Abstract
An efficient pretreatment and analytical method was developed to investigate the occurrence and fate of four free estrogens (estrone (E1), 17β-estradiol (17β-E2), estriol (E3), and 17α-ethinylestradiol (EE2)), four conjugated estrogens (estrone-3-sulfate sodium salt (E1-3S), 17β-estradiol-3-sulfate sodium salt (E2-3S), estrone-3-glucuronide sodium salt (E1-3G), and 17β-estradiol-3-glucuronide sodium salt (E2-3G)), and bisphenol A (BPA) in three livestock farms raising beef cattle, cows, sheep, swine, and chickens in Qi County, which is located in North China. The results demonstrated that one cow and one beef cattle excreted 956.25–1,270.41 and 244.38–319.99 μg/day of total (free and conjugated) estrogen, respectively, primarily through feces (greater than 91 %), while swine excreted 260.09–289.99 μg/day of estrogens, primarily through urine (98–99 %). The total estrogen excreted in sheep and broiler chicken feces was calculated to be 21.64–28.67 and 4.62–5.40 μg/day, respectively. It was determined that conjugated estrogens contributed to 21.1–21.9 % of the total estrogen excreted in cow feces and more than 98 % of the total estrogen excreted in swine urine. After composting, the concentration of total estrogen decreased by 18.7–59.6 %; however, increased levels of BPA were measured. In treated compost samples, estrogens were detected at concentrations up to 74.0 ng/g, which indicates a potential risk of estrogens entering the surrounding environment.
Similar content being viewed by others
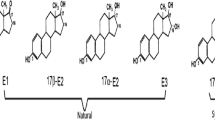
Explore related subjects
Discover the latest articles, news and stories from top researchers in related subjects.Avoid common mistakes on your manuscript.
Introduction
Since the late 1990s, there has been increasing concern of the presence of endocrine-disrupting compounds (EDCs) in the environment, including natural estrogens produced by humans and animals and synthetic chemicals known as xenoestrogens (Belfroid et al. 1999; Panter et al. 2000). Natural estrogens, such as estrone (E1), 17α-estradiol (17α-E2), 17β-estradiol (17β-E2), and estriol (E3) are discharged into the environment from sewage treatment plants (STPs) and livestock manure disposal units (Ternes et al. 1999; Raman et al. 2004; Shi et al. 2004; Combalbert et al. 2011). E1 and 17β-E2 are responsible for the feminization of male fish (Sumpter and Jobling 1995) with their concentrations at the level of nanogram per liter in aquatic environments worldwide including China (Cao et al. 2010; Shi et al. 2013a).
Animals, especially livestock in concentrated animal feeding operation (CAFOs), are an important source of estrogens in the environment. The type and quantity of estrogens excreted depends on the species, sex, age, circadian cycle, and reproductive state of the animal (Hanselman et al. 2003; Combalbert and Hernandez-Raquet 2010). Estrogens are excreted in urine and feces as either biologically active free compounds or inactive compounds conjugated with glucuronide and/or sulfate groups (Panter et al. 1999; Hutchins et al. 2007). In the liver of livestock, estrogens are conjugated to sulfate or glucuronide forms by substitute the hydroxyl groups, which makes free estrogens more soluble and easier to be excreted (Combalbert and Hernandez-Raquet 2010). It was reported that intestinal and fecal microorganisms such as Eschericia coli could hydrolyze estrogen conjugates via glucuronidase and sulfatase enzymes to estrogenically active free forms (Coleman et al. 2004; Ternes et al. 1999). But sulfate conjugates were found in sewage treatment plants as well as in agriculture watershed receiving livestock manure (Dutta et al. 2010; Kumar et al. 2011). Therefore, it is necessary to investigate the occurrence and fate of conjugated estrogens in livestock fresh excreta and manure treatment facilities.
In China, prior to land application, solid manure is composted either in windrows on cement pads or directly on the ground in stacked piles. Estrogens from livestock waste could be discharged into the aquatic environment via surface runoff or land-applied compost. The leaching and migration of estrogens from agricultural fields treated with manure expands the range of contamination, which poses a potential threat to the surrounding groundwater and surface water (Belfroid et al. 1999; Bartelt-Hunt et al. 2011; Dutta et al. 2010). E1 is one of the most frequently detected estrogen in manure, and its sulfate conjugates are also observed in runoff (Dutta et al. 2010). However, the occurrence and fate of conjugated estrogens in compost has not yet been reported. Therefore, it is necessary to investigate the excretion and fate of conjugated estrogens during composting. In addition to natural estrogens, synthetic estrogens, such as 17α-ethinylestradiol (EE2), which are used for human contraception purposes, have been detected in wastewater effluents from CAFO facilities and water in nearby receiving rivers (Chen et al. 2010; Liu et al. 2012) in China despite their prohibition in animal feed additives by the Chinese Ministry of Agriculture (MOA The Ministry of Agriculture of the People’s Republic of China 2013). Other EDCs, such as bisphenol A (BPA), have also been detected in animal manure. BPA may be released from the material that coats the inner surface of food containers or manure storage tanks (Fromme et al. 2002).
