Abstract
Considering changes in irrigation planning and development due to climate change is necessary to avoid system failure. This study demonstrated that changes in dependable flow and diversion water requirements in the future due to climate change will reduce potential irrigable areas. Climate change were based on the published projected climate in the study area. The dependable flow derived from successfully calibrated and validated Soil and Water Assessment Tool (SWAT) model streamflow simulations and the diversion water requirements based on the CROPWAT estimations of irrigation scheme were used to assess the potential irrigable areas. Substantial reductions in potential rice production areas (-4% to – 39%) were largely due to dwindled dependable flow (-1% to -25%) and an increase in diversion water requirement (+ 7% to + 26%). Reduction in potential irrigable area was projected during dry and normal years and may worsen towards the late twenty-first century under the worst-case climate scenario. Swelling of rivers during wet years will increase stream flows and potential irrigable areas but may also pose a danger of flooding. The development of water storage structures is necessary to reduce the adverse impacts of too much water during the wet years. Crop calendars should also be retrofitted to optimize the use of available rainfall during dry and normal years and climate-proof future irrigation systems. The results showed that it is necessary to incorporate climate change in irrigation planning and development. The methodologies described here could be used to climate-proof future irrigation systems in other areas in the Philippines and other countries.
Similar content being viewed by others
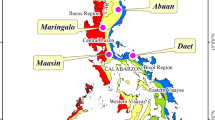
Avoid common mistakes on your manuscript.
1 Introduction
Inadequate projection of available water supply in water development plans is one of the causes of water shortages and the failure of irrigation systems. The actual irrigated areas in large irrigation systems in the country are also less than the design irrigation areas due to land change, flooding, sedimentation, and technical difficulties (Tabios and de Leon 2020). Water resource project planning and design issues, as well as inadequate operation and maintenance, that leads to early rehabilitation (Inocencio and Barker 2018). Physical targeting system for new irrigation development appears to be weak institutional, physical, and operational constraints (Inocencio and Briones 2020). In 2000, (David 2000) reported the alarming inefficiencies in the planning of the large and small irrigation development that leads to reaping less than half of its intended benefits. Bareng et al. (2015) benchmarked the overall system performance of large and small irrigation schemes and found that the selected irrigation systems are performing low and there are wide gaps between their actual and designed service areas. Moreover, climate change would make everything more difficult in agriculture to address food security in the country which results to relying unceasingly on rice imports. Thus, water development in agriculture needs to improve the productivity of available water resources amidst climate change (Inocencio and Barker 2018).
Information on irrigation development planning parameters such as dependable flow, irrigation water requirements, and potential irrigable areas could provide cost-effective support in irrigation project plans. The National Irrigation Administration defines potential irrigable areas as arable land in relatively flat areas with slopes from 0 to 3% that could be supplied with irrigation from water sources (National Irrigation Administration 2020). Studies considered areas with a slope of up to 8% potentially irrigable based on Food and Agriculture Organization (1976) framework for land evaluation (Worqlul et al. 2015, 2017; Nigussie et al. 2019). These studies employed the Geographic Information System to identify the areas that are potentially suitable for irrigation. In addition to the slope, other factors such as soil (drainage, depth, and texture), climate, the proximity of the rivers, and landuse were considered in spatially identifying land suitable for irrigation. The FAO classifies land as provisionally irrigable when it satisfies economic evaluation considering the water supply, development costs, and benefits (Food and Agriculture Organization 1985). These suitability assessment studies, however, are limited to the biophysical factors and did not consider the capacity of the rivers as a source of irrigation. More importantly, climate change was not included. Sustainability of water supplies is a huge challenge for water managers in the twenty-first century amidst global climate change on top of the continuously increasing demand brought by urbanization, industrialization, and population growth (Gleick 2003; Falkenmark 2008).
Climate change has taken its toll on rice production not only in the country but worldwide. Rice imports are beginning to be affected and would likely adversely hit the dependent countries. For example, Vietnam, the fourth largest rice exporter, would likely lessen rice exportation to other countries because of the reduced rice production areas due to salt intrusion attributed to sea level rise and rainfall and temperature changes (IFAD 2014; Yu et al. 2010; Baronchelli and Ricciuti 2018; Kontgis et al. 2019). Countries including the Philippines would be directly affected by these exportation cuts considering that the country also struggles with its rice self-sufficiency targets. Rice production in the uplands even with drought-resistant cultivars has been projected to substantially decrease because of changes in seasonal rainfall and increase in temperature brought by climate change (Alejo 2020). Lowland rice production is highly reliant on water availability thru irrigation systems. If the uplands are adversely affected by climate change, it might be worse in the lowlands.
