Abstract
Five room temperature ionic liquids (ILs), 1-butyl-3-methylimidazolium hexafluorophosphate (L-P104), 1-hexyl-3-methylimidazolium hexafluorophosphate (L-P106), 1-octyl-3-methylimidazolium hexafluorophosphate (L-P108), 1-decyl-3-methylimidazolium hexafluorophosphate (L-P110), and 1-hexyl-3-methylimidazolium tetrafluoroborate (LB106) were studied as 1 wt% additives of polyurea grease for steel/steel contacts. Their tribological behaviors as additives of polyurea grease for steel/steel contacts were evaluated on an Optimol SRV-IV oscillating reciprocating friction and wear tester and an MRS-1J (G) four-ball tester at room and high temperatures. The friction test results showed that the ILs, as 1 wt% additives in polyurea grease for steel/steel contacts, had better friction reduction and anti-wear properties at high temperature than at room temperature, and ILs can significantly improve the friction reduction and anti-wear properties of polyurea grease compared with base grease containing 1 wt% of zinc dialkyldithiophosphate (T204). The excellent tribological properties are attributed to the formation of a surface protective film composed of FeF2, nitrides, and compound containing the P–O bonding on the lubricated metal surface by a tribochemical reaction. The ordered adsorbed films and good miscibility of ILs with the base grease also contributed to the excellent tribological properties. Wear mechanisms and worn steel surfaces were studied by a PHI-5702 multifunctional X-ray photoelectron spectrometer and a JSM-5600LV scanning electron microscope.
Similar content being viewed by others
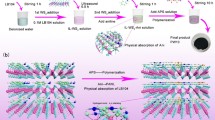
Avoid common mistakes on your manuscript.
1 Introduction
Recognized as the most promising variety of grease in the 21st century, polyurea grease has a wide operating temperature range and excellent oxidation, thermal, mechanical, and colloidal stabilities [1–3]. Used mainly for a variety of high temperature lubrication equipment, it serves to reduce friction and wear between two surfaces in relative motion. Additives with high thermal stability ensure that the grease is not affected in the course of the performance. Therefore, developing new additives suitable for high temperature greases is important.
Recently, ionic liquids (ILs) have attracted considerable interest because of their favorable physical properties, such as negligible volatility, nonflammability, high thermal stability, and low melting point [4, 5]. These attributes are essential to an ideal lubricant. Because of the excellent performance of ILs, they are good candidates for high-performance lubricants [6–31]. Many reports have also been done on IL additives. Phillips and Zabinski [32] studied ILs as additives in water and found that this could improve lubrication and antiwear (AW) behavior. Jimenez and Bermudez studied the tribological properties of several ILs as additives in paraffinic–naftenic mineral base oil and synthetic esters and found that highly polar ILs could reduce both friction and wear with respect to the base oil [33–35]. Yao et al. [36, 37] synthesized and evaluated several imidazolium ILs as anti-wear additives in poly(ethylene glycol). Their results show that these could effectively reduce the friction and wear of sliding pairs compared with cases in which additives were not used.
In the studies discussed above, ILs have been used as lubricants and additives in base oils with good results. Fox et al. studied ILs as additives in grease gave surprisingly large increases in the weld load for the four-ball extreme pressure test [38]. However, in addition to research work by Fox et al., alkyl imidazolium ILs as additives in grease is still relatively small. In this paper, the tribological behavior of five imidazolium ILs, 1-butyl-3-methylimidazolium hexafluorophosphate (L-P104), 1-hexyl-3-methylimidazolium hexafluorophosphate (L-P106), 1-octyl-3-methylimidazolium hexafluorophosphate (L-P108), 1-decyl-3-methylimidazolium hexafluorophosphate (L-P110), and 1-hexyl-3-methylimidazolium tetrafluoroborate (LB106), have been studied as 1 wt% friction reduction and anti-wear additive in polyurea grease for steel/steel contacts. The IL additives were compared to the zinc dialkyldithiophosphate (T204), which is normally used in grease as a friction reducing and AW additive, but it is a sulfur and phosphorus containing compound that may pose pollution to environment.