To examine the occurrence of EDCs in livestock excreta and compost, an efficient and accurate method to analyze free and conjugated estrogens in high matrix samples must be developed. Our previous study (Chen et al. 2012; Shi et al. 2013b) used accelerated solvent extraction (ASE) to extract four estrogens (E1, 17β-E2, E3, and EE2) and BPA from high matrix samples, such as sewage sludge and sediment. The cleanup procedure should be developed further to extract conjugated estrogens from high matrix samples.
The aims of the present study were the following: (1) to develop a feasible and accurate method to detect E1, 17β-E2, E3, EE2, estrone-3-sulfate sodium salt (E1-3S), 17β-estradiol-3-sulfate sodium salt (E2-3S), estrone-3-glucuronide sodium salt (E1-3G), 17β-estradiol-3-glucuronide sodium salt (E2-3G), and BPA in fresh animal excreta and compost; (2) to investigate the types and concentrations of estrogen compounds contained in the fresh excreta of livestock from the Shanxi Province of China; (3) to calculate the daily estrogen excretion of different animal species; and (4) to assess the removal of the nine EDCs compounds from composted manure.
Materials and methods
Chemicals and materials
E1, 17β-E2, E3, EE2, E1-3S, E2-3S, E1-3G, E2-3G, and BPA were purchased from Sigma-Aldrich, USA and had purities >98 %. Stock solutions of the nine compounds were prepared in high-performance liquid chromatography (HPLC)-grade acetonitrile and stored at −20 °C. Working standards (1–100 ng mL−1) were prepared by serial dilution of the stock solutions with acetonitrile and Milli-Q water (1:1) and stored at 4 °C.
All organic solvents, including acetone, acetonitrile (ACN), methanol (MeOH), n-hexane, dichloromethane (DCM), and ethylacetate (EAC) (HPLC grade), and anhydrous sodium sulfate and sodium hydroxide (analytical reagent grade) were obtained from Mallinckrodt, USA. Sample cleanup and extraction were performed using a Florisil cartridge (500 mg, 6 mL, Waters, Beijing, China), Cleanert NH2 cartridge (500 mg, 6 mL; Waters), Oasis HLB cartridge (200 mg, 6 mL; Waters), and nylon filter (0.22 μm, Sigma-Aldrich, Beijing, China). Wastewater filtration was performed with GF-F glass fiber filters (0.7 μm, Whatman, Shanghai, China). All glassware used in the experiment was soaked in acetone, placed in an ultrasonic bath for 2 h, rinsed with Milli-Q water, and heated to 450 °C for 4 h prior to use. Ultrapure water was provided by a Milli-Q ultrapure water system (Millipore, Beijing).
Sampling sites and sample collection
Samples were collected in May 2012 from three livestock farms in Qi County, which is located in the Taiyuan Basin along the Fen River and is under the administration of Jinzhong City in the southern region of the Shanxi Province in China. Shanxi Province is located in North China, with loess plateau in the north. The topography and weather made the northern and middle part of Shanxi suitable for the breeding of cattle and sheep, where livestock breeding has become one of the pillar industries. In recent years, scattered livestock farms have integrated into CAFOs (in China, it means a farm raising more than 500 of swine, 100 of dairy cattle, 200 of beef cattle, or 20,000 of chicken; MEPC 2001). CAFOs have brought nitrogen and phosphorus pollution load to the already fragile water body in Shanxi, and it cannot be neglected that they might brought potential estrogenic risks as well. By the end of 2010, there were (in thousands) 119.5 beef cattle, 16.7 dairy cattle, 114 sheep, 266 swine, and 2,747 chickens in Qi County (Animal Husbandry Bureau of Qi County, 2011), generating a large amount of waste, which was not being effectively managed. The fattening of beef cattle has become a pillar industry in Jinzhong City, and Qi County is now the demonstration plots for the cultivation and fattening of beef cattle with straw.
Fresh excreta and compost samples were collected from the following three livestock farms: Xinben Livestock Farm Cooperation, Dayiyuan Swine Farm, and Xinsheng Sheep Farm. According to the site investigation, Xinben Livestock Farm Cooperation is the largest beef cattle farm in Shanxi Province with 7,800 beef cattle. The Xinben Livestock Farm Cooperation also raises 100 cows, 3,000 sheep, and 30,000 chickens, as well. The Dayiyuan Swine Farm raises 2,000 swine, and the Xinsheng Farm raises 1,500 sheep. Livestock feces from the three farms were composted in several individual heaps classified by animal species. Composting was conducted naturally on the ground without intentionally adding other material like straw. According to the description of the farm owner and field investigation, more than 98 % of the composting material was animal manure, and the less than 2 % was straw or some other material which was unintentionally added. No precautionary measures were performed to rain-proof the compost or prevent seepage. Pictures of the composting sites are provided in the Supplementary Material.
