Abstract
A series of novel 1,2,3-thiadiazole derivatives were designed and synthesized. Their structures were characterized by 1H NMR, MS and HRMS. The bioactivity tests indicated that compound 9b exhibits a favorable KARI inhibition rate, and some of these novel compounds also showed moderate herbicidal activity against Brassica campestris.
Similar content being viewed by others
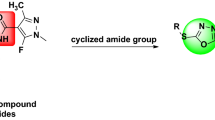
Avoid common mistakes on your manuscript.
Introduction
Recently, the development of herbicides targeting at the key enzymes in the biosynthesis pathway of branched chain amino acids has attracted extensive attention [1]. Some reported herbicides, such as sulfonylureas [2], imidazolinones [3], sulfonamide [4], and pyrimidinylbenzoic acids [5], can inhibit the first enzyme, acetohydroxyacid synthase (AHAS), in the biosynthesis pathway of the branched chain amino acids called. The success of these herbicides has stimulated numerous research into finding efficient inhibitors for other enzymes in this pathway, such as ketol-acid reductoisomerase (KARI; EC 1.1.1.86), which catalyzes the second key step. Furthermore, it was noticed that KARI only exists in microorganisms and plants, rather than in mammals, and thus it is an ideal target for non-toxic KARI-inhibitors for potential herbicide applications.
The KARI-catalyzed reaction in the biosynthesis of branched chain amino acids is shown in Fig. 1. The reactions include two steps [6]. Both steps require a divalent metal ion, such as Mg2+, Mn2+ or Co2+, and Mg2+ is known to be the specific metal ion for alkyl migration.
Until now, there has been many commercialized herbicide targeting at KARI, with some reported compounds including HOE 704 [7], IpOHA [8], 1,2,3-thiadiazoles [9, 10] and CPD derivatives [11–16] have shown certain KARI inhibitory activities, and might be the potential KARI inhibitors (Fig. 1).
Here, we report the synthesis, structural characterizations, and bioactivity tests of a series of novel 1,2,3-thiadiazole derivatives. Some of these compounds showed certain herbicidal and KARI inhibitory activities. Further density functional theory (DFT) studies showed the possible favorable functional groups of 1,2,3-thiadiazole derivatives.
Results and discussion
Chemistry
The synthetic route of the novel 1,2,3-thiadiazole derivatives is presented in Scheme 1. The key intermediate ethyl 4-(bromomethyl)-1,2,3-thiadiazole-5-carboxylate (6) was prepared via two pathways using carbonic acid diethyl ester (1) as the starting material. It is shown in Scheme 1. Ethyl 4-methyl-1,2,3-thiadiazole-5-carboxylate (4) was synthesized according to the reference. To a solution of ethyl 4-methyl-1,2,3-thiadiazole-5-carboxylate (4) (0.04 mol) in CCl4 (120 mL) was added NBS(7g) and a little AIBN, and the mixture was refluxed for 5 h. Afterwards, the reaction was completed, filtrated, washed with brine, dried over Na2SO4, and condensed under reduced pressure to afford the ethyl 4-(bromomethyl)-1,2,3-thiadiazole-5-carboxylate (6). This intermediate was not further purified.
The combination of H2O2 and HOAc has been recognized as an efficient system to oxidize sulfur into sulfone or sulfoxide. In our case, the sulfoxide was easily obtained at room temperature for 12 h. The longer the reaction time is given at room temperature, the more sulfone was obtained. When the mixture was stirred at room temperature for 48 h, all the sulfoxide converted to sulfone. The sulfone can also be obtained at reflux condition for short time.