2 Experimental
2.1 Chemicals
The ILs evaluated in this work were synthesized by a previously reported method [39]. Their structure and properties are shown in Table 1. Zinc dialkyldithiophosphate (T204) was purchased from LuBoRun Lanzhou LanLian Additive Co., Ltd. Polyurea grease was obtained from Lanzhou Petrochemical Grease Factory. The performance parameters for polyurea grease are shown in Table 2. All other chemicals used in this work were of analytical grade.
2.2 Characterization
Mixtures of the base grease and additives were mixed thoroughly before testing. Briefly, the grease was combined with 1 wt% of ILs. For full dissolution, 99 wt% of the polyurea grease was then added for grease mechanical agitation by mechanical stirrer at room temperature. The concoction was mixed under nitrogen for 5 h.
The density, viscosity, and viscosity–temperature index of the ILs were measured by a SVM3000 Stabinger viscometer (Table 1). The thermal behavior of the ILs was studied on a STA 449 C Jupiter® simultaneous thermogravimetry and differential scanning calorimetry (TG–DSC) from ambient temperature to 800 °C at a heating rate of 10 °C/min in air. The morphologies and chemical compositions of the worn surfaces were analyzed by a JSM-5600LV scanning electron microscope (SEM) and a PHI-5702 multifunctional X-ray photoelectron spectrometer (XPS) using Al Kα radiation as the excitation source. The binding energies of the target elements were determined at a pass energy of 29.35 eV with a resolution of about ±0.3 eV and using the binding energy of contaminated carbon (C1s: 284.6 eV) as reference.
2.3 Copper Strip Test
The test was performed by the GB/T7326-87 procedure. A piece of bright finish copper was immersed within a certain amount of the mixture. This was heated for 24 h at 100 °C. At the end of the test cycle, the copper was washed and compared with the corrosion standard tint board.
2.4 Tribological Tests
The tribological behaviors of the ILs as additives of polyurea grease for steel/steel contacts were evaluated on an Optimol SRV-IV oscillating reciprocating friction and wear tester and an MRS-1J (G) four-ball tester.
2.4.1 SRV Friction and Wear Tests
The Optimol SRV-IV oscillating reciprocating friction and wear test was performed at room temperature and at 150 °C with a ball-on-block configuration. Contact between the frictional pairs was achieved by pressing the upper running ball (diameter 10 mm, AISI 52100 steel) with a hardness of about 59–61 HRC against the lower stationary disc (ø 24 × 7.9 mm, AISI 52100 steel) with a hardness of about 63–67 HRC at a frequency of 25 Hz, a sliding amplitude of 1 mm, and a relative humidity of 15–25% for a duration of 30 min. The wear volume of the lower disc was measured by a MicroXAM 3D noncontact surface mapping profiler. Three repetitive measurements were performed for each type of disc, and the average values are reported in this paper.
2.4.2 Four-Ball Friction and Wear Tests
The four-ball test was performed in a ball-on-ball configuration by oscillating an AISI52100 steel ball (diameter, 12.7 mm) over three AISI52100 steel balls (diameter, 12.7 mm each) under the following conditions: rotating rate, 1450 rpm; frequency, 33.5 Hz; duration, 30 min; load, 392 N; temperatures, 20 and 75 °C. The wear scar diameters (WSD) on the three lower balls were measured using an optical microscope, and the friction coefficients were recorded automatically through the computer with a data-acquiring system linked to the four-ball tester.
3 Results and Discussion
3.1 Copper Strip Test
Results of the copper strip test of 1 wt% IL additives are listed in Table 3. After the test, the color of the copper strip exhibited only a mild discoloration. No corrosion generation and copper corrosion control standard shade guide was used to determine the corrosion level of 1b. The copper strip test results indicated that ILs qualified as additives in polyurea grease.
