Abstract
Cultivation of specialty mushrooms on lignocellulosic wastes represents one of the most economical organic recycling processes. Compared with other cultivated mushrooms, very little is known about the nature of the lignocellulolytic enzymes produced by the edible and medicinal fungus Grifola frondosa, the parameters affecting their production, and enzyme activity profiles during different stages of the developmental cycle. In this work we investigated the enzymes that enable G. frondosa, to colonize and deconstruct two formulations based on industrial lignocellulosic by-products. G. frondosa degraded both substrates (oak-sawdust plus corn bran, and oak/corn bran supplemented with coffee spent-ground) decreasing 67 and 50% of their lignin content, along with 44 and 37% of the polysaccharides (hemicellulose and cellulose) respectively. 35.3% biological efficiency was obtained when using oak sawdust plus corn bran as substrate. Coffee spent-ground addition inhibited mushroom production, decreased growth, xylanase and cellulase activities. However, taking into account that G. frondosa successfully colonized this residue; this substrate formula might be considered for its growth and medicinal polysaccharide production. Although G. frondosa tested positive for Azure B plate degradation, a qualitative assay for lignin-peroxidase, attempts to detect this activity during solid state fermentation were unsuccessful. Enzyme activities peaked during colonization but declined drastically during fruiting body formation. Highest activities achieved were: endoglucanase 12.3, exoglucanase 16.2, β-glucosidase 2.3, endoxylanase 20.3, amylase 0.26, laccase 14.8 and Mn-peroxidase 7.4 U/g dry substrate.
Similar content being viewed by others
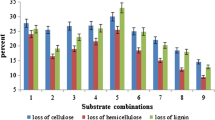
Explore related subjects
Discover the latest articles, news and stories from top researchers in related subjects.Avoid common mistakes on your manuscript.
Introduction
Grifola frondosa (Dicks.:Fr.) S.F. Gray, commonly known as maitake, a wood decay basidiomycete, is one of the most popular edible mushrooms. It is a nutritious food source and is reported to contain bioactive metabolites which exhibit many medicinal effects including antitumour, hypocholesterolemic, antioxidant and antidiabetic activities (Illana-Esteban 2008).
White-rot fungi, like G. frondosa, are known to produce a wide range of hydrolytic and oxidative enzymes that enable them to successfully colonize, degrade and bioconvert many lignocellulosic substrates. Such degradation results from the concerted and synergistic action of many enzymes. Briefly, cellulose is a linear polymer of glucose units, which can be hydrolyzed by the action of endoglucanases, exoglucanases and β-glucosidases. Hemicellulose is a heterogeneous, branched polymer. The backbone of the polymer is built up by sugar monomers like xylose, in this case the enzymes involved in its degradation are named xylanases. Similar to cellulases, the xylanases can act synergistically to achieve hydrolysis; predominant enzymes within this system are endoxylanases which attack the polysaccharide backbone, and β-xylosidases, which hydrolyze short xylooligosaccharides to xylose. Lignin is a polymer of phenylpropane units connected by diverse C–C and C–O–C linkages. This molecule is degraded by (at least) three enzymes: lignin-peroxidase (LiP), Mn-peroxidase (MnP), and laccase (Eriksson et al. 1990). The production of these enzymes is important in substrate colonization, as well as decisive in fruit body production (Ohga and Royse 2001).
Thus far, little effort has been directed to improve culture conditions for G. frondosa commercial development, due to its short history of cultivation (Shen and Royse 2001). In spite of the commercial significance of this mushroom, our current knowledge on its physiology is limited, and therefore further research is needed to realize its potential. In order to obtain the nutrients required for growth and fruiting, G. frondosa is assumed to secrete the hydrolytic/oxidative enzymes that catalyze the degradation of the major macromolecular components (cellulose, hemicellulose and lignin) of its growth substrate. However, compared with other cultivated mushrooms, very little is known about the nature of the lignocellulolytic enzymes produced by G. frondosa, the parameters affecting their production, and enzyme activity profiles during different stages of the developmental cycle (Orth et al. 1993; Svagelj et al. 2007; Xing et al. 2006; Yoshida et al. 2002).
The suitability of a mushroom-culturing substrate depends, in great part, on its composition, availability, and cost. Diverse technologies have been proposed for utilizing the byproducts generated by the coffee industry (Pandey et al. 2000a). Culturing edible mushrooms on coffee-spent ground seems especially attractive, since it represents a direct conversion of an agricultural waste to human food. Finally, coffee byproducts degraded by mushroom cultures might have further post-production value in the elaboration of forages, vermicomposts, and organic fertilizers (Salmones et al. 2005).
The objectives of the present work were the characterization of G. frondosa growth, fruiting and lignocellulolytic enzyme production during solid state fermentation (SSF) on two formulations based on industrial Colombian by-products: coffee spent-ground (obtained during the processing of raw coffee powder to prepare “instant coffee”) and oak-sawdust (a residue from the industry devoted to the production of oak barrels for the liquor manufacturing).
