.org/10.1021/es5025367
Tang YF, Wang YX, Cai HS (2004) Use and pollution of organophosphorus pesticides. J Wuhan Inst Chem Technol 26(1):11–14
Triassi M, Nardone A, Giovinetti MC, De Rosa E, Canzanella S, Sarnacchiaro P, Montuori P (2019) Ecological risk and estimates of organophosphate pesticides load into the Central Mediterranean Sea from Volturno River, the river of the “Land of Fires” area, southern Italy. Sci Total Environ 678:741–754
Tsygankov VY (2019) Organochlorine pesticides in marine ecosystems of the Far Eastern Seas of Russia (2000–2017). Water Res 161:43–53
USEPA (2010) USEPA (United States Environmental Protection Agency) ProUCL V. 4.1 Office of Research and Development, Washington, DC
Vryzas Z, Vassiliou G, Alexoudis C, Papadopouloumourkidou E (2009) Spatial and temporal distribution of pesticide residues in surface waters innortheastern Greece. Water Res 43(1):1–10
Wang D, Wang Y, Singh VP, Zhu J, Jiang L, Zeng D, Liu D, Zeng X, Wu J, Wang L, Zeng C (2018) Ecological and health risk assessment of PAHs, OCPs, and PCBs in Taihu Lake basin. Ecol Indic 92:171–180
Water Quality – Determination of organic phosphorous pesticide in water (GB 13192-1991), China. https://www.mee.gov.cn/image20010518/3804.pdf
Wu Y, Zhang S, Ren C, Xie YW, Zhang XW, Sojinu SO, Chen TH, Wang JZ (2015) Residues of organophosphorus insecticides in sediment around a highly eutrophic lake, eastern China. J Soils Sediments 15(2):436–444
Xie H, Wang X, Chen J, Li X, Jia G, Zou Y, Zhang Y, Cui Y (2019) Occurrence, distribution and ecological risks of antibiotics and pesticides in coastal waters around Liaodong Peninsula, China. Sci Total Environ 656:946–951
Xu M, Huang H, Li N, Li F, Wang D, Luo Q (2019) Occurrence and ecological risk of pharmaceuticals and personal care products (PPCPs) and pesticides in typical surface watersheds, China. Ecotoxicol Environ Safe 175:289–298
Yang GL, Wen WANG, Liang SM, Yu YJ, Zhao HY, Qiang WANG, Qian YZ (2017) Pesticide residues in bayberry (Myrica rubra) and probabilistic risk assessment for consumers in Zhejiang, China. J Integr Agric 16(9):2101–2109
Zhang YB, Sun J (1999) Survey of organic phosphorus pesticide both at home and abroad and suggestion of the development of domestic organic phosphorus pesticides. Pesticides 38(7):1–3
Funding
This work was financially supported by the National Natural Science Foundation of China (NSFC) (Grant No. 41877409), the Ministry of Science and Technology of the People’s Republic of China (Grant No. 2015FY110900).
Author information
Authors and Affiliations
Corresponding authors
Additional information
Responsible editor: Ester Heath
Publisher’s note
Springer Nature remains neutral with regard to jurisdictional claims in published maps and institutional affiliations.
Electronic supplementary material
ESM 1
(DOC 61 kb)
Rights and permissions
About this article
Cite this article
Zhang, Y., Qin, P., Lu, S. et al. Occurrence and risk evaluation of organophosphorus pesticides in typical water bodies of Beijing, China. Environ Sci Pollut Res 28, 1454–1463 (2021). https://doi.org/10.1007/s11356-020-10288-z
Received:
Accepted:
Published:
Issue Date:
DOI: https://doi.org/10.1007/s11356-020-10288-z