3.2 SRV Friction and Wear Tests
3.2.1 Room Temperature Tests
Figure 1 shows the variation of mean friction coefficients and wear volume of the sliding discs under lubrication of polyurea grease plus 1 wt% T204, 1 wt% L-P104, 1 wt% L-P106, 1 wt% L-P108, 1 wt% L-P110, and 1 wt% LB106 at room temperature (20 °C). The friction coefficients of polyurea grease with additives decreased as the load increased. Under low loads (100–200 N), the friction coefficient of the seven kinds of lubricants were almost similar. Under high loads (300–500 N), however, the friction coefficients of 1 wt% T204 were higher than the other six lubricants. Figure 1b shows that the wear volumes of the steel discs lubricated with polyurea grease containing 1 wt% T204 is 1.5 times more than that of polyurea grease containing 1 wt% ILs at all loads. The chain length of the ILs did not significantly influence the tribological performance. A decrease of wear was not observed when 1 wt% LB106 was used as an additive of the polyurea grease under similar conditions. The absence of wear reduction could be due to the effect of different anions. Results show that polyurea grease with IL additives had better anti-wear properties than the commonly used additive, T204.
The SEM micrographs of the worn surfaces of the steel discs lubricated by polyurea grease and three additives at 300 N and 20 °C are shown in Fig. 2. The worn steel surface under 1 wt% T204 lubrication showed a much wider and deeper wear scar than the surface lubricated with polyurea grease. The steel lubricated with polyurea grease showed only slight deep and narrow grooves. The wear scar of the steel discs lubricated by 1 wt% LB106 was relatively shallow, and scuffing was greatly alleviated. In marked contrast, for steel lubricated with 1 wt% L-P106, the width of the wear scars were smaller, and the abrasions became more smooth (Fig. 2e, f). This result is consistent with the wear volume results in Fig. 1b. Images of the worn steel surfaces lubricated by 1 wt% L-P104, 1 wt% L-P108, and 1 wt% L-P110 were not given because these appeared similar to L-P106.
SEM morphologies of the worn steel surfaces lubricated by polyurea grease and different additives: a, b polyurea grease; c, d 1 wt% T204; e, f 1 wt% L-P106; g, h 1 wt% LB106 (the magnification of the images on the left is ×80 and on the right, it is ×300; load: 300 N, stroke: 1 mm, frequency: 25 Hz, duration: 30 min, temperature: 20 °C)
3.2.2 High Temperature Tests
3.2.2.1 Effect of Load
The effect of the load on the tribological behavior of polyurea grease containing 1 wt% T204, 1 wt% L-P104, 1 wt% L-P106, 1 wt% L-P108, and 1 wt% L-P110 are shown in Fig. 3. Figures 1 and 3 show the results of testing under the same conditions as that in Fig. 1 but at a temperature of 150 °C. The friction coefficients of polyurea grease with additives decreased as the load increased, and the wear volumes of the steel discs lubricated by all the lubricants increased as the load increased. The IL additives showed excellent tribological performance. The friction coefficients of IL additives were better than that of polyurea grease with 1 wt% T204 about 20% lower. The wear volumes of the steel discs remained lower using IL additives than 1 wt% T204 for polyurea grease at all loads. The IL additives were registered with a load-carrying capacity that is greater than 500 N at both 20 and 150 °C. This load-carrying capacity was much higher than that of polyurea grease alone (400 N).
Figure 4 shows the SEM micrographs of the worn surfaces of steel discs lubricated by polyurea grease, 1 wt% T204, and 1 wt% L-P106 at 300 N and 150 °C. All the SEM images were obtained under the same conditions. As shown in Fig. 4c and f, the worn surface lubricated with 1 wt% L-P106 was smooth, and only slight grooves can be seen, demonstrating its favorable anti-wear properties. The wear scar of the steel discs lubricated by polyurea grease containing 1 wt% T204 show a wide worn surface and a relatively slight adhesive wear with fewer grooves. The worn steel surface under polyurea grease lubrication showed a much wider and deeper wear scar than that lubricated with polyurea grease containing 1 wt% L-P106 and 1 wt% T204, in which severe adhesive wear with some deep and narrow grooves were observed. This is consistent with the measured wear volume results in Fig. 3b. The SEM micrographs of worn surfaces of the steel discs lubricated by 1 wt% L-P104, 1 wt% L-P108, and 1 wt% L-P110 were similar to that of L-P106. Thus, the data is not shown here.