Materials and methods
Organism and culture conditions
Grifola frondosa (PSUMCC 922) was obtained from the Pennsylvania State University Mushroom Culture Collection, USA, and maintained on potato dextrose agar (PDA) at 4°C with periodic transfer. The mycelium was transferred from the stock culture to the center of a Petri dish containing PDA and incubated at 25°C for 21 days. The experiment was conducted under greenhouse conditions, in Manizales Colombia, located at 2,250 m above the sea level, where the average annual temperature and the relative humidity (RH) are 17°C and 70% respectively.
Spawn preparation
Five pieces of 1.0 cm2 of PDA medium colonized by the mycelium were inoculated in a 500 ml glass jar, in which half of the volume contained corn grains. To adjust the moisture content of the grains for spawn preparation, they were soaked in hot water (0.5 kg/l), drained, cooled off and dried up to a moisture content of 33–40% of fresh weight. The jars were incubated at 25°C for 25 days, until total substrate colonization.
Substrates and fruiting conditions
Substrates basic raw materials were oak sawdust (Quercus sp.), by-product of the company Cubas y Barriles de Colombia S.A. (Cubacol S.A.) and coffee spent-ground, by-product of the company FOODEX S.A. Both companies are located in Manizales, Colombia. Two different formulations were assayed. The first one consisted of (dry weight basis) 75% oak sawdust (25% moisture content), 23% corn bran (15% moisture content), 1% sucrose (2% moisture content) and 1% calcium carbonate. In the second formulation, the oak sawdust was replaced by 50% oak sawdust and 25% coffee spent-ground (70% moisture content). The substrates were packed in polypropylene bags and autoclaved at 121°C for 1 h. The moisture content was calculated in relation to the dry weight of the components. It was measured after drying the samples in an electric forced convection oven (Binder®, max. 300°C). Each bag of 32 cm height and 12 cm diameter contained 1 kg of substrate (58% moisture content). One square hole of 2.54 cm2 was made at the top of each bag and covered with a microporous breather strip to allow for gas exchange. The bags containing the composts were aseptically inoculated with 3% (wet basis) of spawn. During the first week (adaptation phase to the new substrate), the cultures were maintained at 25°C and 60% RH, to facilitate a faster growth. Then, they were incubated at 20 ± 1°C for approx. 23 days more, until the complete colonization of the substrate. The third step before primordial formation, which included the development of a dense white mycelial mat (mycelial coat), that progressively acquired an orange brown color, and ended with the production of an orange exudates, required 40–45 days at 18–20°C and 60–65% RH. A cold shock to 10°C for 24 h (induction) was essential to facilitate primordial initiation. While the mycelium could grow in the dark, a low level of light (50–100 luxes) was necessary afterwards for basidiome development. The period of basidiome development, which was initiated when the primordia began to grow and differentiate to form small pilei and stipes (7–10 days) required one air exchange (16–18°C and 70–80% RH, around 10 min) every 12 h. At this stage, holes were cut in the bags exposing the developing primordia. The next phase, until harvesting, lasted 3–5 days and required an air-renewal of 10 min every 2 h [in order to keep CO2 concentrations below 700 ppm, measured with a portable gas analyzer (CheckPoint O2/CO2 PBI-Dansensor® equipped with a non-dispersive infrared (NDIR) sensor, accuracy 2%)] and 70–80% RH. The total time required for spawn run, primordial formation and mature basidiome harvest was in the range of 81–96 days (first crop cycle). About 15–20 additional days were required for a second crop (Montoya-Barreto et al. 2008). Biological efficiency (BE) was determined as the weight ratio between fresh mushrooms harvested in both crops and the dry substrate, expressed as a percentage.
Quantification of the n-acetyl-d-glucosamine content and reducing sugars
Substrates samples (10 g) were collected from both formulations at different incubation periods during G. frondosa vegetative growth (20, 30, 45, 60 and 75 days), and also at days 87 and 107 (both fruiting cycles) in oak sawdust/corn bran (the unique substrate in which the fungus fully developed its carpophores). Entire solid cultures were dried at 105°C, until constant weight, ground in a mortar, and stored until they were used for chitin determination. The fungal biomass content of dry-solid cultures was determined by n-acetyl-d-glucosamine (NAG) released from chitin after hydrolysis with 6 N HCl. Analytical grade NAG served as reference (Plassard et al. 1982). Biomass content was indirectly determined by measuring glucosamine in the solid substrate and recalculated to dry biomass concentration (mg fungal biomass per g of dry substrate), taking into account glucosamine content of G. frondosa mycelium grown in 100-ml Erlenmeyer flasks with 25 ml of medium, containing glucose (30 g/l), yeast extract (6 g/l), SO4Mg.5H2O (0.5 g/l), K2HPO4 (0.5 g/l). The water-soluble compounds were extracted by hydrolysis with hot water for 3 h (Tappi Methods 1993), the reducing sugars were determined in the filtrate by the Somogyi-Nelson method (Nelson 1944). Moisture levels in the substrates were determined after drying them at 90°C until constant weight. Total N (% in dry basis) of the substrates was determined by AOAC methods (2000).