For each livestock species, excreta of six individual animals of the same species, sex, age and reproductive stage were mixed and then split into three parallel samples. Similarly, compost at six points of the same pile were mixed and then split into three parallel samples for each species. Fecal and urine samples were collected immediately after excretion and stored in precleaned, 500-mL glass bottles. Compost samples were also collected in precleaned 500-mL glass bottles. The bottles contained 10 mL MeOH and 50 mL sodium azide (0.5 mg L−1), which effectively prevented the target compounds from microbiological degradation. The collected samples were packed in cool boxes with ice (<4 °C) and transported to the laboratory. Urine and wastewater samples were analyzed upon arrival at the laboratory, and the fecal and compost samples were immediately frozen at −20 °C for further pretreatment and analysis.
Pretreatment of urine samples before estrogen analysis
Milli-Q water was used to dilute 10 mL of each urine sample to 100 mL in a glass bottle. Prior to extraction, pH value of each diluted urine sample was adjusted to 3 using 4 mol L−1 HCl. First, the sample was loaded onto an Oasis HLB SPE cartridge which was conditioned with 5 mL EAC, 5 mL MeOH, and 10 mL Milli-Q water at a flow rate of approximately 10 mL min−1. Second, to obtain both free and conjugated estrogens, a NH2 cartridge was connected below the HLB cartridge containing the sample extract. Thus, the eluted solution from HLB cartridge had to filter through the NH2 cartridge before being collected in a centrifuge tube. As conjugated estrogens are more polar than free ones, they can be retained in NH2 cartridge, while free estrogens and BPA can go through NH2 cartridge with the elution. The elution (part A) was collected using 10 mL MeOH, and the conjugated estrogens remained on the NH2 cartridge. Then, 10 mL of 2 % NH4OH methanol was loaded onto the NH2 cartridge to elute the conjugated estrogens (part B). The part A elution was air dried under a gentle nitrogen flow and reconstituted with 5 mL n-hexane/DCM (3:1, v/v). Third, the reconstituted sample was filtered through a Florisil cartridge (preconditioned successively with 5 mL n-hexane and 5 mL n-hexane/DCM) at a flow rate of approximately 1 mL min−1, and the Florisil cartridge was eluted with 6 mL acetone/n-hexane (1:4, v/v) (part C). The mixture of elutions of parts B and C was air dried under a gentle stream of nitrogen and reconstituted in 1 mL MeOH prior to LC–MS/MS analysis.
Pretreatment of fecal and compost samples before LC-MS analysis
Each frozen fecal or compost sample was freeze-dried in a lyophilizer, and the dried sample was uniformly mixed with a pestle. A total of 1 g of the fecal or compost sample was precisely weighed, mixed with 5 g Florisil, and placed at the bottom of a precleaned extraction cell (66 mL). The preparation of the extraction solvent, ASE (ASE 300, Dionex, USA) operation, and evaporation were performed according to the protocol developed by Chen et al. (2012).
Cleanup using a NH2 cartridge was added to the four-step cleanup procedure reported by Chen et al. (2012): liquid–liquid extraction (LLE), Florisil cleanup, aqueous alkali extraction (AAE), and hydrophilic–lipophilic balance (HLB) enrichment. A five-step cleanup procedure was developed to extract conjugated estrogens from fecal or compost samples. First, the evaporated sample was reconstituted in 5 mL ACN. Then, 10 mL n-hexane was added, and the mixture was subjected to liquid–liquid extraction. The solution was mixed using a vortex mixer for 10 min and centrifuged at 2,500 r min−1 for 10 min before the upper n-hexane layer was removed. This process was repeated two more times. Second, an aqueous alkali extraction was performed. The ACN layer acquired from the first step was air dried under a gentle stream of nitrogen, reconstituted in 5 mL of 0.1 mol/L NaOH in an ultrasonic bath for 10 min, and mixed using a vortex mixer for 2 min. The solution was filtered through a 0.22-μm nylon filter and transferred into a 500-mL precleaned sample bottle. A total of 100 mL Milli-Q water and 6 mL methanol was added to each sample bottle, and the pH was adjusted to 3 using 4 mol/L HCl. The last three steps, including HLB extraction, NH2 cartridge cleanup, and Florisil cartridge cleanup, were performed in a similar manner to the preparation of the urine samples.
