Abstract
Studies of crop coefficients as a function of vegetation indices are reported less often for sugarcane than other crops because of the variation in crop evapotranspiration (ETc) and spectral response over the long growth period. In this study, the possibility of correlating crop coefficient (Kc) and ground based normalized difference vegetation index (NDVI) of a sugarcane crop was investigated based on 2 years field experiments conducted in 2015 and 2016 in semi-arid India. The Kc values for the full crop season were determined by the field water balance method and ground NDVI was estimated from spectral reflectance measurements using a field spectro-radiometer. The sugarcane Kc values for the tillering (development stage), grand growth (mid-season) and maturity stages (end season) were 0.70, 1.20 and 0.78, respectively. The results found that the Kc was 16.6% less than that suggested by FAO-56. The sugarcane NDVI ranged from 0.48 to 0.69 at the tillering stage and 0.69 to 0.93 in the grand growth stage. Unlike other crops, sugarcane NDVI at the maturity stage did not reduce from 0.85 even at harvest due to the continued production of fresh green leaves at the top of the plant. Regression equations were developed to estimate the seasonal distribution of Kc with NDVI as the dependent variables and ratio of days \(\left(\frac{t}{T}\right)\) after planting (t) to the total crop period (T) as the independent variable. The relationship between crop Kc and NDVI was characterized with 2nd order polynomial regression but correlation was moderately strong (r = 0.75, n = 315). Stronger correlations between Kc and NDVI were obtained by splitting growth period into the growth phase (r = 0.98, n = 245) and decline phase (r = 0.99, n = 70). The estimated Kc will be helpful for correcting irrigation scheduling of sugarcane in semi-arid conditions. The Kc-NDVI relationships for sugarcane investigated in this study are important for potential real time irrigation water management in the future.
Similar content being viewed by others
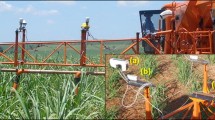
Avoid common mistakes on your manuscript.
Introduction
Sugarcane is a major agro-industrial crop and plays a vital role in the economy of India. India ranks 2nd after Brazil among sugarcane producing countries of the world and contributes 18.4% and 19% in area and production volume for the world, respectively (CACP 2019). However, a large proportion of Indian sugarcane is cultivated in semi-arid regions where irrigation is practiced but water availability can be a major limitation. This necessitates the need for continued improvements in sugarcane water use with a greater emphasis on the determination of actual crop evapotranspiration (ETc) during the growing period. Numerous approaches have been used to measure sugarcane evapotranspiration around the world (Thompson and Boyce 1971; Omary and Izuno 1995; Allen et al. 1998; Chabot et al. 2005; Silva et al. 2012; Win et al. 2014; Anderson et al. 2015; Cardoso et al. 2015). However, the crop coefficient (Kc)-based estimation of crop evapotranspiration (FAO-56 model) is one of the most commonly used methods for irrigation water management. Allen et al. (1998) suggested Kc values of 0.4, 1.25 and 0.75 for establishment, mid-season and at harvest for sugarcane; however, these values are based on global averages of Kc and thus, it is necessary to determine crop coefficient values for local conditions (Jagtap and Jones 1989).
Many studies have shown that remotely sensed spectral reflectance may provide an indirect estimate for Kc (Heilman et al. 1982; Bausch and Neale 1987; Bausch 1995).The similarities between the Kc and normalized difference vegetation index (NDVI) have potential for modelling Kc as a function of NDVI as both of them show a definite trend and are good indicators of the water stress at the canopy surface (Jackson and Huete 1992; Choudhury et al. 1994; Moran et al. 1995; Gontia and Tiwari 2010; Kamble et al. 2013).
The NDVI data can be routinely measured either on the ground from field radiometry, by aircraft measurements or by high and low resolution satellite images (Shibayama and Akiyama 1986; Wooten et al. 1999; Bastiaanssen et al. 2000; Hill and Donald 2003; Doraiswamy et al. 2004; Kastens et al. 2005; Mkhabela et al. 2005; Simoes et al. 2005; Raki et al. 2006; Almeida et al. 2006; Wardlow et al. 2007, 2008; Funk and Budde 2009; Becker-Reshef et al. 2010). However, satellite data at a high temporal resolution are often unavailable because of the satellite revisit time, the presence of cloud cover or the high costs of images. Hence, NDVI time series can lose their temporal resolution, sometimes making their use difficult for practical purposes in agriculture (Begue et al. 2010; Ferrara et al. 2010). This limitation can be overcome by the ground-based vegetation indices estimated from a portable and low-cost spectrometer (Gutman 1999; Huemmerich et al. 1999; Wang et al. 2004). Good results have been reported using hand-held radiometers or high resolution ground spectro-radiometers for establishing the irrigation scheduling of corn (Bausch and Neale 1987; Spiliotopoulos and Loukas 2019), potato (Jayanthi et al. 2007), Onion (Bhagyawant 2014), wheat (Kadam et al. 2017), grass hay/pasture (Gautam 2018) cotton and sugar beets (Spiliotopoulos and Loukas 2019).