Bioactivity and structure–activity relationship
To investigate the KARI inhibitory activities and herbicidal activities of the synthesized 1,2,3-thiadiazole compounds, cyclopropane-1,1-dicarboxylic acid (CPD), a potent inhibitor of KARI in vitro, was used as the reference. The bioactivities of the selected 1,2,3-thiadiazole compounds as well as CPD are shown in Table 1. With regard to the KARI inhibitory assay, most of the 1,2,3-thiadiazole compounds do not dissolve in the test system, except for the compound 8, 9, 11. The KARI inhibitory activities of these four compounds are lower than that of CPD, with the highest inhibitory activity of 60 % of compound 9b. Based on the herbicidal activities against Brassica napus and Echinochloa crusgalli tests, the synthesized 1,2,3-thiadiazole compounds displayed low activities. Compounds 8a, 9b and 10a exhibited moderate inhibitory activities against B. napus. According to the in vitro activities tests, the 1,2,3-thiadiazole compounds containing based carboxylic acid groups as well as the electron-withdrawing groups, such as phenyl, showed higher activity than their analogues. In addition, the sulfoxides led to higher activity than sulfonyls. In general, it was revealed that the ketol-acid reductoisomerase in vitro inhibitory activities of these new compounds are rather limited.
DFT study of structure–activity relationship
According to the frontier molecular orbital theory, HOMO and LUMO are two crucial factors influencing the bioactivities of compounds. HOMO is usually prone to provide electrons, while LUMO accepts electrons [16]. Based on such a concept, the study on the frontier orbital energy can provide useful information for the working mechanism of the bioactive compounds. DFT is a useful tool to investigate the HOMO–LOMO orbital energies. Thus, on the selected compound 9b with the highest KARI inhibitory activity, the DFT study was carried out using the DFT-B3LYP/6-31G method to find the favorable functionalities as well as the possible working mechanisms. The DFT-derived graphic results are presented in Fig. 2. The red and green parts represent the cloud density of frontier orbitals at HOMO or LOMO states. The total energy of the compound 9b is −1,901.67561163 a.u. The energy gap between HOMO and LUMO of the compound 9b is rather small (0.129 a.u.) suggesting that the stability of the compound 9b is limited. As seen from Fig. 2 of compound 9b in the HOMO, electrons are mainly delocalized on the phenyl ring, the carboxyl group as well as the sulfoxide group in compound 9b. However, when electron transitions take place, some electrons will enter into the LUMO, then, in the LUMO, the electrons will mainly be delocalized on the phenyl ring, carboxy group, SO2 group, and the 1,2,3-thiadiazole ring. Since these polar functionalities with favorable frontier molecular orbitals are the main positions where the interaction with KARI takes place, the possible working mechanism between compound 9b and the rice KARI amino acid residues is estimated to be the hydrophobic interactions.
Experimental
Materials and methods
All the reagents are analytical grade. Melting points were determined using an X-4 apparatus and uncorrected. 1H NMR spectra were measured on a Bruker AC-P500 instrument using TMS as an internal standard and CDCl3 as solvent. Mass spectra were recorded on a Thermo Finnigan LCQ Advantage LC/mass detector apparatus. Infrared spectra were recorded on a Nicolet MAGNA-560 spectrophotometer using KBr tablets. HRMS data was obtained on a FTICR-MS instrument (Ionspec 7.0T).
Synthesis
Synthesis of the intermediate ethyl 4-(chloromethyl)-1,2,3-thiadiazole-5-carboxylate (6)
Carbonic acid diethyl ester (1) (11.8 g, 0.1 mol) and hydrazine hydrate (5.6 mL, 0.095 mol, 85 %) were added into a 250-mL round-bottom flask equipped with a condenser. The reaction mixture was heated to 50 °C and stirred for 20 min, and then cooled to room temperature and further stirred for 30 h. Net, water, ethanol, and the unreacted carbonic acid diethyl ester were distilled off under reduced pressure. After drying, a white crystal (2) (9.88 g) was obtained with a yield of 95 %.
To a stirred solution of compound 2 (6.36 g, 0.06 mol) in ethanol (16.7 mL), a solution of ethyl 4-chloro-3-oxobutanoate (9.84 g, 0.06 mol) in ethanol (3.7 mL) was added at room temperature. The reaction mixture was stirred for 6 h. Then, the solvent was removed in vacuo and the crude product ethyl 2-(1-chloro-4-ethoxy-4-oxobutan-2-ylidene)hydrazinecarboxylate (3) was directly used in the next step without further purification.
