ABSTRACT
Purpose
Chitosan (CH) coated ciprofloxacin-sodium deoxycholate surfplex (CFn-SDC) loaded nanoemulsion (LE-CH-CFn-SDC) developed in order to improve tissue penetration of the CFn as well as to mop up the endotoxin (Lipopolysaccharides or LPS) released from bacteria during antibiotic treatment.
Methods
Size and zeta potential was evaluated for nanoemulsions prepared by high-speed homogenization and sonication. Drug analysis in samples was done by HPLC equipped with fluorescence detector. All formulations were evaluated for any change in LPS induced NO and TNF-α release and ROS generation in J774 macrophages. The formulations were also evaluated for in-vitro killing efficiency on E-Coli. The efficacy of formulations in terms of survival and pharmacokinetics and inhibition of induction of cytokines was carried out in E-coli induced peritonitis model in rats. LE-CH-CFn-SDC interacted with LPS both by electrostatic and hydrophobic interactions.
Results
LE-CH-CFn-SDC resulted in reduction of endotoxin release and MIC values for E. coli. LE-CH-CFn-SDC also reduced NO and TNF-α as well as ROS generation by reducing the uptake of LPS in J774 macrophages. LE-CH-CFn-SDC improved CFn pharmacokinetics and tissue distribution, by reducing the bacterial burden, LPS and cytokines (TNF-α and IL-6) thereby improving survival in a rat model of E. coli induced peritonitis.
Conclusion
In conclusion, this work highlights the effectiveness of the chitosan-coated nanoemulsion as intracorporeal approach for therapeutic intervention of E. coli induced peritonitis as well as in sepsis.
Similar content being viewed by others
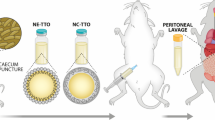
Avoid common mistakes on your manuscript.
INTRODUCTION
Sepsis is a spectrum of clinical conditions caused by the immune response of a patient to infection that is characterized by systemic inflammation and coagulation (1). Complicated intra-abdominal infections (cIAI) often lead to sepsis and septic shock (2) which results in high health care costs (3). The etiology of cIAI is often polymicrobial (4), the microbiological results that are difficult to interpret. Sepsis the full range of response from systemic inflammatory response (de-regulation of cytokines, like interleukin-1β (IL-1β), IL-6 and tumor necrosis factor alpha (TNF-α) in response to Lipopolysaccharide) to organ dysfunction to multiple organ failure and ultimately death (5).
Therapeutic intervention involves use of surgical methods and broad-spectrum antimicrobial therapy for optimal outcome. In recent past, extracorporeal dialysis based anti-endotoxin therapies are being mainly in use for reduction of circulating levels of LPS (5–7). The major limitation of dialysis methods is that they are very painful and expensive.
Ciprofloxacin (CFn) is a fluoroquinolone frequently employed in therapeutic management of gram −ve peritonitis in clinical settings (8). Besides immunomodulatory effect, the frequency of spontaneous resistance to ciprofloxacin (CFn) is very low. Previously, our group (9) has reported preparation of surf-plexes of CFn with sodium deoxycholate (SDC) i.e. CFn-SDC, which improved entrapment efficiency of CFn upon loading within lipid nanoemulsion and also improved the antimicrobial efficacy when cationicity was imparted on the surface using chitosan (CH). As sustained release has been proved to be successful strategy to improve drug efficacy and/or reduce drug toxicity due to alteration of its tissue distribution and pharmacokinetics (10). Phospholipid emulsion has been known to attenuate clinical and laboratory effects associated with the administration of LPS. Attenuation of TNF-α and IL-6 levels were related to serum phospholipid levels in plasma thereby suggesting a novel approach to the treatment of endotoxemia (11).
Compounds that bind to components of the gram-negative outer membrane [i.e. Lipopolysaccharide (LPS)] and ablate their ability to activate surface receptors would be good candidates as an anti-endotoxic agent. However, to develop a molecule that could bind specifically to LPS is tedious job. Few polycationic substances are known to bind with lipid A portion of LPS by simple hydrophobic and electrostatic interaction but are reported to be severely toxic and cannot be administered repeatedly.
Nanoengineered system with cationic polymer chitosan are commonly used in drug delivery (10). Chitosan is having anti-microbial, anti-oxidative, anti-tumor and immune-promoting activities. Chitosan can directly interact with LPS and forms a stable LPS-chitosan complex. The induction of TNF-α, IL-6 biosynthesis by LPS–chitosan complex was lower than that of parent LPS (9,12).