Sugarcane is a perennial crop which requires a large volume of water to grow so its yield potential is tightly coupled with proper irrigation water management. Predictions of sugarcane water requirement using ground canopy reflectance are rarely discussed in the literature perhaps due to the difficulty in collecting data for a large crop and the year-long growing period (Simoes et al. 2005).The overall objective of this study was to estimate NDVI values from ground-based spectral measurements for sugarcane crop over its entire growth period (1 year) for two different seasons (2015 and 2016) and to investigate its relationships with crop coefficient (Kc) in semi-arid climatic conditions in India. Crop coefficient (Kc) values for sugarcane were determined by the field water balance method.
Materials and methods
The study area
The study area is located in the western part of Maharashtra, India (latitude 19°48′N; longitude 19°57′E; altitude 657 m). Agro-climatically, the region is in the semi-arid and sub-tropical zone with average annual rainfall of 555 mm. About 80% of the total annual rainfall falls during the South-West monsoon (June to September), while the rest is received during the North-East monsoon (October–November). The spatial and temporal distribution of rain is erratic, unevenly distributed over 15 to 45 rainy days a year. The annual mean values based on the 50-year average of important weather parameters are given in Table 1.
The amounts of rainfall received between two successive observations of moisture content in both seasons are given in Table 2. The total rainfall received during the crop growth periods of 2015 and 2016 were 313.2 mm in 38 rainy days and 534.3 mm in 40 rainy days, respectively. The rainfall was 43.6% lower during 2015 and less than average annual rainfall in 2016.
Soil characteristics
A trench was dug at the experimental site to a depth of 1.8 m for extracting soil samples at 0.15 m intervals that were used to determine the textural class, bulk density, field capacity and the wilting point. The soil physical and hydraulic properties for 0.15 m layers are given in Table 3. The textural class of the soil at the experimental site was clay at all depths down to 1.8 m. The soil was medium alkaline having a pH of 8.36 determined in a 1:2.5 (soil:water) solution by the potentiometer method (Jackson 1973). The electrical conductivity of the soil was 0.40 dS m−1.
The average field capacity and permanent wilting point were 41.4 and 17%, respectively, with a bulk density of 1.27 Mg m−3.
Experimental details
Sugarcane seedlings were raised in a nursery and transplanted 35 days after planting in a 6 × 27 m field. The sugarcane seedlings were transplanted at a spacing of 1.5 m × 0.6 m. In the nursery, water was applied every 2 days equal to crop evapotranspiration (ETc) measured by the climatological method (Allen et al. 1998). This irrigation scheduling was continued up to 15 days after transplanting until the seedling was established. The value of the crop coefficient for irrigation was 0.4 (Allen et al. 1998). Other agronomic practices in relation to weeding, off barring, earthing up, fertigation schedule and operations to prevent lodging were followed as recommended by the Mahatma Phule Krishi Vidyapeeth (An Agricultural University), Rahuri, India (Krishidarshini 2014; Pawar et al. 2014).
Irrigation management
At fifteen days, the seedlings were transplanted into the field, the irrigation schedule was started using the field water balance method based on available soil moisture content as measured by the gravimetric method (Reynolds 1970). Soil moisture measurements were made for each 0.15 m layer in the soil profile to the effective rooting depth. The effective root zone was determined by carefully digging and then uprooting one healthy plant for every observation day. The depth of water applied for irrigation was calculated according to the formula (Michael 2010).
where FCi is field capacity in % for ith layer; MCi is the moisture content at the time of irrigation in %; BDi is the bulk density of the soil, for ith layer in g cc−1; Di is effective root zone depth for the ith layer in cm and n is the number of soil layers sampled in the root zone depth.
Irrigation was scheduled every 8–10 days by drip irrigation. The drip irrigation system was only installed to output irrigation and fertigation applications in an exact manner. The drip system was configured with the single row planting of sugarcane where distance between two laterals and emitters was 1.5 m and 0.4 m, respectively.
Estimation of crop evapotranspiration
The simplified soil moisture balance method was used to estimate crop evapotranspiration (ETc) in the root zone between consecutive soil moisture measurement days. The moisture balance, expressed in terms of depletion at the end of consecutive soil moisture measurement days, was calculated by the following equation (Allen et al. 1998);
where ETc is crop evapotranspiration, I is depth of irrigation applied, \({R}_{e}\) is effective portion of rainfall, RO is runoff from the soil surface, DP is deep percolation below the root zone, CR is capillary rise (in the case of a shallow water table), ΔSF is horizontal subsurface flow in or out of the root zone and ΔS is the change in soil profile water storage, respectively. All parameters were expressed in mm.
The effective rainfall was computed using the method mentioned in FAO Document No. 25 (Dastane 1974). Surface runoff, deep percolation and capillary rise were considered zero under flat topography with a deep water table (3 m). Capillary rise was assumed to be zero because the water table was more than 1 m beneath the root zone. To prevent entry of subsurface flow, a 1 m deep interceptor drain was dug around the entire periphery of the experimental plot.