SEM morphologies of the worn surfaces lubricated by polyurea grease and different additives: a, d polyurea grease; b, e 1 wt% T204; c, f 1 wt% L-P106 (the magnification of the images on the left is ×80 and that at the right is ×300; load: 300 N, stroke: 1 mm, frequency: 25 Hz, duration: 30 min, temperature: 150 °C)
After SRV friction and wear tests, the IL additives in polyurea grease were shown to have better excellent tribological properties for steel/steel contacts at high temperature (150 °C) than at room temperature (20 °C). This may be attributed to high thermal stability and better high temperature mobility of ILs.
3.2.2.2 Effect of Frequency
Figure 5 shows a frequency ramp test amplified from 10 Hz up to 35 Hz in 5 Hz intervals. The test was conducted under a load of 200 N for six kinds of additives and polyurea grease at 150 °C. The test duration for each frequency was 5 min. All the lubricants experienced a running-in period, and the five kinds of IL additives exhibited lower friction coefficients than 1 wt% T204 and polyurea grease during a frequency ramp test from 10 to 35 Hz.
a Evolution of friction coefficient with time during a frequency ramp test from 10 to 35 Hz for polyurea grease with different additives at 150 °C. b Wear volumes of steel discs lubricated by polyurea grease plus 1 wt% T204, 1 wt% L-P104, 1 wt% L-P106, 1 wt% L-P108, 1 wt% L-P110, and 1 wt% LB106 at 150 °C. c Wear scar diameter of steel balls lubricated by polyurea grease with different additives at 150 °C (load: 200 N, stroke: 1 mm, frequency: 10–35 Hz)
The wear volumes and friction diameters were also investigated, and the results are shown in Fig. 5b and c, respectively. The wear volumes of the lubricants increased in the following sequence: 1 wt% ILs < 1 wt% T204 < polyurea grease. The wear scar diameter of the steel balls increased in the order of: 1 wt% ILs < 1 wt% T204 < polyurea grease. However, the wear volumes of the worn discs and WSD in all the samples using IL additives were quite similar to those of the steel balls, except in the case of LB106, which showed slightly higher wear volumes and scar diameters in the disc. The good tribological performance of the ILs has been attributed to the polarity of their molecules and their ability to form ordered adsorbed layers [30].
3.3 Four-Ball Friction and Wear Tests
3.3.1 Room Temperature Tests
A standard four-ball test was also used to study the friction behavior of the additives in polyurea grease at 392 N and room temperature (20 °C). The results are shown in Fig. 6. The friction coefficient of the lubricants increased in the following sequence: 1 wt% ILs < 1 wt% T204|1 wt% L-P104 < polyurea grease. The WSD of the steels balls increased in the order of: 1 wt% ILs < 1 wt% T204 < 1 wt% L-P104 < polyurea grease. Except for 1 wt% L-P104, the rest of the ILs had lower friction coefficients and smaller WSD than 1 wt% T204. These data showed outstanding friction reduction and anti-wear properties, ILs are considered good additives for polyurea grease.
3.3.2 High Temperature Tests
The evolution of the friction coefficient with time at 392 N for polyurea grease with IL additives at 75 °C is shown in Fig. 7a. The five kinds of IL additives had lower friction coefficients than that of polyurea grease and 1 wt% T204. However, polyurea grease with 1 wt% T204 had a friction behavior that is comparable to polyurea grease. In these high temperature tests, polyurea grease exhibited an evolution of its friction coefficient with time, as characterized by relatively large fluctuations in the friction curve. Figure 7b shows that the WSD of the steels balls increased in the order of: 1 wt% ILs < 1 wt% T204 < polyurea grease. The four-ball friction and wear tests further proved that the ILs were good additives for polyurea grease, allowing for better tribological capability.
Four-ball friction and wear tests showed that the IL additives had better friction reduction and anti-wear properties at high temperature (75 °C) than at room temperature (20 °C). This is consistent with the results of the SRV friction and wear tests.