Therefore, the strategy focuses on the development of target-oriented delivery systems with certain degree of cationicity and hydrophobicity that could interact with LPS (either hydrophobic or electrostatic) to alleviate macrophage stimulation and circumvent further toxic cascading events. This strategy would be advantageous for therapeutic intervention of sepsis after antibiotic treatment of E. coli induced peritonitis. Here, we propose to develop cationic lipid nanoemulsion incorporating ciprofloxacin (CFn) for parenteral delivery with an attempt to reduce bacterial burden, removal of LPS and attenuation of inflammatory responses in gram −ve E. coli induced peritonitis.
Materials
Soya-oil, lecithin, poloxamer 188 (Pluronic F68; PF-68), chitosan, Sodium deoxycholate (SDC), MTT, Dulbecco’s modified eagle medium (DMEM) with glutamate, Griess reagent, fetal bovine serum (FBS), antibiotic solution (penicillin/streptomycin, 0.1%, v/v) and Trypan blue solution were purchased from Sigma Aldrich (MO, USA). All other ingredients were of pharmaceutical grade. Alkem Laboratories (Mumbai, India) generously provided Ciprofloxacin (CFn) as a gift sample.
Preparation and Optimization of Lipid Nanoemulsions Comprising CFn and CFn-SDC Complex
Different lipid nanoemulsions (Table I) loaded with CFn (LE-CFn) and CFn-SDC complex (LE-CFn-SDC) were prepared and optimized by the method reported described previously by our group (9). Briefly, CFn or CFn-SDC (equivalent to 0.3% CFn) was incorporated in the lipid phase (soya oil and lecithin) after dissolving in chloroform, which was then removed by evaporation. This lipid phase added gradually to aqueous phase containing PF-68 at 70°C accompanied with stirring. The primary emulsion was prepared by stirring for 20 min using a high shear mixer (Ultra Turrax T25, Janke & Kunkel, Germany) at the speed of 22,000 rpm, and subsequently sonicated using an ultrasonic probe at 20% amplitude for 5 min. Similarly, Chitosan (CH) based i.e. blank cationic lipid nanoemulsion (LE-CH) and loaded with CFn-SDC (LE-CH-CFn-SDC) was developed. Cationic lipid nanoemulsion was prepared by the method described above by using isotonic aqueous CH solution in 5% sorbitol (pH 6.0).
Physicochemical Characterization
Globule Size and Zeta Potential
The globule size and zeta potential were measured using the Malvern Zetasizer (Malvern, UK) while its pH was recorded at given time intervals using a pH meter (Corning pH meter 245, USA). The viscosity of the sample was determined using cone and plate Viscometer (Bohlin, USA). Measurements were been done at room temperature after appropriated dilution of emulsion samples.
Entrapment Efficiency
The CFn loaded emulsions were centrifuged at 48,000×g and 4°C for 30 min in a Beckman Optima MAX® ultracentrifuge (Beckman Coulter, USA) in order to separate the incorporated drug from the non-incorporated drug. The supernatants were analyzed by RP-HPLC for the un-incorporated drug (A1) concentration to determine the encapsulation percentage from total amount of drug (A2). Entrapment Efficiency (EE) was calculated using the equation: EE (%) = (A2 − A1/A2) × 100.
Drug Analysis
CFn concentrations in the samples were measured by reported RP-HPLC method with slight modifications (9).
Stability Assessment
The drug pH and droplet size distribution were monitored over a period of time stored at 4°C and 37°C. The creaming and the phase separation were assessed visually for any changes.
Interaction Studies Between LPS and Cationic Lipid Nanoemulsion
Zeta-potential distribution assay and quasi-elastic light scattering was employed to study interaction between LPS (lipopolysaccharide from E. coli 055 B5) and cationic lipid nanoemulsion. The sizes and zeta-potentials of the LPS aggregates and LPS–Cationic lipid nanoemulsion complexes in solution were determined by means of a Zetasizer Nano ZS (Malvern, UK) operating at a wavelength 633 nm. Prior to measurement the samples were left for 1 h at 25°C (13) Briefly LPS (1 mg/ml) and nanoemulsions (5 mg/ml) were incubated in quantities equivalent to 1:0.5 w/w and 1:1 ratio of LPS and chitosan (i.e. 100 and 200 μl of nanoemulsion respectively with 1 ml of LPS).
Dissolution Kinetics of CFn-SDC Complex
A dissociation study in PBS (pH 7.4) was performed by a modified protocol described previously (14). Briefly, ion pair complex (CFn-SDC) was dissolved in chloroform and poured into a glass vial chloroform was evaporated by using nitrogen stream to form a continuous film on bottom of vial. Ten milliliter of PBS and distilled water was added to the vial kept on shaking water bath at 37°C. The samples were taken at different time intervals At designated time points, 100 μl of aliquots were removed, filtered using a 0.2 mm pore size syringe filter and assayed by HPLC method described (9).