Ethyl 2-(1-chloro-4-ethoxy-4-oxobutan-2-ylidene)hydrazinecarboxylate (3) (15.0 g, 0.06 mol) was dissolved in dry dichloromethane (25 mL), and thionyl chloride (25 mL) was subsequently added into the stirred reaction mixture drop wise at 0 °C for 1 h. Next, the reaction mixture was permitted to stand for 20 h at room temperature. The excess thionyl chloride and dichloromethane were distilled off, and the resulting crude product was subjected to fractional distillation under reduced pressure. An umber oil (4) was obtained with yield of 75.4 %.
Synthesis of the title compounds
General procedure for the compound 7a–7d
The synthetic route was shown in Scheme 1. To a stirred solution of 4 (1.05 g, 5.1 mmol) and K2CO3 (0.2 g, 5.6 mmol) in DMF (15 mL), a mixture of a substituted benzenethiol (5.1 mmol) was added dropwise. The resulting mixture was stirred at room temperature overnight. Next, the mixture was poured into water. The precipitate formed was filtered off. The crude product was purified by chromatography over silica gel to give 7a–7d. (PE:EA = 5:1).
General procedure for sulfoxides
To a stirred solution of compound 7b–7c (1 mmol) in HOAc (10 mL) was added H2O2 (2 mmol), then the mixture was stirred at room temperature overnight. When the reaction reached completion indicated by TLC, CH2Cl2 (20 mL) was added and the organic layer was separated and washed with water. Next, the solvent was evaporated in vacuo to afford crude compounds 8a–8c. The pure products 8a–8c were purified by chromatography over silica gel to give 8a–8c.
General procedure for sulfones
To a stirred solution of compound 7b–7c (1 mmol) in HOAc (10 mL) was added H2O2 (2 mmol), then the mixture was stirred at 80 °C for 1 h. During this course, a large amount of white solid was formed. After filtration, the solid was purified by chromatography over silica gel to give the pure 10a–10b.
General procedure for carboxylic acid
To a stirred solution of ester (1 mmol) in CH3OH (10 mL) was added NaOH (1 mmol) in CH3OH (5 mL), and the mixture was stirred at room temperature for 24 h. Next, diluted HCl solution was added dropwise to adjust pH to 7 and formed light yellow precipitates. After filtration, the pure product was obtained by recrystallization from EtOH/H2O mixture.
Ethyl 4-((phenylthio)methyl)-1,2,3-thiadiazole-5-carboxylate (7a): yellow crystal, yield 45.2 %, m.p. 28–30 °C; 1H NMR(CDCl3)δ: 1.36(t, J = 7.14 Hz, 3H, CH3), 4.33(q, J = 7.15 Hz, 2H, CH2), 4.80(s, 2H, SCH2), 7.20–7.24(m, 2H, Ar–H), 7.36–7.40(m, 2H, Ar–H); ESI–MS: 281.16[M+H]+; HRMS for C13H12N2O2S2: found 303.0227, calcd. 303.0232[M+Na]+.
Ethyl 4-((p-tolylthio)methyl)-1,2,3-thiadiazole-5-carboxylate (7b): reddish-brown crystal, yield 43.4 %, m.p. 36–37 °C; 1H NMR(CDCl3)δ: 1.35(t, J = 7.13Hz, 3H, CH3), 2.30(s, 3H, CH3), 4.30(q, J = 7.16Hz, 2H, CH2), 4.74(s, 2H, SCH2), 7.05(d, J = 8.12Hz, 2H, Ar–H), 7.23(d, J = 8.12Hz, 2H, Ar–H); ESI-MS: 295.10[M+H]+; HRMS for C13H14N2O2S2: found 317.0388, calcd. 317.0389 [M+Na]+.