Without major policy interventions, an increase in water demand in various sectors is projected to heighten competition among water users under decreasing water availability (Abansi et al. 2018). Efficient and effective water resources development in the country is suppressed by the lack of data and unreliable information on water supply and demand (Pulhin et al. 2018). Projections on irrigation water demand and supply would support climate-proofed irrigation development planning efficiently and effectively. Conventionally, irrigation designs do not consider climate change in the Philippines and in many developing countries. It is one of the reasons why many irrigation systems service areas are underirrigated (Bareng et al. 2015). This study presents a method in climate proofing future irrigation systems by incorporating climate projections in determining irrigation systems design parameters such as the dependable flow, diversion water requirement and potential service areas. It also used the latest available climate projections published by the weather authority in the Philippines. Climate change is dynamic and evolving and thus, it is important to update water resources projections with the latest available data.
There have been no published studies in the Philippines utilizing the latest representative concentration pathways (RCP) climate projections to examine climate change implications on streamflow and irrigation water requirements for irrigation planning and development. The RCP climate projections show the RCP for the dry, normal, and wet years in the mid and late twenty-first century. This is necessary to provide irrigation managers and the government a basis of what may happen in the future that could allow for the irrigation systems’ plans and designs to consider drought, flood, and normal climate conditions in the future. Araza et al. (2020) employed SWAT to model streamflow across climate change and landuse change scenarios, and approximated the potential irrigation supply as 81.3 percent of the streamflow. They calculated the possible irrigable area based on this. They used the RCP scenarios for climate change but did not consider the uncertatinties of dry, normal and wet years. It could be assumed that they used the normal year projections representing the mean values. Alejo and Ella (2019) evaluated the impacts of climate change on existing irrigation systems’ irrigable areas using the PRECIS-based climate projections for 2020 and 2050 published by PAGASA in 2011. The PRECIS-based climate projections only provided changes in rainfall and temperature as a seasonal average for two time slices for 2020 and 2050.
Since the present study was done for non-existing irrigation systems, validation of the results on potential irrigable areas was necessary to assess if such area is possibly available on the ground for lowland rice production. This paper theoretically validated the generated potential irrigable areas based on SWAT-based dependable flow and CROPWAT-based diversion water requirements using the GIS-based model framework. The study was conducted under Climate Type II which has a condition where rainfall could reach up to more than 5000 mm annually. The study assessed how climate changes affect the irrigation supply and demand of the future irrigation systems under areas with substantially abundant annual rainfall.
2 Methods
2.1 The Daet River Watershed
The Daet river is located in the southeastern part of the province of Camarines Norte in the Philippines. Its watershed has a total area of 4,174 hectares. (Fig. 1). Most of the watershed’s topography ranged from flat to nearly flat (0–3%) to rolling (9–18%) covering 65% of the total area. This is why 93% of the watershed area is devoted to agriculture, most of which is coconut plantations. Most of the Daet River watershed soil is loam (45%) to clay loam (55%). The Daet River watershed is under the Climate Type II of the Modified Corona classification system. The mean annual rainfall amounts to around 3,500 mm. Historically, annual rainfall ranged from 1,856 mm to as high as 5,418 mm. The last quarter of the year is the rainiest with more than 500 mm monthly rainfall. The average monthly temperature ranged from 26 °C in January to 28.9 °C from May to June.
To quantify the potential irrigable area for the baseline and all the climate change scenarios during the mid-21st and late-twenty-first century, the supply side and demand for lowland rice production were estimated (Fig. 2). The supply side or the available water for irrigation was determined from the calibrated and validated SWAT simulated streamflow. The demand side or the diversion water requirements across scenarios were obtained by incorporating efficiencies on the CROPWAT estimation of field irrigation requirements. The theoretical service area was determined considering the suitable land use, slope, and soil and then subtracting the built-up areas from the result. This was conducted to validate if the potential irrigable area based on dependable flows and diversion water requirement conforms to the theoretically available land for rice production. The succeeding sections provide a more detailed explanation of the steps in the methodological framework.
2.2 Estimation of Available Water Supply for Irrigation
The available water supply for irrigation refers to the streamflow with an 80 percent probability of exceedance, also known as the dependable irrigation flow. SWAT was used to determine the dependable flow under the baseline and climate change scenarios.
2.2.1 The SWAT Model
Soil and Water Assessment Tool is a semi-distributed process-based watershed modeling tool that was developed more than 30 years ago (Gassman et al. 2007). It estimates hydrological processes with time ranging from hourly to annual. It generates output from a spatial scale of hydrologic response unit to basin (Srinivasan et al. 1998).