LC-MS/MS analysis
The target compounds were analyzed by LC-MS/MS using an Agilent 1100 HPLC coupled to a 3200 QTRAP mass spectrometer (Applied Biosystems, USA), which was equipped with Analyst 1.4.1 data processing software. The conditions of the HPLC mobile phase gradient are listed in Table 1. A Nova-Pak C18 HPLC column (150 mm × 3.9 mm, 4 μm, Waters) was used. Electrospray ionization was used in the negative ion mode, and the multiple-reaction monitoring (MRM) mode was used to analyze the estrogens. The LC-MS/MS parameters for E1, 17β-E2, E3, EE2, and BPA were referenced from the protocol developed by Chen et al. (2012). Analysis of E1-3S and 17β-E2-3S was performed under the same conditions that were used to analyze the free-form estrogens. The mobile phase solvents and LC-MS/MS parameters of E1-3G and 17β-E2-3G are shown in Table 2.
Quality assurance and quality control
Before analyzing the samples, a standard solution containing the nine target compounds was prepared and used to identify the analytical conditions of LC-MS/MS. A good linear relationship between concentration and signal was observed within the range of estrogen concentrations detected in the samples. The concentrations of the standard curve were 1, 2, 5, 10, 20, 50, and 100 ng/mL. All linear correlation coefficients between the concentrations and peaks of the nine target compounds were greater than 0.995. The limit of detection (LOD) of the instrument (Table 2) was determined using the signal-to-noise ratio. The method detection limits are summarized in Table 2s, Supplementary Material. The repeatability was checked by analyzing duplicate samples. The relative standard deviation (RSD) ranged from 2 to 20 %. Recoveries were calculated based on the triplicate analyses of urine, feces, and compost samples supplemented with standard solutions containing the target compounds at concentrations of 10 μg/L, 10 μg/L, 10 ng/g, and 10 ng/g, respectively. The recovery of each sample is shown in Table 2.
Results and discussion
Occurrence of free estrogens, conjugated estrogens, and BPA in fresh excreta
To investigate the concentration of free estrogens, conjugated estrogens, and BPA in the excreta of different animal mature, fresh fecal and urine samples were collected from three farms, as shown in Table 3. Of the free estrogens, E1 and 17β-E2 were more frequently detected in fresh feces than E3. The most frequently detected estrogen in fresh urine was E1, followed by 17β-E2 and E3, which confirms that E1 and 17β-E2 are the predominant estrogens excreted by cattle (Combalbert et al. 2010; Hanselman et al. 2003; Shore and Shemesh 2003). Of the conjugated estrogens, E1-3S and 17β-E2-3S were detected in all samples, whereas E1-3G and 17β-E2-3G were only detected in urine. This is because glucuronide conjugates are predominantly excreted through animal urine (Hanselman et al. 2003), and sulfate conjugates are more recalcitrant and difficult to deconjugate than glucuronide estrogens (D’Ascenzo et al. 2003; Palme et al. 1996).
In addition, the amounts of estrogenic compounds varied between different animal species, as shown in Table 3. For example, E3 was neither detected in feces nor in urine of cattle, and E1 was not detected in feces of beef cattle. The total concentrations of free estrogens (E1, 17β-E2, and E3) were higher in the feces of cows (102.0–136.4 ng/g) than beef cattle (17.3–25.9 ng/g). Lange et al. (2002) reported a slightly lower concentration of free estrogens in cow feces (5 ng/g) compared to beef cattle feces (9 ng/g). These concentrations are lower than the concentrations reported in the present study. Shore and Shemesh (2003) measured estrogen (E1 and 17β-E2) concentrations ranging from 9.9 to 71.4 ng/g in cow feces, which is on the same order of magnitude but slightly lower than the concentrations measured in this study. A higher concentration of total free estrogens was measured in urine samples from beef cattle (0.646–0.814 μg/L) compared to urine samples from cows (0.067–0.069 μg/L). Additionally, 17β-E2 was not detected in cow urine.
Previous studies mainly focused on the concentration of free estrogens in cattle excreta and seldom reported on the concentration of conjugated estrogens. The total concentration of estrogens (free and conjugated) measured in cow feces (130.6–172.8 ng/g) was also higher than the total concentration of estrogens measured in beef cattle feces (55.2–73.2 ng/g). However, a lower percentage of conjugated estrogens was measured in cow feces (21.1–21.9 %) compared to beef cattle feces (64.6–68.7 %). Unlike feces, the total concentration of estrogens (free and conjugated) was lower in cow urine (1.865–2.003 ng/g) than in beef cattle urine (2.915–3.290 ng/g); however, a higher percentage of conjugated estrogens was measured in cow urine (96.4–96.5 %) than beef cattle urine (75.3–77.8 %).
E1 (5.6–6.2 ng/g) was the only free estrogen detected in sow feces, and both E1 and E3 were detected in sow urine. The total (free and conjugated) estrogen concentration was lower in sow feces (23.1–25.7 ng/g) than cattle feces, but a higher total estrogen concentration was measured in sow urine (71.272–79.474 μg/L) compared to cattle urine. Previous studies have also reported that E1 is the predominant free estrogen in swine excreta (Johnson et al. 2006). Shore and Shemesh (2003) used differential enzyme hydrolysis and radio immunoassay to report the detection of E1 in sow feces at concentrations ranging from 15 to 28 ng/g.