Reference evapotranspiration
Daily air temperature, wind speed, solar radiation and relative humidity for estimating reference evapotranspiration (ETo) as well as rainfall data were measured using an automatic weather station, situated in the experimental field from 12 December 2014 to 23 December 2015 and 24 December 2015 to 31 December 2016 to get information for the 2015 and 2016 growing seasons. The reference evapotranspiration (ETo) was obtained using the Penman–Monteith approach (Allen et al. 1998).
where ETo is reference evapotranspiration (mm day−1); Rn is net radiation at the crop surface (MJ m−2 day−1); G is soil heat flux density (MJ m−2 day−1); T is mean daily air temperature at 2 m height (°C); u2 is wind speed at 2 m height (m s− 1); es is saturation vapour pressure (kPa); ea is actual vapour pressure (kPa); Δ is slope of vapour pressure curve (kPa °C−1) and γ is psychrometric constant (kPa °C−1).
Crop coefficients of sugarcane
The crop coefficients were computed for different growth stages of sugarcane. The growth period was divided into four stages: establishment (0–45 days after planting), tillering (60–135 DAP), grand growth (135–300 DAP) and maturity (300 to 360 DAP). These four growth stages correspond to those defined in FAO-56 (Allen et al. 1998) respectively as initial, development, mid-season and end of season. In this investigation, the later three stages were considered. The initial stage was skipped with considering that all the farmers in this region essentially raise seedlings in a nursery to attain good germination at the initial stage.
Crop evapotranspiration values were obtained by monitoring the soil moisture balance in the crop root zone and ETo values estimated using climatic data for specified periods. The crop coefficients (Kc) were computed as the ratio of crop evapotranspiration and reference evapotranspiration (ETc/ETo) for the respective period (7–10 days interval). The polynomial equation was fitted with Kct as the dependent variable and (t/T) for the three development stages as the independent variables.
where, Kct is the crop coefficient of tth day; a0, a1, a2 are regression coefficients; t is day considered; T is total period of crop growth from planting to harvesting (days). The regression coefficients were estimated and tested for their significance to decide on the validity of the particular equation. Using the best fit equation, the daily Kc values were estimated for both seasonal and average daily Kc values [starting from 50 days after planting (DAP)] were determined and represented in the form of polynomial equations.
Spectral measurements
In both seasons, sugarcane canopy spectral reflectance was recorded using a ground spectro-radiometer (HR-1024, multispectral radiometer manufactured by Spectra Vista Corporation, New York, USA) at 10 day intervals (except on rainy days). The HR-1024 spectro-radiometer is capable of measuring the spectra of different light reflected from the target between 350 and 2500 nm. In both seasons (2015 and 2016), spectral signatures were categorized during the entire growth period namely, the tillering (45–135 DAP), grand growth (135–300 DAP) and maturity stages (300–360 DAP). Initially five sugarcane plants from each replication were selected randomly, labelled with pegs and red cloth and subsequently used for recording spectral observations. Spectral signatures were obtained from these five locations for the entire crop season. Spectral reflectance observations commenced 50 days after planting. Early observations were taken directly by holding the spectro-radiometer over the canopy. However, later in the season (> 120 DAP) when the canopy was high, a portable folding ladder was used. Ten readings were taken for each observation day.
Data analysis
The data recorded at the time of field measurement were stored in the form of an ASCII file in a personal digital assistant (PDA) provided with the instrument. The data were then transferred to a computer for further processing in a 32-bit data processing software with Graphic Interface Application developed by manufacturer Spectra Vista Corporation, New York, USA. The overlay matching of data was performed and the data were subsequently exported to an Excel spreadsheet and average spectral signatures were calculated. This procedure was performed for each observation day. The NDVI was calculated using the standard formula of Rouse et al. (1974). The near infrared (NIR) band ranges from 700 to 1300 nm and red (IR) band ranges from 620 to 699 nm.
NDVI values were calculated by averaging the observed reflectance values. The observed NDVI values were further used to develop temporal NDVI functions over the growth period to estimate daily NDVI values. Polynomial equations of different orders were fitted as a function for the relationship between NDVI and ratio of day since planting (t) to total crop growth period (T). The best fit equation was selected on the basis of the coefficient of determination (R2), root mean square error (RMSE), Chi square and mean absolute relative error (MARE). The average daily NDVI values were then estimated by taking the average of daily estimated NDVI values for 2015 and 2016. The NDVI values were related to crop coefficient values by examining the relationship between Kc and NDVI.
Results and discussion
Crop evapotranspiration
On an annual basis, the total reference evapotranspiration (ETo) ranged from 1318 to 1426 mm. The total irrigation water applied, effective rainfall and sugarcane evapotranspiration observed for 2015 were 1173, 313 and 1387 mm, respectively whereas the corresponding values for 2016 were 808, 534 and 1291 mm, respectively. The 2-year average crop evapotranspiration for sugarcane was 1339 mm including 991 mm of irrigation water and 424 mm effective rainfall. The moisture remaining in the soil as soil moisture storage was 76 mm. Sugarcane evapotranspiration (ETc) varies considerably from place to place depending on weather conditions, texture of soil and age of the crop. Depending on the approach used, sugarcane evapotranspiration is reported to range between 1000 and 1800 mm for different locations in the world (Carr and Knox 2011). Therefore, the sugarcane evapotranspiration of 1339 mm derived by the field water balance approach in this study seems to be appropriate for semi-arid conditions.