Preparation of crude enzyme extracts
Soluble proteins were extracted by adding 20 ml of distilled water to 2.5 g of substrate. Thirty min ultrasound-disrupting extraction was followed by 1 h centrifugation at 4,500 rpm and filtration. The resulting crude extracts were used for enzyme activities determination. All the steps for crude extraction were performed at room temperature. The supernatants were stored at −18°C until needed.
Determination of enzyme activities
Cellulolytic and xylanolytic enzyme activities were assayed at 50°C, while ligninases were determined at 30°C. Laccase (E.C.1.10.3.2) activity was determined by oxidation of 0.5 mM ABTS [2,2′azinobis(3-ethylbenzthiazoline-6-sulphonate)] in 0.1 M sodium acetate buffer pH 3.6 (ε420 = 36/mM cm) (Bourbonnais et al. 1995). Mn-peroxidase (MnP) (E.C.1.11.1.13) activity was determined by oxidation of phenol red (0.01%). The reaction product was measured at 610 nm (ε610 = 22/mM cm). The reaction mixture contained 0.05 M sodium succinate buffer pH 4.5, 0.1 mM MnSO4, 0.1 mM H2O2 (Glenn and Gold 1985). Lignin-peroxidase (E.C.1.11.1.14) (LiP) activity was assayed using Azure B as substrate (Archibald 1992). Endo-β-D-1,4-glucanase (E.C.3.2.1.4), exo-β-D-1,4-glucanase (E.C.3.2.1.91), endo-β-D-1,4-xylanase (E.C.3.2.1.8) and amylase activities were determined measuring the reducing sugars produced after hydrolysis of the substrate by the Somogyi-Nelson method (Nelson 1944). Amylases (a scientific term that collectively refers to α-amylase, β-amylase and glucoamylase) are enzymes that hydrolyze starch molecule to give diverse products including dextrin and progressively smaller polymers made up of glucose units (Pandey et al. 2000b). Measurements were made in 0.1 M sodium acetate buffer, pH 4.8, using the following substrates: carboxymethylcellulose 0.5% for endoglucanase; crystalline cellulose 1% for exoglucanase; xylan from oat-spelts 0.2% for endoxylanase (Wood and Bhat 1988) and soluble starch 2% for total amylase activity (Teller 1950). β-glucosidase (E.C.3.2.1.21) activity was determined measuring the product released from hydrolysis of p-nitrophenyl- β-D-glucopyranoside (0.02%) in sodium acetate buffer (pH 4.8). The reaction was stopped by adding Clark and Lubs buffer (pH 9.8), and absorbance was measured at 430 nm (ε430 = 18.5/mM cm) (Wood and Bhat 1988). Standard curves with glucose (for endoglucanase, exoglucanase and amylase) and xylose (for endoxylanase) were made to estimate enzyme activities. Enzyme activity is expressed in International Units (U), as the amount of enzyme needed to release 1 μmol of product per min. Enzymatic activities in the crude extracts recovered from the solid-state cultures were reported in U/ml. In terms of production, the activity was defined as U per g dry residue (substrate plus mycelium) (U/g). The same unique non-specific enzymes and mechanisms that give white-rot fungi the ability to degrade lignin also allow them to degrade a wide range of pollutants, among them dyes rendering as a product a colorless substance. LiP activity is responsible for Azure B degradation; therefore plates containing this dye were used to assess this activity (Archibald 1992). For qualitative LiP determination G. frondosa was inoculated on agar plates containing malt extract (12.7 g/l), glucose (10 g/l) and agar (20 g/l) (MEA) supplemented with Azure B (50 μM). Inoculum consisted of a 25-mm2 surface agar plug from a 7-day-old culture grown on MEA. Un-inoculated plates served as controls for abiotic decoloration. The plates were incubated at 28°C for 28 days. A decolorized zone appeared when the fungus degraded the Azure B dye.
Quantification of lignin and carbohydrates in the substrates during solid state fermentation
Changes in lignin and carbohydrates content of the substrates during SSF were determined. Substrate samples were dried at 90°C until constant weight; water-soluble compounds were extracted by hot water hydrolysis (Tappi Methods 1993), and later filtered. The retained solid was dried at 90°C for 24 h, and water soluble materials (among them starch) were determined by weight difference. To determine lignin, this solid was subsequently hydrolyzed using sulphuric acid (72%), rendering lignin as an insoluble residue (Tappi Methods 1998), while dissolving the carbohydrates (cellulose and hemicelluloses). The acid insoluble lignin was filtered off dried and then weighed. Carbohydrates content was calculated by weight difference.