Three free estrogens (E1, 17β-E2, and E3) were detected in chicken feces, and the concentration of free estrogens ranged from 45.3 to 52.3 ng/g. The concentration of total estrogens (free and conjugated) measured in chicken feces was 72.4–84.6 ng/g, with conjugated estrogens contributing 37.4–38.2 %. The main estrogens excreted by chickens were reported to be E1 and E3 (Shore and Shemesh 2003). Chickens were also found to excrete 17β-E2 (Hanselman et al. 2003). Johnson et al. (2006) estimated the average concentration of estrogens in broiler chicken feces to be 39 ng/g, with average concentrations of E1 and 17β-E2 being 29 and 12 ng/g, respectively. The concentrations of E1 and 17β-E2 that were estimated by Johnson et al. are similar to the concentrations measured in the present study. However, the total concentration of estrogens estimated by Johnson et al. is lower than the concentration we measured. This is because E3 was excluded from their analysis.
In sheep feces, E3 was not detected, and the concentration of total free estrogens (E1 and 17β-E2) ranged from 10.6 to 12.8 ng/g. The concentrations of individual estrogen compounds in sheep feces have rarely been reported. The concentration of total (free and conjugated) estrogens was 33.3 to 44.1 ng/g, with conjugated estrogens contributing to 68.2 to 71.0 %. Because the sheep were easily startled during sampling, sheep urine samples were not able to be collected; therefore, no data on sheep urine were available in this study.
The synthetic estrogen EE2 was not detected in any of the fecal or urine samples. It has been reported that EE2 is used by farmers to improve protein deposition and increase body weight. However, its use is forbidden in China and the European Union because of the carcinogenic potential of its residues (MOA 2013; Durant et al. 2002). Similar concentrations of BPA were measured in fecal (nd, 4.1 ng/g) and urine samples (346–2,120 ng/L).
Daily excretions of free estrogens, conjugated estrogens, and BPA by livestock
Considering fresh fecal and urine production, water content, and estrogen concentrations, the daily estrogen excretion of four free and four conjugated estrogens was calculated using the formula and parameters in the Supplementary Material. The results are listed in Table 4. The calculated daily excretion of total (free and conjugated) estrogens in the feces of one cow was 1,159.86–1,533.12 μg/day, which was higher than the daily estrogen that was excreted from one beef cattle (223.71–296.65 μg/day) and other investigated animals. The total estrogens (free and conjugated) excreted in the urine of one cow and one beef cattle were 23.59–26.42 and 20.67–23.34 μg/day, respectively. This indicates that free and conjugated estrogens are mostly excreted in cattle feces (greater than 91 %). Shore and Shemesh (2003) reported that cattle mostly excreted free estrogens (not conjugated estrogens) in their feces (greater than 90 %). Sheep excreted less total (free and conjugated) estrogens than cattle, ranging 21.64–28.67 μg/day, which is similar to the concentration estimated by Johnson et al. (2006) (20–22 μg/day) even though conjugated estrogens were excluded from their study.
As calculated in this study, a sow excreted 14.98–16.67 and 245.11–273.32 μg/day of total (free and conjugated) estrogens in their feces and urine, respectively, indicating that approximately 94 % of the estrogens were excreted in the urine. Other results have shown that swine excrete mostly free estrogens in their urine (70–99 % by Johnson et al. (2006) and 90 % by Combalbert and Hernandez-Raquet (2010)). One broiler chicken excreted 4.62–5.40 μg/day of total estrogens. Shore and Shemesh (2003) reported that a nonlaying hen excreted 2.5 μg/day of estrogens (only E1 and 17β-E2).
It has been reported that most estrogens excreted in urine are conjugated compounds, whereas most estrogens excreted in the feces of livestock animals are free estrogen compounds (Hanselman et al. 2003). In this study, conjugated estrogens contributed to 96.4–96.5, 75.3–77.8, and more than 98 % of the total estrogens detected in the urine of cows, beef cattle, and swine, respectively. However, conjugated estrogens contributed to 21.1–21.9, 64.6–68.6, 48.3–68.1, 75.7–75.9, and 37.4–38.2 % of the total estrogens in the fecal excretions from cows, beef cattle, sheep, swine, and chickens, respectively. These results were obtained because of the effective pretreatment and precise LC-MS/MS analytical detection of free and conjugated estrogens.
Assuming that BPA was continually excreted in feces and urine, the daily excretion of BPA from cows and beef cattle was determined to be 23.95 and 15.03 μg/day, respectively, as shown in Table 4. Differences in BPA excretion were attributed to variations in feeding methods and feces/urine production.