In Vitro Studies on E. coli and J774 Macrophages Tissue Culture
In-Vitro Killing Efficiency and Free LPS Release
Two milliliter of Bacterial culture (5 × 105 CFU/ml) in cation-supplemented Mueller-Hinton (MH) broth was treated with 2, 5 10 times of MIC of the respective formulations (LE-CFn, LE-CFn-SDC, LE-CH-CFn-SDC) and activity was compared with respective blank formulation (LE-CH) and plain CFn solution. After 6 h of incubation, viable bacterial cells were determined by sub-culturing on MH agar plates. For LPS estimation the supernatant of the bacterial suspension was separated by centrifugation and frozen at −80°C until determination of free LPS by limulus amebocyte lysate (LAL) test (15).
Effect of CFn Lipid Nanoemulsion on Release of Pro-Inflammatory Mediator and Cellular Binding
LPS Induced NO and TNF-α Release
J 774 Φ (1.5 × 106 cells/well) in DMEM media containing 10% Fetal bovine serum were seeded in a 48 well plates and after 24 h (at 37°C) cells were incubated with LPS (1 μg/ml) alone and LPS (1 μg/ml) along with formulations at different dose levels for 18 h. TNF-α and NO in cell supernatant of CFn, LE-CH and LE-CH-CFn-SDC treated groups were determined using sandwich enzyme-linked immunosorbent assays (ELISAs) and Griess reagent (16) respectively.
The TNF-α in cell supernatant described earlier was determined by sandwich enzyme-linked immunosorbent assays (ELISAs) (R&D system, USA) according to standard procedures as per the manufacturer’s instructions.
Measurement of LPS-Induced Intracellular ROS
Intracellular ROS (Reactive oxygen species) stimulated by LPS was measured by detecting fluorescent intensity of either Carboxy 2,7-dichlorofluorescein diacetate (DCFH-DA) (Molecular Probes, Inc., Eugene), as described previously (17). Briefly, 1.5 × 106 J774 Φ cells/ml grown in phenol red-free DMEM medium (starvation medium) for 24 h were pre-incubated with DCFH-DA (10 μM) and LPS (1 μg/ml) and CFn, LE, LE-CH-CFn-SDC, at 37°C for 30 min in the dark. The relative fluorescent intensity of fluorophore DCF (Carboxy 2,7-dichlorofluorescein), formed due to peroxide oxidation of non-fluorescent precursor, was detected in a FACS-Calibur flow-cytometer (Becton Dickinson,UK) at an excitation wavelength of 485 nm and an emission wavelength of 530 nm. Fluorescence was calculated by means of stimulatory index (SI). In contrast, DCFH with starvation medium was used as a blank control.
Cellular Binding of LPS
Cellular Binding study was performed using Flow-cytometry Briefly J774 Φ cells (2 × 106 cells/well) were incubated with 1 μg/mL of FITC-labeled LPS in the absence or presence of formulations (LE-CH, LE-CH-CFn-SDC) at 37°C for 1 h. The cells were washed with DMEM media and then fluorescence was quantified using a FACS-Calibur flow cytometer with Cell Quest software (Becton Dickinson, Mountain View, CA).
In-Vivo Studies
E. coli-Induced Peritonitis or Abdominal Sepsis
Two experimental sets of Wistar rats with 10 and 4 animals in each group in a set received an inoculum of E. coli suspension in normal saline (2 × 1010 CFU/ml). Immediately after bacterial challenge, animals received isotonic NaCl solution (control group), CFn, LE-CFn-SDC and LE-CH-CFn-SDC (i.p.), containing CFn equivalent to 10 mg/kg. In this study first set of 10 animals in each group were monitored for survival until 72 h following the treatment. the second set of animals (4 animals in each group) were sacrificed after 2 and 6 h and CFn concentration in different tissues i.e., liver, spleen, lung and kidney were determined along with simultaneous bacterial counts in the blood, peritoneal fluid, tissue homogenate and concentration of cytokine (TNF-α, IL-6), endotoxin in plasma was estimated at 6 h. Blood samples were collected by aseptic percutaneous transthoracic cardiac puncture in sterile heparinized evacuated blood collection tubes before sacrificing the rats. Plasma was separated by centrifugation collected, frozen, and kept at −70°C till further analysis. Abdominal fluid and tissue samples from rats were collected aseptically using sterilized equipments after sacrificing animals.