Data Inputs
For SWAT to sufficiently generate outputs, local weather, landuse, soil, and elevation data are required. Weather data, which include rainfall, temperature, relative humidity, wind speed, and solar radiation were requested from the DOST- Philippine Atmospheric, Geophysical, and Astronomical Services Administration (PAGASA). Solar radiation data was not available. However, it was generated using the DSSAT program. The DSSAT program was also used as a data quality checker since it can scan errors through the scan button in its Weatherman module. It is also capable of filling in missing data including daily solar radiation. It utilizes the WGEN and SIMMETEO weather simulators based on actual data (Soltani and Hoogenboom 2003). Soil maps were requested from the Bureau of Soil and Water Management (BSWM). Landuse was the result of the reclassified landcover map, which was requested from the National Mapping and Resource Information Authority (NAMRIA). High resolution DEM (5 m by 5 m) was also requested from the NAMRIA.
There were no streamflow data at the exact location at the chosen watershed outlet as the proposed location of the dam along the Daet River. However, 5 years (1982–1986) streamflow data was available along the Daet river and another 5 years (2006–2010) at a nearby river, the Labo river. Limited hydrological data is one of the issues impending sustainable irrigation planning and development (Palao et al. 2013; Briones et al. 2016). The drainage-area ratio method was used to augment the Daet river flow data based on the Labo river flow data. This technique interpolates the ratio between streamflow data and drainage area of the watershed under study to that of a watershed nearby or with a similar hydro-meteorological condition. The length of the streamflow data was found adequate for hydrological studies. Based on the test of data sufficiency the length of years for the entire study should at least be 7 years. These streamflow data were used to optimize and localize the SWAT model for the Daet River watershed. The streamflow data was requested from the Department of Public Works and Highways (DPWH).
SWAT Calibration and Validation Process
Manual calibrations of SWAT were employed to initially yield a sufficient performance before carrying out the use of SWAT-CUP for auto-calibration of 18 parameters. SWAT models were created using the optimized 18 parameters. The most sensitive parameters among the 18 calibration parameters were chosen based on the sensitivity analysis module of SWAT-CUP. These are the most sensitive parameters for the site which were optimized further to have the final calibrated and validated SWAT projects.
The SWAT- Calibration and Uncertainty Procedures (CUP) was used for streamflow calibration. A recalibration was done the most sensitive parameters based on its initial run. The SWAT model's performance was assessed using the most widely used statistical indices (Moriasi et al. 2007). The Nash–Sutcliffe model efficiency (NSE), percent bias (PBIAS), RMSE-observations standard deviation ratio (RSR), and coefficient of determination (R2) were the indicators used. For a model to be deemed satisfactory, it has to produce an R2 (Santhi et al. 2002; Van Liew et al. 2003) and NSE (Nash and Sutcliffe 1970) more than 0.5, RSR of less than 0.70 and PBIAS is between + 25% and -25% (Gupta 2017).
The SWAT-CUP program provides uncertainty approximations from its 95PPU results which indicates the percentage of the best estimates. The uncertainties can be attributed from the model inputs. The p-factor and the r-factor reflect the uncertainties of the model. The p-factor is the percentage of observed streamflow which is adequately estimated by the model. The thickness of the 95PPU is characterized by the r-factor. A p-factor of more than 70% and r-factor of almost 1 is considered satisfactory (Abbaspour 2015).
2.3 Climate Change Scenarios
Climate change scenarios were created based on projections to assess the effects on dependable flow and irrigable land. The DOST-PAGASA provided the recent climate projections. Their downscaling techniques and other details are further detailed in Philippine Atmospheric Geophysical and Astronomical Services Administration (2018).
The changes in rainfall and the maximum and minimum temperature represent climate change in this study. The historical weather data in the study area are the basis of the climate parameter changes for the scenarios. Climate scenarios considered were the following:
-
1.
Moderate level (RCP 4.5) – mid-twenty-first century (2036–2065) – dry years
-
2.
Moderate level (RCP 4.5) – mid-twenty-first century – normal years
-
3.
Moderate level (RCP 4.5) – mid-twenty-first century – wet years
-
4.
Moderate level (RCP 4.5) – end of the twenty-first century– dry years
-
5.
Moderate level (RCP 4.5) – end of the twenty-first century– normal years
-
6.
Moderate level (RCP 4.5) – end of the twenty-first century– wet years
-
7.
High level of GHG (RCP 8.5) – mid-twenty-first century (2070–2099) – dry years
-
8.
High level of GHG (RCP 8.5) – mid-twenty-first century – normal years
-
9.
High level of GHG (RCP 8.5) – mid-twenty-first century – wet years
-
10.
High level of GHG (RCP 8.5) – end of the twenty-first century– dry years
-
11.
High level of GHG (RCP 8.5) – end of the twenty-first century– normal years
-
12.