Ethyl 4-(((4-chlorophenyl)thio)methyl)-1,2,3-thiadiazole-5-carboxylate (7c): reddish-brown crystal, yield 37.6 %, m.p. 41–43 °C; 1H NMR(CDCl3)δ: 1.37(t, J = 7.14 Hz, 3H, CH3), 4.34(q, J = 7.12 Hz, 2H, CH2), 4.78(s, 2H, SCH2), 7.22(d, J = 8.54 Hz, 2H, Ar–H), 7.30(d, J = 6.72 Hz, 2H, Ar–H); ESI-MS: 315.26[M+H]+; HRMS for C12H11ClN2O2S2: found 336.9846, calcd. 336.9843 [M+Na]+.
Ethyl 4-(((3-chlorophenyl)thio)methyl)-1,2,3-thiadiazole-5-carboxylate (7d): reddish-brown oil, yield 38.9 %; 1H NMR(CDCl3)δ: 1.40(t, J = 7.14 Hz, 3H, CH3), 4.38(q, J = 7.12 Hz, 2H, CH2), 4.84(s, 2H, SCH2), 7.21(d, J = 5.26 Hz, 2H, Ar–H), 7.28(s, 1H, Ar–H), 7.41(s, 1H, Ar–H); ESI-MS: 315.10[M+H]+; HRMS for C12H11ClN2O2S2: found 336.9839, calcd. 336.9843 [M+Na]+.
Ethyl 4-((p-tolylsulfinyl)methyl)-1,2,3-thiadiazole-5-carboxylate (8a): yellow solid, yield 31.2 %, m.p. 72–74 °C; 1H NMR(CDCl3)δ: 1.39(t, J = 7.17 Hz, 3H, CH3), 4.37(q, J = 7.07 Hz, 2H, CH2), 4.98(dd, J = 12.74 Hz, J = 12.74 Hz, 2H, SCH2), 7.39(d, J = 8.51 Hz, 2H, Ar–H), 7.45(d, J = 8.60 Hz, 2H, Ar–H); ESI-MS: 620.78[2M]+, 311.15[M+H]+; HRMS for C13H14N2O3S22: found 333.0336, calcd. 333.0336 [M+Na]+.
Ethyl 4-(((4-chlorophenyl)sulfinyl)methyl)-1,2,3-thiadiazole-5-carboxylate (8b): reddish-brown oil, yield 31.2 %; 1H NMR(CDCl3)δ: 1.38(t, J = 7.14Hz, 3H, CH3), 2.40(s, 3H, CH3), 4.36(q, J = 5.24 Hz, 2H, CH2), 4.95(dd, J = 12.70 Hz, J = 12.70 Hz, 2H, SCH2), 7.25(d, J = 8.20 Hz, 2H, Ar–H), 7.33(d, J = 8.20 Hz, 2H, Ar–H); ESI-MS: 662.69[2M]+, 331.07[M+H]+; HRMS for C12H11ClN2O3S2: found 352.9787, calcd. 352.9792 [M+Na]+.
4-((p-tolylthio)methyl)-1,2,3-thiadiazole-5-carboxylic acid (9a): yellow solid, yield 34.6 %, m.p. 139–141 °C; 1H NMR(CDCl3)δ: 2.31(s, 3H, CH3), 4.77(s, 2H, SCH2), 7.08(d, J = 7.98 Hz, 2H, Ar–H), 7.25(d, J = 7.98 Hz, 2H, Ar–H); ESI-MS: 530.79[2M]−, 264.92[M−H]−; HRMS for C11H10N2O2S2: found 265.0116, calcd. 265.0111[M−H]−.
4-(((4-chlorophenyl)thio)methyl)-1,2,3-thiadiazole-5-carboxylic acid (9b): yellow solid, yield 35.8 %, m.p. 142–143 °C; 1H NMR(CDCl3)δ: 4.79(s, 2H, SCH2), 7.25(d, J = 8.55Hz, 2H, Ar–H), 7.33(d, J = 8.55Hz, 2H, Ar–H); FTIR ν(KBr): 3020 cm−1, 2991 cm−1, 2940 cm−1, 1720 cm−1, 1513 cm−1, 1492 cm−1, 1415 cm−1, 1275 cm−1; ESI-MS: 570.78[2M]−, 284.79[M–H]−; HRMS for C10H7ClN2O2S2: found 384.9567, calcd. 284.9565 [M–H]−.