CFn Concentration in Blood and Tissues
Immediately after bacterial challenge and dosage form administration by IP route for pharmacokinetic studies blood was withdrawn from rats (n = 4) by retro orbital plexus of rat eye at different time intervals (0, 0.25, 0.5, 2, 6, 12, and 24 h). The extraction and analysis of samples was done using validated HPLC method using fluorescence detector as described previously (9).
Microbial Count and LPS Estimation in Blood and Tissues
Evaluation of bacterial growth of E. coli in blood, abdominal fluid, blood and tissue was evaluated by colony enumeration by plating and incubation (35°C for 48 h) on agar plates and counting CFU at 6 h after E. coli infection. Liver and spleen were homogenized aseptically in normal saline and serially diluted, and was spread onto agar plates for estimation of bacterial load in tissues.
Endotoxin concentration in plasma was determined by the commercially available LAL test (E-TOXATE; Sigma-Aldrich). The endotoxin content was determined as described previously (15).
Cytokine Estimation in Plasma and Hepatic Tissues
Liver homogenates obtained by homogenizing liver (1 g) in 10 ml of cold phosphate buffered saline (PBS), pH 7.4, containing a protease inhibitors cocktail (Complete Roche, Germany) were centrifuged at 15,000 rpm for 15 min at 4°C and supernatant was stored at −70°C for cytokine estimation. Plasma and hepatic TNF-α levels were determined by enzyme linked immunosorbent assay (ELISA) as described above, using commercial kits that are selective rat cytokines (R& D system, USA). Plasma, cytokine concentrations has been expressed as pg/ml and hepatic cytokine amounts are expressed as pg/mg of tissue.
Statistical Analysis
MICs were given as average values from three independent measurements. Mortality rates and qualitative results for blood cultures between groups were compared by use of Fisher’s exact test (significance level fixed at 0.05). TNF-α mean values and quantitative evaluations of the bacteria in the intra-abdominal fluid cultures are presented as means ± standard deviations (SDs) of the mean; statistical comparisons between groups were made by analysis of variance (significance level was fixed at 0.05). Due to the presence of several values below the lower limit of sensitivity, plasma endotoxin levels were compared between groups by Kruskal-Wallis nonparametric test, adjusted for ties; the post hoc comparisons were performed by the Bonferroni method. Significance was accepted when the P value was <0.05.
RESULTS
Physiochemical Characterization and Stability Studies
The average globule size of all the composition of parenteral nanoemulsions was found to be in the range of 250–290 nm and was in accordance with the requirement of emulsion for parenteral administration (Globule Size ≤300 nm). Zeta potential of uncoated emulsion was found to be negative (in the range of −28 to −33 mV) indicating stability of the formulations. Formulations on coating with chitosan (0.5% w/w) via electrostatic attraction resulted in charge reversal of the formulation to a positive value (zeta potential for LE-CH; +32.8 ± 3.5 mV and for LE-CH-CFn-SDC; +30.9 ± 2.2 mV respectively) (Table II).
Ciprofloxacin entrapment was increased to ~three folds in all batches containing surf-plexes i.e. (LE-CFn-SDC ~ 85.48 ± 1.72%) and (LE-CH-CFn-SDC ~ 92.58 ± 1.86%) as compared to the formulation LE-CFn (30.56 ± 3.16%) containing only CFn. The results demonstrated that coating with chitosan further restricted the movement of drug from oil globules to bulk aqueous phase (18).
The storage stability of formulation have been observed in terms of change in globule size and pH of the emulsion samples stored at 37°C over a period of 3 months. The mean droplet size did not differ markedly when these formulations were stored for period of 3 months at 37°C. The pH of LE-CFn-SDC was in range of 7.2–7.4 while LE-CH-CFn-SDC has lower pH range (5.8–6.0) above which chitosan precipitate out. It was clearly demonstrated that the pH of emulsion was affected by the storage temperature with time. Especially at, 37°C a slight decrease in the pH value of the emulsions was observed this could be due to the formation of fatty acids as a result of the hydrolysis of triglyceride and phospholipid moieties (19) (Fig. 1). Lipopolysaccaride micelles were negatively charged under experimental conditions (phosphate buffer, pH 5.5) with a monomodal zeta-potential distribution. Electrostatically driven interactions of LPS with chitosan coated nanoemulsion LE-CH and LE-CH-CFn-SDC in ratio (1:0.5 and 1:1 w/w; LPS: chitosan ratio) of components resulted in reduction of the negative charge of LPS towards a more positive value (Table III). The particle size distribution in LPS as well as LE-CH and LE-CH-CFn-SDC, characterized by dynamic light scattering (DLS), revealed a monomodal distribution in all cases. After incubation of LPS with nanoemulsion formulations trimodal size distribution was observed, the lowest value for uninteracted LPS, middle values for interaction between free LPS and free chitosan present in nanoemulsion continuous phase and higher values for Nanoemulsion coated with LPS micelles.