3.4 Surface Analysis
To gain further insights into the lubricating mechanism of ILs as additives in polyurea grease, the surface chemical composition was characterized by XPS (Fig. 8; Table 4). The binding energies of all the samples are given in Table 5. Figure 8A shows the XPS spectra of the steel block surface. Figure 8B (SRV at 150 °C) shows the XPS spectra of the worn steel disc surface lubricated by polyurea grease. Figure 8E, C, and D present the XPS spectra of neat L-P106 and the corresponding worn surface (SRV at 20 °C and 150 °C). Table 5 shows that the binding energies of Fe, O, F, and P of the worn surfaces lubricated by L-P106 at 20 °C were similar to those of L-P106 at 150 °C. Both samples differed from the binding energies of neat L-P106 and steel block surface. Thus, we can conclude that complicated tribochemical reactions were involved during the friction process. The peaks of F1s at about 685.5 and 684.6 eV, which might correspond to FeF2, appeared on the worn steel surface (http://www.srdata.nist.gov/xps/) [13]. The N1s spectra of the worn surface are very wide, indicating that the signal was generated by anime, analog, and/or nitrogen oxide [30]. The P2p peaks appeared at around 133.2 eV (Fig. 8C, D). After comparing the results with the standard spectrum of the element (http://www.srdata.nist.gov/xps/), we identified the presence of P at the worn surface, which could be assigned to P–O bonding. The XPS spectra of O1s (Fig. 8C, D) in the worn surface were very wide, indicating the generation of complex oxide species. Based on these data, we concluded that the IL additives in polyurea grease experienced complicated tribochemical reactions and generated a surface protective film composed of FeF2, nitrides, and compound containing the P–O bonding on the lubricated metal surface, which significantly contributed to the friction reduction and anti-wear properties.
4 Conclusions
Five room temperature ILs were studied as additives for polyurea grease in steel/steel contacts. The copper strip test results showed that the IL additives were not corrosive to copper, which fulfilled the requirement for a polyurea grease additive. The friction test results showed that the ILs, as 1 wt% additives in polyurea grease for steel/steel contacts, had better friction reduction and anti-wear properties at high temperature than at room temperature. This may be attributed to the high thermal stability, good solubility, and better high temperature mobility of the ILs. The XPS analysis showed that complicated tribochemical reactions were involved in the friction process and that a surface protective film composed of FeF2, nitrides, and compound containing the P–O bonding was generated on the lubricated metal surface. This significantly contributed to the friction reduction and anti-wear properties of IL additives in polyurea grease for steel/steel contacts. Thus, ILs has a potential use as additives in polyurea grease for the rolling bearings industry.
References
Wagh, S., Dhumal, S., Suresh, A.: An experimental study of polyurea membrane formation by interfacial polycondensation. J. Memb. Sci. 328, 246–256 (2009)
Roland, C., Twigg, J., Vu, Y., Mott, P.: High strain rate mechanical behavior of polyurea. Polymer 48, 574–578 (2007)
Sheth, J.P., Yilgor, E., Erenturk, B., Ozhalici, H., Yilgor, I., Garth, L., Wilkes, G.L.: Structure–property behavior of poly(dimethylsiloxane) based segmented polyurea copolymers modified with poly(propylene oxide). Polymer 46, 8185–8193 (2005)