Removal of free estrogens, conjugated estrogens, and BPA from compost
E1 (nd–36.0 ng/g) and 17β-E2 (nd–32.9 ng/g) were the most frequently detected estrogens in compost samples, whereas E3 and glucuronide conjugates were not detected at all, as shown in Fig. 1. EE2 (3.3–4.5 ng/g) was only detected in cow compost samples collected from Xinben Livestock Farm Cooperation. Sulfate conjugates were identified in the compost samples; a higher concentration of 17β-E2-3S (13.0–30.0 ng/g) than E1-3S (nd–12.5 ng/g, p < 0.005) was detected. Glucuronide conjugates were not detected in the compost samples. All compost samples were collected from compost products that were ready to be used on farmland, and composting time varied from 3 to 6 weeks according to situation of livestock excreta and composting conditions. However, estrogen compounds were still detected in compost products with total (free and conjugated) estrogen concentrations ranging from 13.0 to 74.0 ng/g. Estrogens in land-applied compost could leach and migrate by runoff into the aquatic environment and pose a potential threat to the surrounding groundwater and surface water (Belfroid et al. 1999; Bartelt-Hunt et al. 2011; Dutta et al. 2012). In the area studied, feces and urine were generally composted directly on the ground. No precautionary measures were performed to rain-proof the compost or prevent seepage, which could increase the possibility of estrogens entering the aquatic environment.
According to the farmers at Xinben Livestock Farm Cooperation, the compost samples were approximately 1 month old. Variations in the concentration of free estrogens, conjugated estrogens, and BPA between fecal samples and compost products are shown in Fig. 2. After composting, the concentration of free estrogens in chicken, beef cattle, cow, and sheep feces decreased by 6.5, 67.6, 55.8, and 100 %, respectively. On the contrary, a higher concentration of free estrogens was measured in swine compost samples compared to swine fecal samples. This difference is most likely observed because swine predominantly excrete estrogen compounds in their urine, which is mixed with fecal waste during composting. Thus, the data are insufficient to determine whether estrogens were removed from swine compost samples. Conjugated estrogens can be deconjugated into free forms, which increased the concentration of free estrogens. Because of deconjugation, the concentrations of conjugated estrogens were generally lower in compost samples than fecal samples, as shown in Fig. 2. After composting, the total concentration of conjugated estrogens in chicken, swine, beef cattle, cow, and sheep feces decreased by 40.5, 2.6, 7.7, 36.5, and 41.9 %, respectively. The removal efficiency of conjugated estrogens from compost has seldom been reported.
After composting, the total (free and conjugated) estrogen removal efficiencies were 18.7, 34.1, 50.9, and 59.6 % for chicken, beef cattle, cow, and sheep excreta, respectively. A lower concentration of total estrogens was measured in swine fecal samples than in swine compost samples because swine predominantly excrete estrogens in their urine, which is also included in the composting material. The concentration of BPA increased after composting (3.2–28.4 ng/g). It is more likely that conjugated BPA was deconjugated during the composting. However, based solely on the concentration of estrogens and BPA in fresh and composted excreta, it was difficult to determine the total amount that was removed during composting. Since there is no precautionary measures performed to rain-proof the compost or prevent seepage, the removal efficiencies of the chemicals may be overestimated. Therefore, more accurate removal efficiencies could be attained by performing a controlled composting experiment in the laboratory.
Although removal efficiencies of up to 59.6 % of total estrogen were attained in the present study, some improvements should be considered to enhance composting at Xinben Livestock Farm Cooperation. According to the field investigation performed during sample collection, manure was distributed over the ground to increase air contact and improve aeration. However, under this naturally dry condition the moisture content and microorganism activity of manure is reduced (El Kader et al. 2007), which may affect estrogen removal efficiency. Additionally, a lower moisture content might also decrease the quantity of ammoniacal nitrogen and reduce the value of the organic fertilizer (Le et al. 2012). Parkinson et al. (2004) have suggested that seasonal weather patterns strongly affect nutrient loss and that effective composting management should be implemented, such as using proper facilities and turning and watering the compost pile. Thus, to minimize estrogenic risks, reduce nutrient loss, and maximize financial benefits, some necessary improvements to compost management should be considered for the livestock farms of Qi County, such as building fences, adding rain- and seepage-proof facilities, and regulating the moisture content and temperature of the compost.
Conclusions
Due to the reliable pretreatment processes and analytical methods, the estrogen excretions of livestock animals were better characterized and quantified. Cows and beef cattle excreted 956.25–1,270.41 and 244.38–319.99 μg/day of total estrogens, respectively, primarily through feces (greater than 91 %), whereas swine excreted 260.09–289.99 μg/day of estrogens, primarily through urine (98–99 %).