Crop coefficient of sugarcane
The temporal pattern of crop coefficient values derived from field soil water balance curves showed good agreement with the Kc-FAO in both growing seasons. However, some variations were observed among growth stages in both seasons (Figs. 1 and 2). In both seasons, the Kc ranged between 0.34 and 1.33. In the first year, Kc consistently increased from 0.34 to 0.98 (54–128 DAP) but in the second season it increased to 1.13. This is due to the rapid crop development in the form of tillering which caused an increase in ETc and hence resulted.
in an increase in the Kc value. Win et al. (2014) reported the Kc value for the sugarcane during the development stage as 0.81 estimated from lysimeters in Myanmar. Kc showed rapid increase due to crop development (cane elongation) in the grand growth stage (mid-season stage). During 2015 (129–299 DAP), Kc increased and remained in the range of 1.19–1.30 with a peak value of 1.33 (189 DAP). In 2016, the range was 1.24–1.15 with a peak value of 1.32 at 217 DAP. Inman-Bamber and McGlinchey (2003) found a Kc value of 1.25 for sugar cane using the Bowen ratio energy balance (BREB) method in Australia and Swaziland; while, Silva et al. (2012) estimated it to be 1.43 in Brazil.
The Kc of the 2015 decreased gradually from 1.10 to 0.58 during the maturity stage i.e. end of season (300–360 DAP). The corresponding range obtained for 2016 was 1.15 to 0.63 (305–360 DAP) but the rate of decrease was lower than in the first season. For the decline phase of the current study, the Kc curve showed an early decrease with a lower Kc value (0.78) than the FAO-56 value (0.98). The average crop coefficient values for tillering, grand growth and maturity stages in 2015 were 0.67; 1.20 and 0.73, respectively and for 2016 they were 0.75; 1.23 and 0.80, respectively. In 2016, the estimated Kc was slightly higher than that of 2015 at almost all the stages. The lesser total ETo (1318 mm) might have elevated Kc values in 2016 as a ratio of ETc/ETo.
The 2 year average Kc value for the tillering stage was 0.70, which is lower than the FAO-56 value of 0.94. The average Kc value for the grand growth (mid season) stage was slightly lower, 1.20 than the FAO-56 value of 1.25. The percentage difference between the FAO Kc and the values developed in this study was 25.5%, 4% and 20.4% for the tillering, grand growth and maturity stages, respectively. The overall average percent difference between the values developed in this study and those of the FAO-56 was 16.6%. The lower Kc obtained in this investigation may be due to the semi-arid climatic conditions in the study area.
The crop coefficient (Kc) can be estimated by 2nd order polynomial functions (Table 4) expressed as the ratio of days after transplanting to the total crop period \(\left(\frac{t}{T}\right)\).
Spectral reflectance of the sugarcane crop
The trend of reflectance was similar in both years but slightly higher reflectance was observed in 2016. The trend of spectral reflectance for both of the seasons indicated an increase in reflectance in the visible region and a decrease in the NIR. In the visible region, maximum reflectance of the blue, green and red bands was obtained at 450 nm, 550 nm and 650 nm, respectively. However, beyond the visible range (> 700 nm), there was a strong rise in the reflectance in the NIR region (750 to 1300 nm). The reflectance was lower at 1400 nm because of strong absorption by water in leaves. Overall, high reflectance was observed in the visible region in 2015 while, in 2016, it was higher in the NIR due to favourable climatic conditions in respective growth stages (Fig. 3).
Spectral reflectance in different growth stages of sugarcane
The spectral response at different crop stages showed higher reflectance in the visible region (< 700 nm) at the tillering stage compared to both the grand growth and maturity stages (Figs. 4 and 5). In the near infrared region (700–1300 nm), reflectance was lowest in the tillering stage, but was the highest in both the grand and maturity growth stages, especially in 2015.
Unlike other crops, the production of fresh green leaves at the top of the cane continues in sugarcane, and thus the reflectance in the near infrared region in maturity stage did not decrease. Overall, the shapes of the reflectance curves for both years showed higher reflectance in the green band (550 nm) and NIR (700 to 1300 nm).
NDVI variation over growth period
The NDVI temporal profiles for both 2015 and 2016 showed a similar pattern that corresponded to the crop cycle. The graphical variation of observed NDVI values is shown in Fig. 6. During the tillering stage, the NDVI values increased from 0.48 to 0.63 in 2015 and 0.46 to 0.75 in 2016. In the grand growth stage in 2015, NDVI increased from 0.73 to 0.95 and thereafter decreased to 0.91 during the maturity stage. In the grand growth stage in 2016, NDVI increased from 0.79 to 0.95 and remained in the range of 0.91–0.95 due to the effect of late monsoon rains. This greater NDVI value was observed due to a greater amount of healthy green vegetation in the grand growth stage. This relationship is deduced from the physiological fact that chlorophyll a and b in the palisade layer of healthy green leaves absorbs most of the incident red radiant flux while the spongy mesophyll leaf layer reflects much of the near infrared radiant flux (Ferrara et al. 2010).