Quantification of Zn content in the substrates
After heating to ash, the samples were digested with HCl 50%, evaporated to dryness, cooled, redissolved in HCl 50% and filtered with hot distilled water, finally bring them up to a volume of 25 ml. Zn content was determined at 213,9 nm by atomic absorption spectrophotometry (AOAC methods 2000).
Effect of zinc on G. frondosa growth
To investigate the effect of zinc on G. frondosa growth in agar plates, Petri dishes (90 mm diameter) containing PDA medium supplemented with 25, 100, 250 or 500 ppm of ZnCl2 or ZnSO4, initial pH 5.5, were inoculated and incubated at 25°C for 21 days. Radial growth was measured in two perpendicular directions from the edge of the inoculum to the advancing margin of the colony. The radial growth rates (mm per day) were determined.
Ligninolytic enzyme production under submerged fermentation
Basal culture medium YPG: yeast extract 6 g, glucose 30 g, peptone 2 g, MgSO4.7H2O 0.5 g, H2KPO4 0.5 g, MnSO4.5H2O 0.2 g, distilled water up to 1 l. Alternatively, either CuSO4 0.1 mM or poplar sawdust 10 g/l were added to the medium. Incubation was carried out statically at 28°C in 125 ml Erlenmeyer flasks with 40 ml of medium, which were inoculated with a 25-mm2 surface agar plug from a 7-day-old colony grown on Bacto-agar 2%. Aliquots of the supernatant were collected aseptically during 28 days and were used as enzyme sources.
Statistical analysis
A completely randomized two-way experimental design was applied. The first factor was the type of substrate at two levels (both substrate formulations), the second factor was the time at four levels (20, 30, 45, and 75 days of incubation), and the response variable was the enzyme activity (in U/g). Each treatment had ten replicas (10 substrates, 10 kg each, packed in bags of 1 kg).
Results and discussion
It has been well documented that composition of the substrate influences the pattern of enzyme production by white-rot fungi. Thus, the capacity of a particular substrate for inducing or increasing the production of lignocellulases is another factor that indirectly confers ability for growth and fruiting (Lechner and Papinutti 2006). This work characterized G. frondosa growth during SSF in two different substrates, determined the major components degraded by the fungus, and identified enzymes associated with medium degradation. To investigate its growth and basidiome development, SSF was performed by using oak sawdust plus corn bran as substrate during 107 days (last sampling day corresponds to second crop). A second formulation supplemented with coffee spent-ground was also evaluated for G. frondosa SSF during 75 days (fermentation time required for primordial induction). With oak sawdust plus corn bran we obtained a crop cycle time comparable to those previously reported by Shen and Royse (2001), consistent yields with combined high BE (35.3%), and good quality mushrooms [rated 1 on a scale of 1–4 (Shen and Royse 2002), where 1 is the highest quality: basidiomes with black to dark gray color, uniform and no misshapen pilei (Montoya-Barreto et al. 2008)]. Figure 1a–d display the kinetics of growth and lignocellulolytic enzymatic production in both formulations. Consumption of the main components of the two substrates is presented in Figs 1e, f. The growth was characterized by measuring NAG (released from chitin), and reducing sugars (Fig. 1a). The substrate was fully colonized after 25–30 days. Reducing sugars produced by enzymatic substrate hydrolysis, reached their highest value after the same period, and then declined sharply, probably consumed during G. frondosa growth. NAG content increased steadily for the first 87 days, to a maximum in coincidence with the first fruiting (60.5 μg NAG/mg dry sample). NAG content in the carpophore was 77.5 μg NAG/mg dry solid. As mycelium of G. frondosa contains 104 mg NAG/mg, actual biomass could be estimated, attaining a maximum of 0.58 mg/g dry solid. G. frondosa almost ran out of reducing sugars at approximately 85 days of fermentation. Since fungal growth also ended at this time point, it is suggested that the absence of carbon substrate limited further growth. Considering that total N (% in dry basis) increased from 0.23% in the un-inoculated oak sawdust plus corn bran substrate, to 0.48 at the last sampling day; and from an initial 0.7% up to 1.32 when coffee spent-ground was added, the cease of growth can’t be attributed to nitrogen depletion, nevertheless a concomitant deficiency of phosphates or micronutrients cannot be ruled out.