Ethyl 4-(tosylmethyl)-1,2,3-thiadiazole-5-carboxylate (10a): yellow solid, yield 78.0 %, m.p. 75–78 °C; 1H NMR(CDCl3)δ: 1.42(t, J = 6.80 Hz, 3H, CH3), 2.46(s, 3H, CH3), 4.41(q, J = 6.88 Hz, 2H, CH2), 5.29(s, 2H, SCH2), 7.21(d, J = 7.49 Hz, 2H, Ar–H), 7.60(d, J = 7.62 Hz, 2H, Ar–H); ESI-MS: 324.16[M–H]−, 327.17[M+H]+; HRMS for C13H14N2O3S2: found 327.0468, calcd. 327.0468 [M+H]+.
Ethyl 4-(((4-chlorophenyl)sulfonyl)methyl)-1,2,3-thiadiazole-5-carboxylate (10b): white solid, yield 83.2 %, m.p. 114–115 °C; 1H NMR(CDCl3)δ: 1.43(t, J = 7.16 Hz, 3H, CH3), 4.41(q, J =7.16 Hz, 2H, CH2), 5.32(s, 2H, SCH2), 7.51(d, J = 8.57 Hz, 2H, Ar–H), 7.67(d, J = 8.59 Hz, 2H, Ar–H); ESI-MS: 344.83[M−H]−; HRMS for C12H11ClN2O3S2: found 346.9916, calcd. 346.9922 [M+H]+.
4-((p-tolylsulfinyl)methyl)-1,2,3-thiadiazole-5-carboxylic acid (11): yellow solid, yield 31.3 %, m.p. 71–73 °C; 1H NMR(CDCl3)δ: 2.42(s, 3H, CH3), 4.90(dd, J = 12.56 Hz, J = 12.56 Hz, 2H, SCH2), 7.21(d, J = 3.77 Hz, 2H, Ar–H), 7.34(d, J = 8.21 Hz, 2H, Ar–H); ESI-MS: 281.06[M−H]−;
Theoretical calculations
On the basis of the structure of 9b, an isolated molecule was selected as the initial structure. DFT-B3LYP/6-31G (d,p) methods in Gaussian 03 package [17] were used to optimize the structure of 9b. Vibration analysis showed that the optimized structures were in accordance with the minimum points on the potential energy surfaces. All the convergent precisions were the system default values, and all the calculations were carried out on the Nankai Stars supercomputer at Nankai University.
KARI assay
Cloning, expression and purification of rice KARI
The DNA sequence corresponding to mature KARI was amplified by PCR using the oligonucleotide primers 5′-aaaggatCCATGGTCGCGGCGC-3′ and 5′-cccAaaTTtgaagcttCTACG ATGACTGCCGGAG-3′. In these sequences, lower case represents mismatched bases, underlining indicates the location of introduced BamHI and HindIII restriction sites, and italics show the Met-54 codon or the reverse complement of the TAG stop codon. The PCR product was digested with BamHI and HindIII and ligated into the pET-30a plasmid that had been digested with the same enzymes. The resultant expression plasmid was used to transform Escherichia coli BL21(DE3) cells.
A single colony of these cells was inoculated into 20 mL of LB medium containing 50 mg/mL kanamycin. The culture was incubated overnight at 37 °C and was used to inoculate each of two 500-mL volumes of LB medium containing 50 mg/mL kanamycin; the cultures were incubated at 37 °C with shaking. When an OD600 of 0.8 was reached, expression was induced by adding 0.5 mM isopropyl β-d-thiogalactoside to each culture; these were then incubated at room temperature (~22 °C) for a further 4 h with shaking and the cells were harvested by centrifugation.