In both years, contrary to annual crops, the NDVI of sugarcane at the maturity stage did not drop below 0.86 (Fig. 6). This was mainly due to the physiological characteristics of sugarcane crop which continuously produced fresh green leaves at the top irrespective of the crop growth stage. Similar results were also reported by Nagy et al. (2013) for apple.
Regression equations for sugarcane NDVI
The NDVI profile for the 2 years exhibited peaks and valleys as a result of the sequence and amount of water applied (Fig. 6). To deal with these peaks and valleys, higher order polynomial curve fitting could have been done but they resulted in large variation in observed and estimated NDVI values. Therefore, only 2nd and 3rd order curve fitting was considered as it gave a comprehensive trend of NDVI variation over the growth period (Table 5). The equations are valid from 50 DAP i.e. 10 days after transplanting to 360 DAP.
Combining Kc and NDVI variation for sugarcane
The Kc and NDVI values varied significantly over both seasons (Figs. 7 and 8). The average Kc and NDVI values increased from the tillering stage and to the grand growth stage (Fig. 9). During the tillering stage, both Kc and NDVI values ranged between 0.48 and 0.69. However, during grand growth stage (mid-season stage), Kc ranged between 1 and 1.2 whereas NDVI ranged between 0.69 and 0.93. The Kc decreased sharply during the maturity stage due to decreasing crop evapotranspiration (ETc) whereas NDVI values remained constant around 0.90–0.95. This revealed the typical physiological characteristics of the sugarcane crop of producing new fresh green leaves at the top of the cane throughout the season and hence, poor association of the Kc and NDVI observed at the maturity stage.
Kc–NDVI relationship for sugarcane
Curve fitting of linear, exponential, logarithmic, power and polynomial Kc–NDVI equations for 2015, 2016 and the average of both years are given in Tables 6, 7 and 8. In both years and for the average, a 2nd order polynomial was found to be the best fit on the basis of a low Chi square value and mean absolute relative error (MARE).
The Kc–NDVI equations developed for sugarcane in this study are useful for estimating real time Kc values for real time irrigation water management in sugarcane. The NDVI can be obtained from ground spectro-radiometer measurements or by using a handheld NDVI meter.
Kc–NDVI relationship for the growth and decline phase
The correlation between Kc and NDVI was moderately strong for the year 2015 (r = 0.76, n = 315) and 2016 (r = 0.74, n = 315). A number of researchers have reported r values ranging from 0.80 to 0.95 when studying the relationship between vegetation indices and Kc values for various crops such as corn (Neale et al. 1989; Bausch 1995), wheat (Bandyopadhyay and Mallick 2003; Gontia and Tiwari 2010); cotton (Hunsaker et al. 2003), potato (Jayanthi et al. 2007), soybean (Singh and Irmak 2009). However, no results are available for sugarcane as a crop. Sugarcane plants continue to produce fresh green leaves at the top of the plant and thus the NDVI does not decrease at maturity. This probably explains a slight low correlation (r value) between NDVI and Kc in the current study.
In addition, the Kc-NDVI relationships mostly showed two NDVI values for a Kc value (Fig. 10) which is not correct in principal. Therefore, the growth period was divided into two segments, one for increasing values (growth phase) and the other for decreasing values (declining phase) and separate relationships were obtained for both the segments. For this purpose, the peak or maximum values of estimated NDVI were identified as the junction points for the growth and decline phases. Based on the peak value of estimated NDVI, the growth period identified for the growth phase was 50–294 DAP, while the remaining period 295–365 DAP was considered the decline phase.
Second order polynomial equations were found to be the best fit for both the growth and decline phase of Kc–NDVI relationship for sugarcane (Table 9). The coefficient of correlation r was near to 1 for both incline and decline phase. The combination of the growth and decline phase for Kc–NDVI relationships can provide very accurate information for real time irrigation water management for the farmers if coupled with an information technology-based communication system (Figs. 11 and 12).
Conclusions
This study provided synoptic, online and real time information on the crop characteristics on which irrigations can be based and automated, made more precise and made more sensitive to climate in different parts of the world. This investigation developed a Kc estimation model using NDVI data for sugarcane. The Kc data were determined from field water balance and NDVI was derived from canopy spectral reflectance of sugarcane during two experiments. The estimated Kc values for the tillering, grand growth and maturity stages for sugarcane were 0.70, 1.20 and 0.78, respectively. The results showed that the FAO-Kc may lead to an over-estimation in irrigation scheduling of sugarcane in semi-arid conditions.
The spectral responses showed the interesting features of sugarcane. In contrast to other crops, the spectral reflectance in the NIR region did not decrease substantially in the maturity stage. The NDVI increased with advancement of crop age and attained its peak during the mid-season stage. The NDVI in the maturity stage did not drop below 0.85 and this showed the typical physiological character of the sugarcane crop.