Growth (a, b), lignocellulolytic enzyme production (c, d), and content of fiber, lignin and carbohydrates in the substrates (e, f) during solid state fermentation of G. frondosa on oak sawdust plus corn bran (a, c, e) and oak sawdust plus corn bran supplemented with coffee spent-ground (b, d, f). a, b biomass (filled circles); dry weight (open circles); reducing sugars (filled triangles). c, d endoglucanase (filled squares), exoglucanase (open triangles), β-glucosidase (filled triangles), endoxylanase (open squares), amylase (open diamonds), laccase (filled circles) and MnP (open circles). e, f mass percentages of fiber (open triangles), lignin (filled squares) and carbohydrates (cellulose plus hemicellulose, filled triangles)
Many edible fungi developed basidiomes when using different coffee by-products for their cultivation (Fan et al. 2001; Job 2004; Velázquez-Cedeño et al. 2002; Wong and Wang 1991). In particular, coffee spent-ground demonstrated to be a good source for Flammulina velutipes, Pleurotus ostreatus and Lentinus edodes mushroom production (Fan et al. 1999, 2000, 2001; Job 2004). Nevertheless, the coffee supplemented substrate was adequate for G. frondosa mycelial growth [although lower yields were registered (maximum NAG content 46 μg/mg at day 75) (Fig. 1b)], but it did not develop mushrooms. Coffee spent-ground contains caffeine, tannins and polyphenols (Fan et al. 2001) the toxic nature of these compounds may affect basidiome development in G. frondosa. The individually effects of caffeine, tannins and phenols on basidiome development by G. frondosa will be the subject of our future investigations. There were not found literature reports concerning the action of G. frondosa on caffeine and tannins, but other edible fungi had already demonstrated their ability to degrade these compounds. Pleurotus sajor-caju, degraded up to 87% of the tannins when the fungus was cultivated in coffee spent-ground as solid substrate; L. edodes was also able to degrade its tannins (Wong and Wang 1991); P. ostreatus was able to develop fruiting bodies during SSF using several substrates containing up to 55% coffee spent ground (Job 2004), while F. velutipes not only developed edible mushrooms in this substrate, but also decreased its caffeine and tannins content (Fan et al. 2001). In views of the important differences detected when quantifying Zn concentration in the same substrates [17.3 ppm in oak substrate, and 290.25 ppm when the oak was supplemented with spent-ground coffee (Montoya-Barreto et al. 2008)], the lack of basidiome development could be also attributed to high heavy metals concentration, considering that although fungi require trace amounts of essential heavy metals such as Cd, Mn or Zn for their growth, these metals are toxic when present in excess, and can inhibit growth, cause morphological and physiological changes and affect the reproduction of basidiomycetes (Baldrian 2003). The effect of Zn on G. frondosa growth was tested in a concentration range from 25 to 500 ppm. Growth rates on PDA medium after 21 days were slightly affected by concentrations up to 100 ppm of ZnSO4 but decreased to 86 and 61% of the control with 250 and 500 ppm of Zn, respectively (data not shown). When ZnCl2 was used as source of Zn, growth inhibition was higher; a decrease to 64% and 34% of the control respectively with 250 and 500 ppm of Zn was detected. Longer lag-phases during the cultivation with 250–500 ppm of Zn were found (Fig. 2). Knowledge is currently very limited as to how various nutrient types and levels influence maitake crop cycle time, mushroom yield and quality due to the short history of its commercial cultivation. Previous results (Shen and Royse 2001) clearly indicated that not only the nutrient types but also their quantities influenced crop cycle time, yield and basidiome quality. The results obtained in this work extend previously published findings.
Grifola frondosa showed the capacity to degrade both substrates, causing a decrease of 67 and 50% in lignin content, and of 44 and 37% in polysaccharides (hemicellulose and cellulose) respectively for oak and oak/coffee substrates (Table 1).White-rot fungi like G. frondosa are active producers of cellulases and xylanases. It is widely known that they are not able to use lignin as the only source of carbon and energy (Kirk et al. 1976). The oxidation of lignin does not yield a net energy gain, thus lignin is not a substrate in primary metabolism. Rather, lignin is degraded during secondary metabolism in order to access wood polysaccharides locked in lignin-carbohydrate complexes, so providing an energy source to which other organisms do not have access (Jeffries 1990). But G. frondosa growing in SSF, produced hydrolases along with oxidases also during trophophase in parallel with growth (Figs. 1c,d). Cellulolytic, xylanolytic and ligninolytic activities were detected in the extracts recovered from the solid-state cultures. Highest activities achieved were: endoglucanase 12.3, exoglucanase 16.2, β-glucosidase 2.3, endoxylanase 20.3, laccase 14.8 and MnP 7.4 U/g dry substrate (Table 2). Although G. frondosa tested positive for Azure B plate degradation, a qualitative assay for LiP (Levin et al. 2004), attempts to detect this activity during SSF were unsuccessful. If produced in these substrates, LiP levels could be too low to be detected. On the other hand, the extraction procedure may have not been appropriate to guarantee the recovery of the whole enzymes adsorbed on the substrate. In oak substrate, as seen from Fig. 1c, all enzyme activities increased during the colonization phase, reaching a peak when the substrate was fully colonized at days 20–30. They gradually declined afterwards, but decreased markedly during fruiting body formation. Coffee addition not only decreased G. frondosa growth (characterized by chitin content and dry weight loss) (Fig. 1b), but also