High level of GHG (RCP 8.5) – end of the twenty-first century– wet years
These scenarios represent the sustained increasing emission patterns under medium and high greenhouse gas levels in the future if there will be no interventions. The climate change projections come as percentage changes in rainfall and increases in temperature in the coming mid-twenty-first century (2036–2065) and late twenty-first century (2070–2099). These climate projections were provided by the DOST-PAGASA which national mandate is to deliver weather updates, forecast and projections. They recommended 2 trajectories of GHGs in the future, the moderate level (RCP 4.5) and high level of GHG (RCP 8.5). The first being the most likely to happen in future and the second was suggested for impact studies. Both climate change scenarios have uncertainties in the future for the 2 time slices. These uncertainties were captured with the dry, normal, and wet years projections of changes in both time slices. This means that for the mid-twenty-first century between 2036 and 2065, and late-twenty-first century between 2070–2099, there are three scenarios that could happen namely dry (extreme drought), wet years (extreme rainfall conditions) and normal years (no extreme drought or extreme rainfall conditions). The percentile changes in rainfall and increas in temperature in the climate projections were used to alter rainfall and temperature in SWAT across each scenario. All the adjustments were relative to the local baseline data in the area and were inputted as percent changes (rainfall) and increase (temperature) in SWAT. The baseline period used was the year 2013. This year was chosen since there were no climate anomalies during this year (NOAA Climate Prediction Center 2019).
2.4 Calculation of Potential Irrigable Area
The ratio of dependable flow to diversion water requirement is the potential irrigable area. SWAT streamflow frequency analysis was used to determine the dependable flows for each scenario. The streamflow at 80% probability of exceedance was taken as the dependable flow for irrigation planning. The diversion water requirements for each scnerario were generated from the farm water requirement in CropWAT. The impacts of climate change on potential irrigable area were computed as the percent change between the values from each scenario and the baseline.
The FAO developed CropWAT program which was used to estimate the diversion water requirement in the potential service areas. Alejo et al. (2021) provided information on how CropWAT estimates net irrigation requirement and detailed estimation method of diversion water requirement. This part of the present study employed their method in estimating DWR wherein a 70% irrigation application efficiency was considered, which is the typically accepted value for water resources planning. A conveyance efficiency of 80% was assumed for the computation of diversion water requirements.
2.5 Theoretical Validation of Potential Irrigable Area
To validate if there will be enough design service area based on the derived potential irrigable areas, a GIS map on the theoretical service area was developed based on theoretical irrigable area considering the suitable soil, slope, and land use for rice production expansion. Land use such as the built-up areas, roads, water bodies, and existing irrigated service areas was considered as restrictions to rice production expansion. The built-up areas, roads, and water bodies were identified through remote sensing in Google Earth Engine by supervised classification. Training features were based on polygons and points determined using the USGS Landsat 8 TOA Reflectance (Orthorectified). The existing irrigated service areas were based on the map of the National Irrigation Administrations' irrigation development maps which were georeferenced and digitized to generate vector data of these areas. The suitable slope was derived from the digital elevation model reclassified into 2 slope ranges (0–8% and > 8%). Areas with a slope of 0–8% were considered to be potentially irrigable. The reclassified slope was converted into vector format and the 0–8% slope class was retained as a suitable slope for rice production. The suitable slopes were based on the BSWM soil map where unsuitable soils were subtracted. Suitable slope and soil were intersected where the built-up, roads, and water bodies were erased. Only continuous regions of equal to and more than 5 hectares were considered in the final maps, which were considered theoretical service areas. Also, theoretical areas were summed up to assess whether the potential irrigable area based on dependable flow and diversion water requirements are comparable or not. A theoretical area that is equal to or greater than the potential irrigable area could be deemed feasible for irrigation development projects.
3 Results and Discussion
Climate change will restrict rice cultivation regions in the future because of changes in dependable flow and diversion water requirements. The SWAT model for Daet river demonstrated a satisfactory estimate of streamflow for water resources planning. Based on the SWAT climate change simulations, the planned irrigation systems in study area will experience water scarcity for irrigation during dry years but it might experience flooding during wet years. During normal years, climate change is projected to increase dependable flow with an increase in RCPs. Across all climate change scenarios, the irrigation demand or diversion water requirement was expected to increase. As a result, during the wet years, the potential irrigable land is expected to shrink across scenarios. Theoretical validation demonstrated enough contiguous lands for rice production across scenarios.