In contrast to other crops, unique Kc–NDVI relationships were obtained for sugarcane by splitting the growth period into the growth phase (r = 0.98, n = 245) and decline phase (r = 0.99, n = 70). The Kc–NDVI equations are useful to inform precise real time irrigation water management for sugarcane under similar agro-climatic conditions. For NDVI measurement, it is advisable to use a handheld NDVI meter owing to its easier use and coverage for sugarcane vegetation. Future research can involve determining relationships between ground NDVI and satellite derived NDVI. The satellite based NDVI relationship can further be used in classification and mapping of the sugarcane crop over a large area for efficient water resource management.
References
Allen, R. G., Pereira, L. S., Raes, D., & Smith, M. (1998). Crop evapotranspiration. FAO Irrigation and Drainage Paper No. 56, Rome, Italy.
Almeida, T. I. R., De Souza, C. R., & Rossetto, R. (2006). ASTER and Landsat ETM + images applied to sugarcane yield forecast. International Journal of Remote Sensing, 27, 4057–4069.
Anderson, R. G., Wang, D., Tirado- Corbalá, R., Zhang, H., & Ayars, J. E. (2015). Divergence of actual and reference evapotranspiration observations for irrigated sugarcane with windy tropical conditions. Hydrology and Earth System Science, 19, 583–599. https://doi.org/10.5194/hess-19-583-2015.
Bandyopadhyay, P. K., & Mallick, S. (2003). Actual evapotranspiration and crop coefficients of wheat (Triticum aestivum) under varying moisture levels of humid tropical canal command area. Agricultural Water Management, 59, 33–47. https://doi.org/10.1016/S0378-3774(02)00112-9.
Bastiaanssen, W. G. M., Molden, D. J., & Makin, I. W. (2000). Remote sensing for irrigated agriculture: examples from research and possible applications. Agricultural Water Management, 46(2), 137–155. https://doi.org/10.1016/S0378-3774(00)00080-9.
Bausch, W. C. (1995). Remote sensing of crop coefficients for improving the irrigation scheduling of corn. Agricultural Water Management, 27, 55–68. https://doi.org/10.1016/0378-3774(95)01125-3.
Bausch, W. C., & Neale, C. M. U. (1987). Crop coefficients derived from reflected canopy radiation: a concept. Transaction of the ASAE, 30(3), 703–709. https://doi.org/10.13031/2013.30463.
Becker-Reshef, I., Vermonte, E., & Justice, C. (2010). A generalized regression-based model for forecasting winter wheat yields in Kansas and Ukraine using MODIS data. Remote Sensing of Environment, 114, 1312–1323.
Begue, A., Lebourgeois, V., Bappel, E., Todoro, P., & Pellegrino, A. (2010). Spatio-temporal variability of sugarcane fields and recommendations for yield forecast using NDVI. International Journal of Remote Sensing, 31(20), 5391–5407.
Bhagyawant, R. G. (2014). Deficit irrigation for Rabi onion production under semiarid condition. Ph.D. Thesis submitted to Mahatma Phule Krishi Vidyapeeth University, Rahuri, India.
Cardoso, G. G. G., Campos de Oliveira, R., Teixeira, M. B., Dorneles, M. S., Domingos, R. M. O., Megguer, C. A., et al. (2015). Sugarcane crop coefficient by the soil water balance method. African Journal of Agricultural Research, 10(24), 2407–2414. https://doi.org/10.5897/AJAR2015.9805.
Carr, M. K. V., & Knox, J. W. (2011). The water relations and irrigation requirements of sugar cane (Saccharum officinarum): A review. Experimental Agriculture, 47(1), 1–25. https://doi.org/10.1017/S0014479710000645.
Chabot, R., Bouarfa, S., Zimmer, D., Chaumont, C., & Moreau, S. (2005). Evaluation of the sap flow determined with a heat balance method to measure the transpiration of a sugarcane canopy. Agricultural Water Management, 75, 10–24. https://doi.org/10.1016/j.agwat.2004.12.010.
Choudhury, B. J., Ahmed, N. U., Idso, S. B., Reginato, R. J., & Daughtry, C. S. T. (1994). Relations between evaporation coefficients and vegetation indices studied by model simulations. Remote Sensing of Environment, 50, 1–17. https://doi.org/10.1016/0034-4257(94)90090-6.
Commission for Cost and Prices (CACP). (2019). Price policy for sugarcane, 2019–2020 Sugar season (pp. 26–27). New Delhi: Department of Agriculture and Cooperation, Ministry of Agriculture, Government of India.
Dastane, N. G. (1974). Effective rainfall in irrigated agriculture. FAO Irrigation and Drainage Paper No. 25. Rome, Italy.
Doraiswamy, P. C., Hatfield, J. L., Jackson, T. J., Akhmedov, B., Prueger, J., Stern, A., et al. (2004). Crop conditions and yield simulations using Landsat and MODIS. Remote Sensing of Environment, 92, 548–559.
Ferrara, R. M., Fiorentino, C., Martinelli, N., Garofalo, P., & Rana, G. (2010). Comparison of different ground-based NDVI measurement methodologies to evaluate crop biophysical properties. Italian Journal of Agronomy, 5, 145–154.