Olivier, H.: Recent developments in the use of non-aqueous ionic liquids for two-phase catalysis. J. Mol. Catal. A 146, 285–289 (1999)
Hagiwara, R., Ito, Y.: Room temperature ionic liquids of alkylimidazolium cations and fluoroanions. J. Fluor. Chem. 105, 221–227 (2000)
Ye, C.F., Liu, W.M., Chen, Y.X., Yu, L.G.: Room-temperature ionic liquids: a novel versatile lubricant. Chem. Commun. 21, 2244–2245 (2001)
Zhou, F., Liang, Y.M., Liu, W.M.: Ionic liquid lubricants: designed chemistry for engineering applications. Chem. Soc. Rev. 38, 2590–2599 (2009)
Minami, I.: Ionic liquids in tribology. Molecules 14, 2286–2305 (2009)
Bermudez, M.D., Jimenez, A.E., Sanes, J., Carrion, F.J.: Ionic liquids as advanced lubricant fluids. Molecules 14, 2888–2908 (2009)
Torimoto, T., Tsuda, T., Okazaki, K., Kuwabata, S.: New frontiers in materials science opened by ionic liquids. Adv. Mater. 22, 1196–1221 (2010)
Liu, W.M., Ye, C.F., Gong, Q.Y., Wang, H.Z., Wang, P.: Tribological performance of room-temperature ionic liquids as lubricant. Tribol. Lett. 13, 81–85 (2002)
Liu, W.M., Ye, C.F., Chen, Y.X., Ou, Z., Sun, D.C.: Tribological behavior of sialon ceramics sliding against steel lubricated by fluorine-containing oils. Tribol. Int. 35, 503–509 (2002)
Wang, H.Z., Lu, Q.M., Ye, C.F., Liu, W.M., Cui, Z.J.: Friction and wear behaviors of ionic liquid of alkylimidazolium hexafluorophosphates as lubricants for steel/steel contact. Wear 256, 44–48 (2004)
Mu, Z.G., Liu, W.M., Zhang, S.X., Zhou, F.: Functional room-temperature ionic liquids as lubricants for an aluminum-on-steel system. Chem. Lett. 33, 524–525 (2004)
Jin, C., Ye, C.F., Phillips, B.S., Zabinski, J.S., Liu, X.Q., Liu, W.M., Shreeve, J.M.: Polyethylene glycol functionalized dicationic ionic liquids with alkyl or polyfluoroalkyl substituents as high temperature lubricants. J. Mater. Chem. 16, 1529–1535 (2006)
Liu, X.Q., Zhou, F., Liang, Y.M., Liu, W.M.: Tribological performance of phosphonium based ionic liquids for an aluminum-on-steel system and opinions on lubrication mechanism. Wear 261, 1174–1179 (2006)
Qu, J., Truhan, J.J., Dai, S., Luo, H., Blau, P.J.: Ionic liquids with ammonium cations as lubricants or additives. Tribol. Lett. 22, 207–214 (2006)
Kamimura, H., Kubo, T., Minami, I., Mori, S.: Effect and mechanism of additives for ionic liquids as new lubricants. Tribol. Int. 40, 620–625 (2007)
Weng, L.J., Liu, X.Q., Liang, Y.M., Xue, Q.J.: Effect of tetraalkylphosphonium based ionic liquids as lubricants on the tribological performance of a steel-on-steel system. Tribol. Lett. 26, 11–17 (2007)
Jimenez, A.E., Bermudez, M.D.: Ionic liquids as lubricants for steel–aluminum contacts at low and elevated temperatures. Tribol. Lett. 26, 53–60 (2007)
Phillips, B.S., John, G., Zabinski, J.S.: Surface chemistry of fluorine containing ionic liquids on steel substrates at elevated temperature using Mössbauer spectroscopy. Tribol. Lett. 26, 85–91 (2007)
Suzuki, A., Shinka, Y., Masuko, M.: Tribological characteristics of imidazolium-based room temperature ionic liquids under high vacuum. Tribol. Lett. 27, 307–313 (2007)
Zeng, Z., Phillips, B.S., Xiao, J., Shreeve, J.M.: Polyfluoroalkyl, polyethylene glycol, 1,4-bismethylenebenzene, or 1,4-bismethylene-2,3,5,6-tetrafluorobenzene bridged functionalized dicationic ionic liquids: synthesis and properties as high temperature lubricants. Chem. Mater. 20, 2719–2726 (2008)
Minami, I., Kita, M., Kubo, T., Nanao, H., Mori, S.: The tribological properties of ionic liquids composed of trifluorotris(pentafluoroethyl) phosphate as a hydrophobic anion. Tribol. Lett. 30, 215–223 (2008)