3.1 SWAT Model Performance
Calibration of the SWAT model for the Daet River watershed showed adequate performance for streamflow estimation with a coefficient of determination value of 0.81, NSE value of 0.77, 12.17 PBIAS value, and an RSR of 0.48. The calibrated parameters have been validated sufficiently for streamflow with validation statistics of 0.90 R2, 0.83 NSE, 16.32 PBIAS, and 0.41 RSR value. The model sensitivity was found to be primarily affected by the CN2 and the LAT TTIME. The CN2 dominantly affected the surface runoff. Increasing CN2 led to increased surface runoff and decreased the values of lateral flow and return flow. The LAT_TTIME is the travel time of lateral flow determine by soil hydraulic characteristics. The ALPHA_BNK, CH_K2, GW_DELAY, SOL_K and the SOL_BD are among the sensitive variables in the model (Table 1). Shi et al. (2011) and Martínez-Salvador and Conesa-García (2020) also showed similar SWAT model sensitive parameters to streamflow. Running another SWAT-CUP auto-calibration using the most sensitive parameters slightly improved the performance of SWAT in estimating streamflow relative to the calibration using the 18 initial parameters. There was 82% of the actual streamflow data within the 95PPU as indicated by the p-factors with thickness or r-factor of 0.88 that also indicates the good performance of the model. Generally, the model underestimated the peak flows. The model performed better with low flows up to around 5 m3/s.
3.2 Impact of Climate Change on Dependable Flow
The baseline dependable flow in the Daet river is 1.18 m3/s from a 4,174 hectares watershed. The Daet River is located in a Climatic Type II, which has a high mean annual rainfall of more than 5000 mm. The dependable flow in the Daet river is projected to decrease in RCP4.5 mid-21st during normal years and across scenarios during dry years and seen to increase during wet years and the rest of normal years (Fig. 3a). Dependable flow in the Daet river is projected to lessen during dry years (-13% to -25%) and rise during wet years + 3% to 8%. During normal years, it is estimated to increase in almost all the scenarios except in RCP 4.5 during Mid twenty-first century. The decrease in dependable flows in dry years will intensify towards the end of the century. The dependable flow during normal years is observed to increase with RCP. The changes in dependable flows, both in dry and normal years, are directly related to the RCP during the mid-twenty-first century. Notably, the increment in dependable flow in the Daet River during the rainy years ranged from + 22% (1.44 m3/s) in the RCP4.5 mid-twenty-first century to + 34% (1.58 m3/s) in the RCP8.5 late-twenty-first century. Similarly, Rani and Sreekesh (2019) estimated climate change to negatively impact mean annual streamflow in a watershed in India by as much as 14.18%. They used trend analysis methods on the historical climate data to project climate changes. Viola et al. (2019) assessed the impacts of climate change on annual runoff in seasonally dry basins using a framework based on the Fu’s equation and found that climate change could lead up to 50% reduction in annual runoff which is a result of nonlinear interaction with rainfall reduction and increase in evapotranspiration.
Across all scenarios, the expected dependable flow showed a consistent trend similar to the baseline during normal years. This means that during typical years, climate change will have no negative impact on irrigation supply. Climate change, on the other hand, will restrict irrigation supply during dry years, notably in the late twenty-first century. Hence, the size and capacity of the planned irrigation system should be considered in order to control excess rainfall during the wet years. This way, the irrigation system could store more to supply irrigation during dry years and could store more to suppress flooding downstream. The estimated streamflow during wet years should be selected for this purpose. The selection, however, depends on many criteria which includes available financial, physical and institutional resources.
3.3 Impact of Climate Change on Diversion Water Requirement
The diversion water requirements derived from the CROPWAT outputs of field irrigation schemes are presented in Fig. 3b. The diversion water requirement (DWR) ranged from 1.43 to 1.80 li/s/ha (0.00143 to 0.0018 m3/s/ha). Remarkably, despite the high annual volume of rainfall, the DWR under this climate type was seen to only escalate across years and scenarios. Compared to the DWR in the baseline period, climate change is seen to consistently increase the DWR across scenarios. Climate change will cause a doubling of diversion water requirements of future irrigation systems in the Philippines (Alejo et al. 2021). Across all scenarios, diversion water requirement in the site will increase with RCP and towards the late twenty-first century. These findings show that future irrigation systems will require increased water supply even in areas with significant rainfall resources. To mitigate the effects of climate change on crops in Central Iran, Mehrazar et al. (2020) proposed modifying cropping patterns as one of their simulated water demand interventions. Retrofitting the cropping patterns will be a good practice in future irrigated lowland rice systems to optimize the availability of rainfall resources.