Funk, C., & Budde, M. E. (2009). Phenologically-tuned MODIS NDVI-based production anomaly estimates for Zimbabwe. Remote Sensing of Environment, 113, 115–125.
Gautam, S. (2018). Multispectral remote sensing to estimate actual crop coefficients and evapotranspiration rates for grass pastures in western Colorado. PhD. thesis submitted to Colorado State University, Fort Collins, CO, USA.
Gontia, N. K., & Tiwari, K. N. (2010). Estimation of crop coefficient and evapotranspiration of wheat (Triticum aestivum) in an irrigation command using remote sensing and GIS. Water Resources Management, 24, 1399–1414. https://doi.org/10.1007/s11269-009-9505-3.
Gutman, G. G. (1999). On the use of long-term global data of land reflectances and vegetation indices derived from the advanced very high resolution radiometer. Journal of Geophysical Research, 104, 6241–6255. https://doi.org/10.1029/1998JD200106.
Heilman, J. L., Heilman, W. E., & Moore, D. G. (1982). Evaluating the crop coefficient using spectral reflectance. Agronomy Journal, 74, 967–971. https://doi.org/10.2134/agronj1982.00021962007400060010x.
Hill, M. J., & Donald, G. E. (2003). Estimating spatio-temporal patterns of agricultural productivity in fragmented landscapes using AVHRR NDVI time series. Remote Sensing of Environment, 84, 367–384.
Huemmerich, K. F., Black, T. A., Jarvis, P. G., McCaughey, J. H., & Halls, F. G. (1999). High temporal resolution NDVI phenology from micrometeorological radiation sensors. Journal of Geophysical Research, 104(D22), 27935–27944. https://doi.org/10.1029/1999JD900164.
Hunsaker, D. J., Pinter, P. J. Jr., Barnes, E. M., & Kimball, B. A. (2003). Estimating cotton evapotranspiration crop coefficients with a multispectral vegetation index. Irrigation Science, 22, 95–104. https://doi.org/10.1007/s00271-003-0074-6.
Inman-Bamber, N. G., & McGlinchey, M. G. (2003). Crop coefficients and water-use estimates for sugarcane based on long-term Bowen ratio energy balance measurements. Field Crops Research, 83, 125–138. https://doi.org/10.1016/S0378-4290(03)00069-8.
Jackson, M. L. (1973). Soil chemical analysis. New Delhi, India: Prentice Hall of India Private Limited.
Jackson, R. D., & Huete, A. R. (1992). Interpreting vegetation indices. Preventive Veterinary Medicine, 11, 185–200. https://doi.org/10.1016/S0167-5877(05)80004-2.
Jagtap, S. S., & Jones, J. W. (1989). Stability of crop coefficients under different climatic and irrigation management practices. Irrigation Science, 10, 231–244. https://doi.org/10.1007/BF00257955.
Jayanthi, H., Neale, C. M. U., & Wright, J. L. (2007). Development and validation of canopy reflectance based crop coefficient for potato. Agricultural Water Management, 88(1–3), 235–246. https://doi.org/10.1016/j.agwat.2006.10.020.
Kadam, S., Gorantiwar, S., Das, S., & Joshi, A. (2017). Crop evapotranspiration estimation for wheat (Triticum aestivum L.) using remote sensing data in semi-arid region of Maharashtra. Journal of the Indian Society of Remote Sensing, 45(2), ,297-305. https://doi.org/10.1007/s12524-016-0594-1.
Kamble, B., Irmak, A., & Hubbard, K. (2013). Estimating crop coefficients using remote sensing-based vegetation index. Remote Sensing, 5, 1588–1602. https://doi.org/10.3390/rs5041588.
Kastens, J. H., Kastens, T. L., Kastens, D. L. A., Price, K. P., Martinko, E. A., Lee, R., et al. (2005). Imagemasking for crop yield forecasting using AVHRR NDVI time series imagery. Remote Sensing of Environment, 113, 115–125.
Krishidarshini. (2014). Official Publication of Mahatma Phule Krishi Vidyapeeth (An Agricultural University) (pp. 82–92), Rahuri, Maharashtra, India.
Michael, A. M. (2010). Irrigation: Theory and practice (3rd ed.). New Delhi, India: Vikas Publishing House Private Limited.
Mkhabela, M. S., Mkhabela, M. S., & Mashinini, N. N. (2005). Early maize yield forecasting in the four agro-ecological regions of Swaziland using NDVI data from NOAA’s AVHRR. Agricultural and Forest Meteorology, 129, 1–9.
Moran, M. S., Mass, S. J., & Pinter, Jr. P. J. (1995). Combining remote sensing and modelling for estimating surface evaporation and biomass production. Remote Sensing of Environment, 12, 335–353. https://doi.org/10.1080/02757259509532290.
Nagy, A., Peter, R., Eva, B., Bernadett, G., Peter, A. M., Janos, T., et al. (2013). Complex vegetation survey in a fruit plantation by spectral instruments. Agricultural Informatics, 4(2), 37–42. https://doi.org/10.17700/jai.2013.4.2.112.