The frozen cell pellet was thawed, suspended in ice-cold purification buffer [20 mM Tris–HCl (pH 7.9)/500 mM NaCl] containing 5 mM imidazole and then treated with lysozyme (10 mg/g of cells for 30 min at 0 °C). The cells were disrupted by sonication, insoluble material was removed by centrifugation and the supernatant was passed through a 0.45-mm filter. The cell extract was applied to a 7-mL column of His·Bind resin (Novagen) that had been charged by using 50 mM NiSO4 then equilibrated with purification buffer containing 5 mM imidazole. The loaded column was washed with 23 mL of the same buffer, followed by 30 mL of purification buffer containing 25 mM imidazole, and then KARI was eluted with 30 mL of purification buffer containing 400 mM imidazole. Fractions containing the enzyme were pooled, concentrated to 2.5 mL by ultrafiltration, and exchanged into 20 mM Na–Hepes buffer, pH 8.0 using a Pharmacia PD-10 column. The eluate was snap-frozen in liquid nitrogen and stored at −70 °C [18, 19].
Enzyme and protein assays
Gerwick et al. [19] reported that the inhibition of Escherichia coli KARI is time-dependent. To characterize the steady-state inhibition constant, Escherichia coli KARI was preincubated for 10 min with NADPH, Mg2+ and the title compound, then the reaction was initiated with hydroxypyruvate. Under these conditions, the change in A340 was found to be linear with time.
Herbicidal activities assay
Inhibition of the root growth of rape (Brassica campestris)
The evaluated compounds were dissolved in water and emulsified if necessary. Rape seeds were soaked in distilled water for 4 h before being placed on a filter paper in a 6-cm Petri plate, to which 2 mL of inhibitor solution had been added in advance. Usually, 15 seeds were used on each plate. The plate was placed in a dark room and allowed to germinate for 65 h at 28 ± 1 °C. The lengths of ten rape roots selected from each plate were measured, and the means were calculated. The check test was carried out in distilled water only. The percentage of the inhibition was calculated.
Inhibition of the seedling growth of Barnyard grass (Echinochloa crusgalli)
The evaluated compounds were dissolved in water and emulsified if necessary. A total of ten barnyard grass seeds were placed into a 50-mL cup covered with a layer of glass beads and a piece of filter paper at the bottom, to which 5 mL of inhibitor solution had been added in advance. The cup was placed in a bright room and allowed to germinate for 65 h at 28 ± 1 °C. The heights of seedlings of above-ground plant parts from each cup were measured, and the means were calculated. The check test was carried out in distilled water only. The percentage of the inhibition was calculated.
References
R.G. Duggleby, S.S. Pang, J. Biochem. Mol. Biol. 33, 1 (2000)
R.S. Chaleff, C.J. Mauvais, Science 224, 1443 (1984)
D.L. Shaner, P.C. Anderson, M.A. Stidham, Plant Physiol. 76, 545 (1984)
S. Hung, Ind. Chem. 11, 291 (1983)
S.J. Koo, S.C. Ahn, J.S. Lim, S.H. Chae, J.S. Kim, J.H. Lee, J.H. Cho, Pestic. Sci. 51, 109 (1997)