Conjugated estrogens contributed 21.1–21.9, 64.6–68.6, 48.3–68.1, 75.7–75.9, and 37.4–38.2 % to the total estrogen excretion in the feces of cows, beef cattle, sheep, swine, and chickens, respectively. And they also contributed 96.4–96.5, 75.3–77.8, and more than 98 % to the total estrogen excreted in the urine of cows, beef cattle, and swine, respectively.
Composting treatment used in Qi County removed 18.7, 34.1, 50.9, and 59.6 % of estrogens (free and conjugated) from chicken, beef cattle, cow, and sheep feces, respectively. The concentration of estrogens in compost samples ranged from 13.0 to 74.0 ng/g, which is a potential estrogenic risk to the surrounding environment. Moreover, to increase estrogen removal efficiency and reduce nutrient loss, improved composting management should be considered for the livestock farms of Qi County.
References
Bartelt-Hunt S, Snow DD, Damon-Powell T, Miesbach D (2011) Occurrence of steroid hormones and antibiotics in shallow groundwater impacted by livestock waste control facilities. J Contam Hydrol 123:94–103
Belfroid AC, Van der Horst A, Vethaak AD, Sch A, Fer AJ, Rijs G, Wegener J, Cofino WP (1999) Analysis and occurrence of estrogenic hormones and their glucuronides in surface water and waste water in The Netherlands. Sci Total Environ 225:101–108
Cao J, Shi J, Han R, Li Y, Yang Z (2010) Seasonal variations in the occurrence and distribution of estrogens and pharmaceuticals in the Zhangweinanyun river system. Chin Sci Bull 55:3138–3144
Chen Q, Shi J, Wu W, Liu X, Zhang H (2012) A new pretreatment and improved method for determination of selected estrogens in high matrix solid sewage samples by liquid chromatography mass spectrometry. Microchem J 104:49–55
Chen T, Chen T, Yeh KJC, Chao H, Liaw E, Hsieh CY, Chen K, Hsieh LT, Yeh YL (2010) High estrogen concentrations in receiving river discharge from a concentrated livestock feedlot. Sci Total Environ 408:3223–3230
Coleman HM, Routledge EJ, Sumpter JP, Eggins BR, Byrne JA (2004) Rapid loss of estrogenicity of steroid estrogens by UVA photolysis and photocatalysis over an immobilised titanium dioxide catalyst. Water Res 38:3233–3240
Combalbert S, Hernandez-Raquet G (2010) Occurrence, fate, and biodegradation of estrogens in sewage and manure. Appl Microbiol Biot 86:1671–1692
Combalbert S, Pype M, Bernet N, Hernandez-Raquet G (2010) Enhanced methods for conditioning, storage, and extraction of liquid and solid samples of manure for determination of steroid hormones by solid-phase extraction and gas chromatography-mass spectrometry. Anal Bioanal Chem 398:973–984
Combalbert S, Bellet V, Dabert P, Bernet N, Balaguer P, Hernandez-Raquet G (2011) Fate of steroid hormones and endocrine activities in swine manure disposal and treatment facilities. Water Res 46:895–906
D'Ascenzo G, Di Corcia A, Gentili A, Mancini R, Mastropasqua R, Nazzari M, Samperi R (2003) Fate of natural estrogen conjugates in municipal sewage transport and treatment facilities. Sci Total Environ 302:199–209
Durant AA, Fente CA, Franco CM, Vázquez BI, Cepeda A (2002) Gas chromatography-tandem mass spectrometry determination of 17α-ethinylestradiol residue in the hair of cattle. Application to treated animals. J Agr Food Chem 50:436–440
Dutta S, Inamdar S, Tso J, Aga DS, Sims JT (2010) Free and conjugated estrogen exports in surface-runoff from poultry litter-amended soil. J Environ Qual 39:1688–1698
Dutta S, Inamdar S, Tso J, Aga DS (2012) Concentrations of free and conjugated estrogens at different landscape positions in an agricultural watershed receiving poultry litter. Water Air Soil Poll 225:2821–2836
El Kader NA, Robin P, Paillat JM, Leterme P (2007) Turning, compacting and the addition of water as factors affecting gaseous emissions in farm manure composting. Bioresour Technol 98:2619–2628
Fromme H, Küchler T, Otto T, Pilz K, Müller J, Wenzel A (2002) Occurrence of phthalates and bisphenol A and F in the environment. Water Resour 36:1429–1438
Hanselman TA, Graetz DA, Wilkie AC (2003) Manure-borne estrogens as potential environmental contaminants: a review. Environ Sci Technol 37:5471–5478
Hutchins SR, White MV, Hudson FM, Fine DD (2007) Analysis of lagoon samples from different concentrated animal feeding operations for estrogens and estrogen conjugates. Environ Sci Technol 41:738–744
Johnson AC, Williams RJ, Matthiessen P (2006) The potential steroid hormone contribution of farm animals to freshwaters, the United Kingdom as a case study. Sci Total Environ 362:166–178