Neale, C. M. U., Bausch, W. C., & Heerman, D. F. (1989). Development of reflectance-based crop coefficients for corn. Transactions of the ASAE, 32(6), 1891–1899. https://doi.org/10.13031/2013.31240.
Omary, M., & Izuno, F. T. (1995). Evaluation of sugarcane evapotranspiration from water table data in the Everglades agricultural area. Agricultural Water Management, 27, 309–319. https://doi.org/10.1016/0378-3774(95)01149-D.
Pawar, D. D., Dingre, S. K., & Durgude, A. G. (2014). Enhancing nutrient use and sugarcane (Saccharum officinarum) productivity with reduced cost through drip fertigation in Western Maharashtra. Indian Journal of Agricultural Sciences, 84(7), 844–849.
Raki, S. E., Abdelghani, C., Guemouria, N., Duchemin, B., & Ezzahar, J. (2006). Combining FAO-56 model and ground-based remote sensing to estimate water consumptions of wheat crops in a semi-arid region. Agricultural Water Management, 87, 41–54. doi:https://doi.org/10.1016/j.agwat.2006.02.004.
Reynolds, S. G. (1970). The gravimetric method of soil moisture determination. Part I: A study of equipment, and methodological problems. Journal of Hydrology, 11(3), 258–273. https://doi.org/10.1016/0022-1694(70)90066-1.
Rouse, J. W., Haas, R. H., Schell, J. A., Deering, D. W., & Harlan, J. C. (1974). Monitoring the vernal advancement and retrogradation of natural vegetation. NASA/GSFC, Type III. Final report (1-371), Greenbelt, MD.
Shibayama, M., & Akiyama, T. (1986). A spectroradiometer for field use: VI. Radiometric estimation for chlorophyll index of rice canopy. Japanese Journal of Crop Science, 55, 433–438.
Silva, V., Borges, C., Farias, C., Singh, V., Albuquerque, W., Silva, B., et al. (2012). Water requirements and single and dual crop coefficients of sugarcane grown in a tropical region, Brazil. Agricultural Sciences, 3, 274–286. https://doi.org/10.4236/as.2012.32032.
Simoes, M. D. S., Rocha, J. V., & Lamparelli, R. A. C. (2005). Spectral variables, growth analysis and yield of sugarcane. Scientia Agricola, 62, 199–207.
Singh, R. K., & Irmak, A. (2009). Estimation of crop coefficients using satellite remote sensing. Journal of Irrigation and Drainage Engineering, 135(5), 597–608. https://doi.org/10.1061/(ASCE)IR.1943-4774.0000052.
Spiliotopoulos, M., & Loukas, A. (2019). Hybrid methodology for the estimation of crop coefficients based on satellite imagery and ground based measurements. Water, 11(7), 1364. https://doi.org/10.3390/w11071364.
Thompson, G. D., & Boyce, J. P. (1971). Comparisons of measured evapotranspiration of sugarcane from large and small lysimeters: In Proceedings of the South African Sugar Technologists Association, 45:169–176.
Wang, Q., Tenhunen, J., Quoc Dinh, N., Richstein, M., Vesala, T., Keronen, P., et al. (2004). Similarities in ground- and satellite-based NDVI time series and their relationship to physiological activity of a Scots pine forest in Finland. Remote Sensing of Environment, 93, 225–237. https://doi.org/10.1016/j.rse.2004.07.006.
Wardlow, B. D., Egbert, S. L., & Kastens, J. H. (2007). Analysis of time-series MODIS 250 m vegetation index data for crop classification in the U.S. Central Great Plains. Remote Sensing of Environment, 108, 290–310.
Wardlow, B. D., Egbert, S. L., & Kastens, J. H. (2008). Large area crop mapping using timeseries MODIS 250 m NDVI data: An assessment of the U.S. Central Great Plains. Remote Sensing of Environment, 112, 1096–1116.
Win, S. K., Zamora, O. B., & Thein, S. (2014). Determination of the water requirement and kc values of sugarcane at different crop growth stages by lysimetric method. Sugar Tech, 16(3), ,286-294. https://doi.org/10.1007/s12355-013-0282-1.
Wooten, J. R., Akins, D. C., Thomasson, J. A., Shearer, S. A., & Pennington, D. A. (1999). Satellite imagery for crop stress and yield prediction: Cotton in Mississippi. Paper 991133. St. Joseph, MI: ASAE.
Author information
Authors and Affiliations
Corresponding author
Additional information
Publisher’s Note
Springer Nature remains neutral with regard to jurisdictional claims in published maps and institutional affiliations.
Rights and permissions
About this article
Cite this article
Dingre, S.K., Gorantiwar, S.D. & Kadam, S.A. Correlating the field water balance derived crop coefficient (Kc) and canopy reflectance-based NDVI for irrigated sugarcane. Precision Agric 22, 1134–1153 (2021). https://doi.org/10.1007/s11119-020-09774-8
Accepted:
Published:
Issue Date:
DOI: https://doi.org/10.1007/s11119-020-09774-8