endoxylanase, endoglucanase and exoglucanase production (Fig. 1d); this can be attributed to toxic compounds such as caffeine, tannins and polyphenols or even high Zn concentrations present in coffee spent ground; which however, did not affect the synthesis of the other enzymes tested (ligninolytic enzymes and β-glucosidase). Most of the enzymes evaluated, displayed a similar pattern along the cultivation time. Highest activities were achieved in this medium at days 20–30, decreased steadily up to days 45–60, remaining almost constant afterwards (with the exception of endoxylanase which reached its lowest level at the end of the experience). β-glucosidase attained higher titers on days 20–30, showing later almost constant values up to day 75. During trophophase or primary metabolism, the fungus produces the extracellular enzymes necessary to degrade the substrate to soluble molecules that are transported to the intracellular space and stored as glycogen; later, NAG content increases to the final of the incubation period, reflecting probably the associate preparation for fruiting. The onset of fruiting is observable after a stimulus, as physiological stress, given at this moment (Lechner and Papinutti 2006). As in this study, in a previous work during SSF of G. frondosa using beech sawdust plus wheat bran and corn meal as substrates, laccase activity also increased during the colonization phase, reached a peak when the substrate was fully colonized and declined at the fruiting stage (Xing et al. 2006). Such profile is compatible with the assigned role of laccase in lignin degradation which is essential if the fungus is to gain access to the cellulose and hemicellulose components of the growth substrate. Hydrolysis of these polysaccharide materials provides the nutrients required for vegetative growth as a prelude to fruiting (Das et al. 1997). Ligninolytic enzymes have also repeatedly been linked to fruiting body production in higher basidiomycetes but species appear to differ at timings of high enzyme production and there is no clear-cut picture on functional relevance of the enzymes in fruiting (Kües and Liu 2000). In G. frondosa, lignocellulolytic enzymes appear to be produced for regenerative vegetative hyphal growth but not for fruiting body development. Similarly, high ligninolytic activities during growth phases in lignocellulosic substrates, with drastic reductions during the period of fruiting body formation, were observed in the white-rotting basidiomycetes P. ostreatus (Elisashvili et al. 2008; Rühl et al. 2008), Lentinus tigrinus (Lechner and Papinutti 2006), Lentinula edodes (Mata and Savoie 1998; Ohga and Royse 2001) and Agaricus bisporus (Wood and Goodenough 1977). In contrast, during Volvariella volvacea SSF laccase activity increase greatly after vegetative growth at fruiting body initiation and stay at high level until the fruiting bodies matured (Chen et al. 2003). If not also for nutritional reasons produced under an alternative regulation regime, enzyme production might be oxidative stress-related (Savoie et al. 2008) and/or the enzymes might have functions in fruiting body morphogenesis and pigmentation (Chen et al. 2003; Kües and Liu 2000). Laccase may also play a more direct role in the fruiting process (Chen et al. 2003), possibly by polymerizing cell wall components to cross-link hyphal walls during primordial formation and further strengthening of cell-to cell adhesion throughout fruit body development (Thurston 1994). The discussion on biological functions of laccases and peroxidases in relation to fruiting body development of basidiomycetes remains still controversial (Kües and Liu 2000; Rühl et al. 2008).
Pleurotus strains cultured on wheat straw presented more biological activity than strains cultured on coffee pulp. This result suggested that the complex chemical composition of the coffee pulp impeded its efficient conversion to mycelium. But laccase and MnP activities of Pleurotus strains on coffee pulp were higher than on wheat straw; implying their possible role in enzymatic detoxification (Salmones et al. 2005). In our study, although laccase and MnP production by G. frondosa was similar in both substrates assayed, and the results demonstrated that the fungus consumed lignocellulose, degradation percentages varied with each substrate. Higher carbohydrase (xylanolytic and cellulolytic) activities were in agreement with G. frondosa ability to grow more rapidly in oak substrate than in the coffee supplemented medium, consistent with increased dry weight loss, as well as lignin and polysaccharides consumption (Table 1). Moreover, it is likely that in oak/coffee substrate, the mushroom received nutrition and energy from the abundant free sugars that were present, and thus made limited use of the cellulose/hemicellulose fraction. The decrease in the amount of water soluble extractives in both substrates may be attributed in part to amylolytic enzyme production by G. frondosa, which reached its highest levels at day 20.
In this work G. frondosa demonstrated its ability to produce laccase (8 U/l) and MnP (12 U/l) under submerged fermentation in absence of inducers in a medium containing glucose, peptone and yeast extract, suggesting that the ligninolytic enzymes reported here could be constitutive. Laccase production increased with either sawdust or copper addition up to 30 and 1,255 U/l respectively. G. frondosa strain qx2 also produced MnP (3 U/l) and laccase (4.5 U/l) simultaneously when it was cultured on a low nitrogen asparagine succinic acid solution (Yin et al. 2010). Wood sawdust slightly enhanced both activities. Another strain of this fungus (FGF-1) produced laccase (70 U/l) in a liquid medium with glucose, asparagine, NH4NO3, and yeast extract (Xing et al. 2006). Results obtained show that, as most other ligninolytic fungal species (Baldrian 2006), G. frondosa produces constitutively at least one laccase isoenzyme.