Kumar V, Nakada N, Yasojima M, Yamashita N, Johnson AC, Tanaka H (2011) The arrival and discharge of conjugated estrogens from a range of different sewage treatment plants in the UK. Chemosphere 82:1124–1128
Lange IG, Daxenberger A, Schiffer B, Witters H, Ibarreta D, Meyer HHD (2002) Sex hormones originating from different livestock production systems: fate and potential disrupting activity in the environment. Analytica Chimica Acta 473:27–37
Le TAH, Clemens J, Nguyen TH (2012) Performance of different composting techniques in reducing oestrogens content in manure from livestock in a Vietnamese setting. Environ Monit Assess 185:415–423
Liu S, Ying G, Zhang R, Zhou L, Lai H, Chen Z (2012) Fate and occurrence of steroids in swine and dairy cattle farms with different farming scales and wastes disposal systems. Environ Pollut 170:190–201
MEPC (Ministry of Environmental Protection of the People’s Republic of China) (2001) Discharge standard of pollutants for livestock and poultry breeding, Beijing (in Chinese)
MOA (The Ministry of Agriculture of the People’s Republic of China) (2013) Approved feed additives (2013). Ministry of Agriculture the People’s Republic of China, Beijing (in Chinese)
Palme R, Fischer P, Schildorfer H, Ismail MN (1996) Excretion of 14C-steroid hormones via feces and urine in domestic livestock. Anim Reprod Sci 43:43–63
Panter GH, Thompson RS, Beresford N, Sumpter JP (1999) Transformation of a non-oestrogenic steroid metabolite to an oestrogenically active substance by minimal bacterial activity. Chemosphere 38:3579–3596
Panter GH, Thompson RS, Sumpter JP (2000) Intermittent exposure of fish to estradiol. Environ Sci Technol 34:2756–2760
Parkinson R, Gibbs P, Burchett S, Misselbrook T (2004) Effect of turning regime and seasonal weather conditions on nitrogen and phosphorus losses during aerobic composting of cattle manure. Bioresour Technol 91:171–178
QAHB (Qi Animal Husbandry Bureau) (2011) Development planning of livestock breeding in Qi County. Available from: http://www.3948.com/web/ty/xxgknr.asp?id=398&dtid=1505
Raman DR, Williams EL, Layton AC, Burns RT, James P, Daugherty AS, Mullen MD, Sayler GS (2004) Estrogen content of dairy and swine wastes. Environ Sci Technol 38:3567–3573
Shi J, Fujisawa S, Nakai S, Hosomi M (2004) Biodegradation of natural and synthetic estrogens by nitrifying activated sludge and ammonia-oxidizing bacterium Nitrosomonas europaea. Water Resour 38:2323–2330
Shi J, Liu X, Cao J, Li Y, Yang Z (2013a) Occurrence and risk assessment of estrogens and anti-inflammatories in Baiyangdian Lake, North China. Environ Eng Manag J12:1437–1445
Shi J, Chen Q, Liu X, Zhan X, Li J, Li Z (2013b) Sludge/water partition and biochemical transformation of estrone and 17β-estradiol in a pilot-scale step-feed anoxic/oxic wastewater treatment system. Biochem Eng J 74:107–114
Shore LS, Shemesh M (2003) Topic 2.2: Naturally produced steroid hormones and their release into the environment. Pure Appl Chem 75:1859–1872
Sumpter JP, Jobling S (1995) Vitellogenesis as a biomarker for estrogenic contamination of the aquatic environment. Environ Health Perspect 103:173–178
Ternes TA, Stumpf M, Mueller J (1999) Behaviour and occurrence of estrogens in municipal sewage treatment plants—II. Aerobic batch experiments with activated sludge. Sci Total Environ 225:91–99
Acknowledgments
This study was supported by the National Basic Research Program of China (973 Program, 2010CB429003), Special Fund of State Key Joint Laboratory of Environment Simulation and Pollution Control (No. 13L01ESPC), and Youth Science and Technology Fund for Shanxi Basic Research Project (2013011040-7). The manuscript has been edited by Elsevier Webshop Support for the English language.
Author information
Authors and Affiliations
Corresponding author
Additional information
Responsible editor: Ester Heath
Electronic supplementary material
Below is the link to the electronic supplementary material.
ESM 1
(DOC 214 kb)
Rights and permissions
About this article
Cite this article
Zhang, H., Shi, J., Liu, X. et al. Occurrence of free estrogens, conjugated estrogens, and bisphenol A in fresh livestock excreta and their removal by composting in North China. Environ Sci Pollut Res 21, 9939–9947 (2014). https://doi.org/10.1007/s11356-014-3002-9
Received:
Accepted:
Published:
Issue Date:
DOI: https://doi.org/10.1007/s11356-014-3002-9