3.4 Climate Change Impacts on Potential Irrigable Areas
Climate change could inflict unfavorable impacts on potential irrigable areas. Climate change will result in significant decrease of future irrigable lands, owing to increased irrigation demand. The potential irrigable area could shrink by -9% (511 hectares) to -39% (503 hectares) during the dry years in the RCP8.5 late-twenty-first century relative to the 826 hectares in the baseline period (Fig. 3c). The lowest possible reduction in the potential irrigable area during dry years is -19% (671 hectares). Under normal years, climate change can cause a reduction in the potential irrigable area that could range from -4% in the RCP8.5 mid-twenty-first century to -18% in the RCP4.5 mid-twenty-first century. The wet years could have an increase in potential irrigable areas that may range from + 5% (867 hectares) in the RCP4.5 late-twenty-first century to + 22% (1005 hectares) in the RCP 8.5 late-twenty-first century. Araza et al. (2020) also used SWAT to simulate streamflow in a Climate Type III in the Philippines and estimated the potential irrigation water as 81.3% of the total flow. From this, they estimated the potential irrigable area. Using this method, they found that 19.13% of crop lands are at risk due to climate change and land use change during May–August cropping seasons. Climate Type III is characterized by no pronounced dry and wet seasons, with relatively dry from November to April and wet the rest of the year.
Despite plentiful annual rainfall, this analysis highlighted how susceptible future irrigation systems under Climate Type II are to climate change. This might also imply that additional rainfall inputs in a watershed do not always indicate more irrigable land that can be supplied. However, appropriate water management strategies are needed to optimize water available. During wet years, potential irrigable areas will expand due to enough dependable flows. These findings show that in irrigation planning and development, design service areas should be carefully defined to avoid overestimation of such parameters in the designs. This will help to prevent future lowland rice irrigation regions from being under-irrigated. The designed service area for the planned dam could be selected among the potential irrigable area projections in this study. To avoid underirrigation during droughts, projections from dry years should be considered. However, selection depends on many factors including the funds available, and the water conservation management that could be implemented and included in the plans. Assessment of irrigation development program in the country generally showed many existing small and large communal and national irrigation systems prevalent overestimation of designed service areas and under irrigation of lowland rice production areas (Luyun and Elazegui 2020). This study is pertinent and important now that the government is depending on significant irrigation expansion to accomplish the country's aim of inclusive growth and a globally competitive economy by 2040.
3.5 Theoretical Service Areas
Irrigation supply and demand has been calculated at this stage, however it is based on the river's capacity as well as crop and field consumptive use. For irrigation development to be feasible in an area, it is necessary to assess on the ground if the potential irrigable areas are available on actual ground. Assessment results suggest that the theoretical service areas based on suitable slope, soil, and landuse for rice production expansion were found to be larger than their corresponding potential irrigable areas across all scenarios. The site had 3,371 hectares theoretical service area that is yet to be irrigated and is 4 times larger than the potential irrigable area of 826 hectares from the baseline and 503 to 1005 hectares across climate change scenarios.
The irrigation supply and demand have been accounted for, however, these are based on the capacity of the river and crop and field consumptive use. For irrigation development to be feasible in an area, it is necessary to assess if the potential irrigable areas are available on the ground. Assessment results demonstrated that the theoretical service areas based on suitable slope, soil, and landuse for rice production expansion were found to be larger than their corresponding potential irrigable areas across scenarios. This suggests that there is available contiguous land for rice production areas with a size more than the irrigation capacity of the Daet river.
4 Conclusion
Climate change will lead to a reduction in irrigation supply and an increase in irrigation demand. This will further lead to a reduction in potential irrigable areas in future irrigation systems. With the current crop calendar and crop management, results demonstrated that up to 39% of future irrigation systems in rice production areas could be under irrigated largely due to declines in irrigation supply of up to -39% and an increase in irrigation demand of up to + 26% in areas. Hence, assessment of the optimum crop calendar across climate change scenarios to optimally use rainfall resources is highly suggested to come up with a climate-proofed crop calendar. Water storage facilities with sizes and designs that account climate change are required to prevent floods during wet years and store enough irrigation supplies in preparation for dry years. The methods herein demonstrated for assessing climate change impacts on irrigation planning and development parameters could be extended for use in other potential lowland rice production areas for development not only across the country but also across other nations.
Availability of Data and Materials
All the data are available upon request in responsible agencies.