S.K. Chunduru, G.T. Mrachko, K.C. Calvo, Biochemistry 28, 486 (1989)
A. Schulz, P. Sponemann, H. Kocher, F. Wengenmayer, FEBS Lett. 238, 375 (1988)
A. Aulabaugh, J.V. Schloss, Biochemistry 29, 2824 (1990)
F. Halgand, F. Vives, R. Dumas, V. Biou, J. Andersen, J.P. Andrieu, R. Cantegril, J. Gagnon, R. Douce, E. Forest, D. Job, Biochemistry 37, 4773 (1998)
X.H. Liu, L. Pan, J.Q. Weng, C.X. Tan, Y.H. Li, B.L. Wang, Z.M. Li, Mol. Divers. doi:10.1007/s11030-011-9352-z
Y.T. Lee, H.T. Ta, R.G. Duggleby, Plant Sci. 168, 1035 (2005)
X.H. Liu, P.Q. Chen, B.L. Wang, Y.H. Li, Z.M. Li, Bioorg. Med. Chem. Lett. 17, 3784 (2007)
X.H. Liu, J.Q. Weng, C.X. Tan, L. Pan, B.L. Wang, Z.M. Li, Asian J. Chem. 23, 4031 (2011)
X.H. Liu, C.Y. Zhang, W.C. Guo, Y.H. Li, P.Q. Chen, T. Wang, W.L. Dong, B.L. Wang, H.W. Sun, Z.M. Li, J. Enzym. Inhib. Med. Chem. 24, 545 (2009)
X.H. Liu, Y.X. Shi, Y. Ma, C.Y. Zhang, W.L. Dong, P. Li, B.L. Wang, B.J. Li, Z.M. Li, Eur. J. Med. Chem. 44, 2782 (2009)
X.H. Liu, L. Pan, C.X. Tan, J.Q. Weng, B.L. Wang, Z.M. Li, Pest. Biochem. Physiol. 101, 143 (2011)
M.J. Frisch, G.W. Trucks, H.B. Schlegel, G.E. Scuseria, M.A. Robb, J.R. Cheeseman, J.A. Montgomery Jr, T. Vreven, K.N. Kudin, J.C. Burant, J.M. Millam, S.S. Iyengar, J. Tomasi, V. Barone, B. Mennucci, M. Cossi, G. Scalmani, N. Rega, G.A. Petersson, H. Nakatsuji, M. Hada, M. Ehara, K. Toyota, R. Fukuda, J. Hasegawa, M. Ishida, T. Nakajima, Y. Honda, O. Kitao, H. Nakai, M. Klene, X. Li, J.E. Knox, H.P. Hratchian, J.B. Cross, C. Adamo, J. Jaramillo, R. Gomperts, R.E. Stratmann, O. Yazyev, A.J. Austin, R. Cammi, C. Pomelli, J.W. Ochterski, P.Y. Ayala, K. Morokuma, G.A. Voth, P. Salvador, J.J. Dannenberg, V.G. Zakrzewski, S. Dapprich, A.D. Daniels, M.C. Strain, O. Farkas, D.K. Malick, A.D. Rabuck, K. Raghavachari, J.B. Foresman, J.V. Ortiz, Q. Cui, A.G. Baboul, S. Clifford, J. Cioslowski, B.B. Stefanov, G. Liu, A. Liashenko, P. Piskorz, I. Komaromi, R.L. Martin, D.J. Fox, T. Keith, M.A. AlLaham, C.Y. Peng, A. Nanayakkara, M. Challacombe, P.M.W. Gill, B. Johnson, W. Chen, M.W. Wong, C. Gonzalez, J.A. Pople, Gaussian 03, Revision C. 01, Gaussian, Inc., Wallingford CT, 2004
X.H. Liu, P.Q. Chen, B.L. Wang, W.L. Dong, Y.H. Li, X.Q. Xie, Z.M. Li, Chem. Biol. Drug Des. 75, 228 (2010)
B.C. Gerwick, L.C. Mireles, R.J. Eilers, Weed Technol. 7, 519 (1993)
Acknowledgments
This work was funded by National Natural Science Foundation of China (no. 21002090), The Key Innovation Team of Science and Technology in Zhejiang Province (2010R50018) and Scientific Research Fund of Zhejiang Education Department (Y201018479).
Author information
Authors and Affiliations
Corresponding author
Rights and permissions
About this article
Cite this article
Liu, XH., Zhao, WG., Wang, BL. et al. Synthesis, bioactivity and DFT structure–activity relationship study of novel 1,2,3-thiadiazole derivatives. Res Chem Intermed 38, 1999–2008 (2012). https://doi.org/10.1007/s11164-012-0521-1
Received:
Accepted:
Published:
Issue Date:
DOI: https://doi.org/10.1007/s11164-012-0521-1