In conclusion, the results achieved in this investigation contribute to expand the knowledge on the lignocellulolytic enzyme system of G. frondosa, scarcely investigated up till now. G. frondosa utilized an oak sawdust based medium as substrate for growth and fruiting, producing enzymes associated with its degradation such as endoglucanase, exoglucanase, β-glucosidase, endoxylanase, MnP and laccase. Coffee spent-ground was adequate as substrate for G. frondosa mycelial growth, but not for mushroom production. Caffeine, tannins, polyphenols or even high Zn concentrations could affect its growth, enzyme production and reproduction. However, taking into account that G. frondosa successfully colonized this residue, this substrate formula might be considered for its growth and polysaccharide production.
References
AOAC (2000) Official method of analysis of AOAC International, 17th edn. AOAC International, Gaithersburg
Archibald FS (1992) A new assay for lignin-type peroxidases employing the dye Azure B. Appl Environ Microbiol 58:3110–3116
Baldrian P (2003) Interactions of heavy metals with white-rot fungi. Enzyme Microb Technol 32:78–91
Baldrian P (2006) Fungal laccases-occurrence and properties. FEMS Microbiol Rev 30:215–242
Bourbonnais R, Paice MG, Reid ID, Lanthier P, Yaguchi M (1995) Lignin oxidation by laccase isozymes from Trametes versicolor and role of the mediator 2, 2-azinobis(3-ethylbenzthiazoline-6-sulfonate) in kraft lignin depolymerization. Appl Environ Microbiol 61:1876–1880
Chen SC, Ma D, Ge W, Buswell JA (2003) Induction of laccase activity in the edible straw mushroom, Volvariella volvacea. FEMS Microbiol Lett 218:143–148
Das N, Sengupta S, Mukherjee M (1997) Importance of laccase in vegetative growth of Pleurotus florida. Appl Environ Microbiol 63:4120–4122
Elisashvili V, Kachlishvili E, Penninckx MJ (2008) Lignocellulolytic enzymes profile during growth and fruiting of Pleurotus ostreatus on wheat straw and tree leaves. Acta Microbiol Immunol Hung 55:157–168
Eriksson KEL, Blanchette RA, Ander P (1990) Microbial and enzymatic degradation of wood and wood components. Springer-Verlag, Berlin
Fan L, Pandey A, Soccol C (1999) Cultivation of Lentinus edodes on the coffee industry residues and fruiting body production. In: Proceedings of the 3rd international conference on mushroom biology and mushroom products, Sidney, October 1999, pp 293–300
Fan L, Pandey A, Mohan R, Soccol CR (2000) Use of various coffee industry residues for the cultivation of Pleurotus ostreatus in solid state fermentation. Acta Biotechnol 20:41–52
Fan L, Pandey A, Soccol C (2001) Production of Flammulina velutipes on coffee husk and coffee spent-ground. Braz Arch Biol Technol 44:205–212
Glenn JK, Gold MH (1985) Purification and characterization of an extracellular Mn(II)-dependent peroxidase from the lignin degrading basidiomycete Phanerochaete chrysosporium. Arch Biochem Biophys 242:329–341
Illana-Esteban C (2008) El hongo maitake (Grifola frondosa) y su potencial terapéutico. Rev Iberoam Micol 25:141–144
Jeffries TW (1990) Biodegradation of lignin-carbohydrate complex. Biodegradation 1:163–176
Job D (2004) Use of coffee spent industry residues for production of Pleurotus ostreatus (Jacq.:Fr.) Kummer in solid state fermentation. Rev Iberoam Micol 21:195–197
Kirk TK, Connors WJ, Zeikus JG (1976) Requirement for a growth substrate during lignin decomposition by two wood-rotting fungi. Appl Environ Microbiol 32:192–194
Kües U, Liu Y (2000) Fruiting body production in basidiomycetes. Appl Microbiol Biotechnol 54:141–152
Lechner BE, Papinutti L (2006) Production of lignocellulosic enzymes during growth and fruiting of the edible fungus Lentinus tigrinus on wheat straw. Process Biochem 41:594–598
Levin L, Papinutti L, Forchiassin F (2004) Evaluation of Argentinean white rot fungi for their ability to produce lignin-modifying enzymes and decolorize industrial dyes. Bioresour Technol 94:169–176
Mata G, Savoie J-M (1998) Extracellular enzyme activities in six Lentinula edodes strains during cultivation in wheat straw. World J Microbiol Biotechnol 14:513–519
Montoya-Barreto S, Varón-López M, Levin L (2008) Effect of culture parameter on the production of the edible mushroom Grifola frondosa (maitake) in tropical weathers. World J Microbiol Biotechnol 24:1361–1366