References
Abansi CL, Hall RA, Siason IML (2018) Water demand management and improving access to water. Glob Issues Water Policy 8:233–259. https://doi.org/10.1007/978-3-319-70969-7_11
Abbaspour KC (2015) SWAT‐CUP: SWAT calibration and uncertainty programs - a user manual
Alejo LA (2020) Assessing the impacts of climate change on aerobic rice production using the DSSAT-CERES-Rice model. J Water Clim Change. https://doi.org/10.2166/wcc.2020.286
Alejo LA, Ella VB (2019) Assessing the impacts of climate change on dependable flow and potential irrigable area using the SWAT model. The case of Maasin River watershed in Laguna, Philippines. J Agric Eng 50:88–98. https://doi.org/10.4081/jae.2019.941
Alejo LA, Ella VB, Lampayan RM, Delos Reyes AA (2021) Assessing the impacts of climate change on irrigation diversion water requirement in the Philippines. Clim Change 165:1–17. https://doi.org/10.1007/s10584-021-03080-6
Araza A, Perez M, Cruz RVO et al (2020) Probable streamflow changes and its associated risk to the water resources of Abuan watershed, Philippines caused by climate change and land use changes. Stoch Env Res Risk Assess. https://doi.org/10.1007/s00477-020-01953-3
Bareng JLR, Balderama OF, Alejo LA (2015) Analysis of irrigation systems employing comparative performance indicators: A benchmark study for national irrigation and communal irrigation systems in Cagayan River Basin. J Agric Sci Technol A 5:325–335. https://doi.org/10.17265/2161-6256/2015.05.003
Baronchelli A, Ricciuti R (2018) WIDER Working Paper 2018/86: Climate change, rice production, and migration in Vietnamese households
Briones RU, Ella VB, Bantayan NC (2016) Hydrologic impact evaluation of land use and land cover change in Palico Watershed, Batangas, Philippines Using the SWAT model. J Environ Sci Manag 19:96–107
David WP (2000) Constraints, opportunities and options in irrigation development
Falkenmark M (2008) Water and sustainability: A reappraisal. Environment 50:4–16
Food and Agriculture Organization (1976) A framework for land evaluation, FAO Soils. Food and Agriculture Organization of the United Nations, Wageningen, Netherlands
Food and Agriculture Organization (1985) Evaluating and classifying land for irrigated agriculture. In: Guidelines: Land evaluation for irrigated agriculture, FAO soils. Soils Resources, Management and Conservation Service FAO Land and Water Development Division, Rome, Italy
Gassman PW, Reyes MR, Green CH, Arnold JG (2007) The soil and water assessment tool : Historical development, applications, and future research directions. Trans ASAE 50:1211–1250. https://doi.org/10.13031/2013.23637
Gleick PH (2003) Global freshwater resources: Soft-path solutions for the 21st century. Science 302:1524–1528
Gupta RS (2017) Hydrology and hydraulic systems, 4th edition
Inocencio A, Barker R (2018) Current challenges in agricultural water resource development and management in the Philippines. DLSU Bus Econ Rev 28:1–17
Inocencio AB, Briones RM (2020) Irrigation investments: Some recurrent and emerging issues
International Fund for Agricultural Development (2014) Vietnam’s rice production threatened by climate change
Kontgis C, Schneider A, Ozdogan M et al (2019) Climate change impacts on rice productivity in the Mekong River Delta. Appl Geogr 102:71–83. https://doi.org/10.1016/j.apgeog.2018.12.004
Luyun RA, Elazegui DD (2020) Assessing the resurgent irrigation development program of the Philippines-communal irrigation systems component
Martínez-Salvador A, Conesa-García C (2020) Suitability of the SWAT model for simulating water discharge and sediment load in a Karst Watershed of the Semiarid Mediterranean Basin. Water Resour Manag 34:785–802. https://doi.org/10.1007/s11269-019-02477-4
Mehrazar A, Massah Bavani AR, Gohari A et al (2020) Adaptation of Water Resources System to Water Scarcity and Climate Change in the Suburb Area of Megacities. Water Resour Manag 34:12 34:3855–3877. https://doi.org/10.1007/S11269-020-02648-8
Moriasi DN, Arnold JG, van Liew MW et al (2007) Model Evaluation Guidelines for Systematic Quantification of Accuracy in Watershed Simulations. Transactions of the ASABE 50:885–900. https://doi.org/10.13031/2013.23153
Nash JE, Sutcliffe JV (1970) River flow forecasting through conceptual models part I-A discussion of principles. J Hydrol 10:282–290. https://doi.org/10.1016/0022-1694(70)90255-6
National Irrigation Administration (2020) Status of irrigation development. In Definition of Technical Terms. http://region11.nia.gov.ph/?q=node/11. Accessed 17 Mar 2020
Nigussie G, Moges MA, Moges MM, Steenhuis TS (2019) Assessment of suitable land for surface irrigation in ungauged catchments: Blue Nile Basin. Ethiopia Water 11:1465. https://doi.org/10.3390/w11071465
NOAA Climate Prediction Center (2019) Historical El Nino/La Nina episodes (1950-present). Historical El Nino/ La Nina episodes. https://origin.cpc.ncep.noaa.gov/products/analysis_monitoring/ensostuff/ONI_v5.php. Accessed 1 Mar 2019