Yu, B., Zhou, F., Pang, C.J., Wang, B., Liang, Y.M., Liu, W.M.: Tribological evaluation of α,\( \dot{\omega } \)-diimidazoliumalkylene hexafluorophosphate ionic liquid and benzotriazole as additive. Tribol. Int. 41, 797–801 (2008)
Pensado, A.S., Comunas, M.J.P., Fernandez, J.: The pressure–viscosity coefficient of several ionic liquids. Tribol. Lett. 31, 107–118 (2008)
Yao, M.H., Liang, Y.M., Xia, Y.Q., Zhou, F., Liu, X.Q.: High-temperature tribological properties of 2-substituted imidazolium ionic liquids for Si3N4-steel contacts. Tribol. Lett. 32, 73–79 (2008)
Zhang, L., Feng, D.P., Xu, B.: Tribological characteristics of alkylimidazolium diethyl phosphates ionic liquids as lubricants for steel–steel contact. Tribol. Lett. 34, 95–101 (2009)
Qu, J., Blau, P.J., Dai, S., Luo, H., Meyer III, H.M., Truhan, J.J.: Tribological characteristics of aluminum alloys sliding against steel lubricated by ammonium and imidazolium ionic liquids. Wear 267, 1226–1231 (2009)
Yao, M.H., Fan, M.J., Liang, Y.M., Zhou, F., Xia, Y.Q.: Imidazolium hexafluorophosphate ionic liquids as high temperature lubricants for steel–steel contacts. Wear 268, 67–71 (2010)
Minami, I., Watanabe, N., Nanao, H., Mori, S., Fukumoto, K., Ohno, H.: Improvement in the tribological properties of imidazolium-derived ionic liquids by additive technology. J. Synth. Lubr. 25, 45–55 (2008)
Phillips, B.S., Zabinski, J.S.: Ionic liquid lubrication effects on ceramics in a water environment. Tribol. Lett. 17, 533–541 (2004)
Jimenez, A.E., Bermudez, M.D., Iglesias, P., Carrion, F.J., Martınez-Nicolas, G.: 1-N-alkyl-3-methylimidazolium ionic liquids as neat lubricants and lubricant additives in steel–aluminium contacts. Wear 260, 766–782 (2006)
Jimenez, A.E., Bermudez, M.D., Carrion, F.J., Martınez-Nicolas, G.: Room temperature ionic liquids as lubricant additives in steel–aluminium contacts: influence of sliding velocity, normal load and temperature. Wear 261, 347–359 (2006)
Jimenez, A.E., Bermudez, M.D.: Imidazolium ionic liquids as additives of the synthetic ester propylene glycol dioleate in aluminium–steel lubrication. Wear 265, 787–798 (2008)
Yao, M.H., Liang, Y.M., Xia, Y.Q., Zhou, F.: Bisimidazolium ionic liquids as the high-performance antiwear additives in poly(ethylene glycol) for steel–steel contacts. ACS Appl. Mater. Interfaces 1, 467–471 (2009)
Zhang, H.B., Xia, Y.Q., Yao, M.H., Jia, Z.F., Liu, Z.L.: The influences of methyl group at C2 position in imidazolium ring on tribological properties. Tribol. Lett. 36, 105–111 (2009)
Fox, M.F., Priest, M.: Tribological properties of ionic liquids as lubricants and additives. Part 1: synergistic tribofilm formation between ionic liquids and tricresyl phosphate. Proc. Inst. Mech. Eng. J 222, 291–303 (2008)
Bonhote, P., Dias, A.P., Papageorgiou, N., Kalyanasundaram, K., Gratzel, M.: Hydrophobic, highly conductive ambient-temperature molten salts. Inorg. Chem. 35, 1168–1178 (1996)
Acknowledgments
The authors are grateful for the financial support provided by the “973” Program (2007CB607601), NSFC (50721602, 20533080), and the Chinese Academy of Sciences (KJCX2.YW.H16).
Author information
Authors and Affiliations
Corresponding authors
Rights and permissions
About this article
Cite this article
Cai, M., Zhao, Z., Liang, Y. et al. Alkyl Imidazolium Ionic Liquids as Friction Reduction and Anti-Wear Additive in Polyurea Grease for Steel/Steel Contacts. Tribol Lett 40, 215–224 (2010). https://doi.org/10.1007/s11249-010-9624-2
Received:
Accepted:
Published:
Issue Date:
DOI: https://doi.org/10.1007/s11249-010-9624-2