Nelson NJ (1944) A photometric adaptation of the Somogyi method for the determination of glucose. J Biol Chem 153:375–380
Ohga S, Royse DJ (2001) Transcriptional regulation of laccase and cellulase genes during growth and fruiting of Lentinula edodes on supplemented sawdust. FEMS Microbiol Lett 201:111–115
Orth AB, Royse DJ, Tien M (1993) Ubiquity of lignin-degrading peroxidases among various wood-degrading fungi. Appl Environ Microbiol 59:4017–4023
Pandey A, Soccol CR, Nigam P, Brand D, Mohan R, Roussos S (2000a) Biotechnological potential of coffee pulp and coffee husk bioprocesses. Biochem Eng J 6:153–162
Pandey A, Nigam P, Soccol CR, Soccol VT, Singh D, Mohan R (2000b) Advances in microbial amylases. Biotechnol Appl Biochem 31:135–152
Plassard CS, Mousain DG, Salsac LE (1982) Estimation of mycelial growth of basidiomycetes by means of chitin determination. Phytochemistry 21:345–348
Rühl M, Fischer C, Kües U (2008) Ligninolytic enzyme activities alternate with mushroom production during industrial cultivation of Pleurotus ostreatus on wheat straw-based substrate. Curr Trends Biotechnol Pharm 2:478–492
Salmones D, Mata G, Waliszewski KN (2005) Comparative culturing of Pleurotus spp. on coffee pulp and wheat straw: biomass production and substrate biodegradation. Bioresour Technol 96:537–544
Savoie J-M, Minvielle N, Largeteau M (2008) Radical scavenging properties of extracts from the white button mushroom Agaricus bisporus. J Sci Food Agric 88:970–975
Shen Q, Royse D (2001) Effects of nutrient supplements on biological efficiency, quality and crop cycle time of maitake (Grifola frondosa). Appl Microbiol Biotechnol 57:74–78
Shen Q, Royse D (2002) Effects of genotipes of maitake (Grifola frondosa) on biological efficiency, quality and crop cycle time. Appl Microbiol Biotechnol 58:178–182
Svagelj M, Berovic M, Gregori A, Pahor B, Pohleven F (2007) Production of Grifola frondosa enzymes on solid-state brewery industry wastes. J Biotechnol 131:S240–S241
Tappi Methods (1993) Water solubility of wood and pulp T 207 om-93
Tappi Methods (1998) Acid-insoluble lignin in wood and pulp. T222 om-98
Teller JD (1950) Measurement of amylase activity. J Biol Chem 185:701–704
Thurston CF (1994) The structure and function of fungal laccases. Microbiology 140:19–26
Velázquez-Cedeño MA, Mata G, Savoie JM (2002) Waste reducing cultivation of Pleurotus ostreatus and Pleurotus pulmonarius on coffee pulp: changes in the production of some lignocellulolytic enzymes. World J Microbiol Biotechnol 18:201–207
Wong Y-S, Wang X (1991) Degradation of tannins in spent coffee grounds by Pleurotus sajor-caju. World J Microbiol Biotechnol 7:573–574
Wood T, Bhat K (1988) Methods for measuring cellulose activities. Methods Enzymol 160:87–112
Wood DA, Goodenough PW (1977) Fruiting of Agaricus bisporus: changes in extracellular enzyme activities during growth and fruiting. Arch Microbiol 114:161–165
Xing Z, Cheng J, Tan Q, Pan Y (2006) Effect of nutritional parameters on laccase production by the culinary and medicinal mushroom, Grifola frondosa. World J Microbiol Biotechnol 22:1215–1224
Yin L, Chi Y, Wang X (2010) Phylogenetic analysis and detection of the major ligninolytic enzymes system of Grifola frondosa. For Res. doi:CNKI:SUN:LYKX.0.2010-04-016
Yoshida M, Ohira T, Igarashi K, Nagasawa H, Samejima M (2002) Molecular cloning and characterization of a cDNA encoding cellobiose dehydrogenase from the wood-rotting fungus Grifola frondosa. FEMS Microbiol Lett 217:225–230
Acknowledgments
The authors thank Universidad de Caldas (Colombia), Universidad Nacional Sede Manizales (Colombia), Universidad de Buenos Aires and CONICET (Argentina) for financial assistance.
Author information
Authors and Affiliations
Corresponding author
Rights and permissions
About this article
Cite this article
Montoya, S., Orrego, C.E. & Levin, L. Growth, fruiting and lignocellulolytic enzyme production by the edible mushroom Grifola frondosa (maitake). World J Microbiol Biotechnol 28, 1533–1541 (2012). https://doi.org/10.1007/s11274-011-0957-2
Received:
Accepted:
Published:
Issue Date:
DOI: https://doi.org/10.1007/s11274-011-0957-2