Palao LKM, Dorado MM, Anit KPA, Lasco RD (2013) Using the soil and water assessment tool (SWAT) to assess material transfer in the layawan watershed, Mindanao, Philippines and its implications on payment for ecosystem services. J Sustain Dev 6:73–88. https://doi.org/10.5539/jsd.v6n6p73
Philippine Atmospheric Geophysical and Astronomical Services Administration (2018) Observed climate trends and projected climate change in the Philippines. Quezon City, Philippines
Pulhin J, Ibabao R, Rola A, Cruz R (2018) Water supply and demand and the drivers of change. Glob Issues Water Policy 8:15–40. https://doi.org/10.1007/978-3-319-70969-7_2
Rani S, Sreekesh S (2019) Evaluating the responses of streamflow under future climate change scenarios in a Western Indian Himalaya Watershed. Environ Process 6:155–174. https://doi.org/10.1007/S40710-019-00361-2
Santhi C, Arnold JG, Williams JR et al (2002) Validation of the SWAT model on a large river basin with point and nonpoint sources. J Am Water Resour Assoc (JAWRA) 37:1169–1188
Shi P, Chen C, Srinivasan R et al (2011) Evaluating the SWAT model for hydrological modeling in the Xixian Watershed and a comparison with the XAJ Model. Water Resour Manag 25:2595–2612. https://doi.org/10.1007/s11269-011-9828-8
Soltani A, Hoogenboom G (2003) A statistical comparison of the stochastic weather generators WGEN and SIMMETEO. Climate Res 24:215–230. https://doi.org/10.3354/cr024215
Srinivasan R, Ramanarayanan TS, Arnold JG, Bednarz ST (1998) Large area hydrologic modeling and assessment part II: Model application. J Am Water Resour Assoc 34:91–101. https://doi.org/10.1111/j.1752-1688.1998.tb05962.x
Tabios GQ, de Leon TZ (2020) Assessing the Philippine irrigation development program. PIDS Policy Notes 2020–02
Van Liew MW, Arnold JG, Garbrecht JD (2003) Hydrologic simulation on agricultural watersheds: Choosing between two models. Trans ASAE 46:1539–1551
Viola F, Feng X, Caracciolo D (2019) Impacts of Hydrological Changes on Annual Runoff Distribution in Seasonally Dry Basins. Water Resour Manag 2019 33:7 33:2319–2333. https://doi.org/10.1007/S11269-019-02250-7
Worqlul AW, Collick AS, Rossiter DG et al (2015) Assessment of surface water irrigation potential in the Ethiopian highlands: The Lake Tana Basin. CATENA 129:76–85. https://doi.org/10.1016/j.catena.2015.02.020
Worqlul AW, Jeong J, Dile YT et al (2017) Assessing potential land suitable for surface irrigation using groundwater in Ethiopia. Appl Geogr 85:1–13. https://doi.org/10.1016/j.apgeog.2017.05.010
Yu B, Zhu T, Breisinger C, Hai NM (2010) Impacts of climate change on agriculture and policy options for adaptation the case of Vietnam
Acknowledgements
The DOST – Engineering Research and Development for Technology, the DOST – Science Education Institute, the DOST – Philippine Council for Agriculture, Aquatic and Natural Resources Research and Development, and the Department of Agriculture – Bureau of Agricultural Research funded and supported this research. The authors would also like to thank the technical data support of the following agencies: Department of Public Works and Highways, National Mapping and Resource Information Authority, Philippine Atmospheric, Geophysical and Astronomical Services Administration, Isabela State University, and World Wide Fund for Nature-Philippines, as well as Ms. Marissa H. Valdez for proofreading this paper.
Funding
This research was funded and supported by the DOST – Engineering Research and Development for Technology, DOST- Science Education Institute, DOST- Philippine Council for Agriculture, Aquatic and Natural Resources Research and Development, and Department of Agriculture-Bureau of Agricultural Research.
Author information
Authors and Affiliations
Contributions
L.A. Alejo conceptualized and designed the study, as well as supervised and prepared the article. A.S. Alejandro gathered data, conducted analysis, and participated and contributed in the preparation and writing of the article.
Corresponding author
Ethics declarations
Ethical Approval
None of the authors conducted any human or animal experiments for this publication.
Consent to Participate
Not applicable.
Consent to Publish
Not applicable.
Competing Interests
The authors have no relevant financial or non-financial interests to disclose.
Additional information
Publisher's Note
Springer Nature remains neutral with regard to jurisdictional claims in published maps and institutional affiliations.
Rights and permissions
About this article
Cite this article
Alejo, L.A., Alejandro, A.S. Changes in Irrigation Planning and Development Parameters Due to Climate Change. Water Resour Manage 36, 1711–1726 (2022). https://doi.org/10.1007/s11269-022-03105-4
Received:
Accepted:
Published:
Issue Date:
DOI: https://doi.org/10.1007/s11269-022-03105-4