Dissolution profile of CFn-SDC complex was biphasic as demonstrated in Fig. 1b followed first order kinetics with rate constant of 0.024872 min−1 and estimated t1/2 was 27.94 min with r 2 = 0.98 up to 60 min. There was significant improvement in lipophilicity of CFn on ionic complexation as partition coefficient of CFn-SDC (4.934) in chloroform was almost increased to 25 times, when compared to free CFn (0.176) as reported previously by our group (9).
In-Vitro Killing Efficiency and Free LPS Release
Release of LPS by antibiotic treatment is usually responsible for massive sensitization of macrophages leading to release of proinflammatory cytokines (i.e. TNF-alpha) and NO leading to sepsis and septic shock. Therefore, in order to evaluate LPS releasing potential of CFn loaded formulations, upon exposure to E. coli, dose dependent killing was observed (Fig. 2) and on treatment with CFn at (5 times of MIC) the free endotoxin levels were found to be higher than control group (no treatment). CFn (10 times of MIC) at a higher concentration also suppressed LPS release to a level comparable to control group. When CFn was loaded in lipid emulsion there was an improvement in efficacy of CFn but significant difference in endotoxin levels was not observed. Better antimicrobial efficacy was shown by LE-CH-CFn-SDC as compared to uncoated formulation (LE-CFn-SDC) due to inherent antimicrobial activity of chitosan in accordance to previous reports (20,21). There was a reduction in endotoxin release up to a significant levels (P < 0.05) at all dose levels for chitosan coated formulations when compared to uncoated formulations probably due to interaction of LPS with chitosan (13,22). The observed MIC values of various formulations are depicted in Fig. 2a.
Effect of Selected CFn Loaded Formulation on Pro-Inflammatory Mediator and Cellular Binding
LPS Induced NO and Cytokine Release
Nitiric oxide and cytokines specifically TNF-α are principal mediators of inflammation during sepsis. Generally rise in levels of these factors in plasma and or tissues dictate outcomes like mortality in sepsis and septic shock. When formulation (LE-CH-CFn-SDC) equivalent to 1 and 2 μg/ml CFn (containing 62.5 and 125 μg/ml total lipid respectively) was incubated with LPS (1 μg/ml) there was a dose dependent reduction in NO and TNF-α secretion. But when these values were compared to the blank formulation (LE, LE-CH) at same lipid concentration, changes in NO and TNF-α were not significant (P > 0.05) as depicted in Fig. 2b suggesting that CFn at lower concentration does not have any significant immunomodulatory effect.
LE-CH and LE-CH-CFn-SDC (≈250 μg/ml of total lipid) emulsion containing CFn equivalent to 4 μg/ml significantly reduced TNF-α and NO secretions (P < 0.05) when compared to blank formulation (LE) at same lipid concentration (Fig. 2b) demonstrating a synergistic effect of chitosan and CFn to overcome pro-inflammatory cascading events at optimized concentrations (22).
LPS Induced ROS Generation
Oxidative damage in various tissues in sepsis is generally a result of reactive oxygen species (ROS) generated in response to LPS and is a major contributor towards multiple organ failure leading to increased mortality in case of sepsis (23). The effect of formulations on LPS induced ROS was evaluated using flow cytometry. The relative mean fluorescent intensity of fluorophore DCF (Carboxy 2, 7-dichlorofluorescein), formed due to peroxide oxidation of non-fluorescent precursor was directly related to mean stimulatory index. Macrophage cells treated with LPS showed an increased level of ROS [Mean stimulatory index (SI); 21.40 ± 0.57] as compared to control cell (Mean SI; 5.20 ± 0.62). However, CFn at concentration level of 1, 2 and 4 μg/ml caused no significant changes in LPS induced ROS generation. LE-CH-CFn-SDC at CFn concentration 1, 2 μg and 4 μg/ml (containing equivalent to 62.5, 125 and 250 μg/ml of total lipid) respectively decreases, LPS induced ROS generation in dose dependent manner and were significant as compared to LPS treated control (P > 0.05) as reported previously (24) and depicted in (Fig. 3a).
Effect of different formulations (equivalent to 1.2 and 4 μg/ml of CFn) on (a) LPS induced intracellular ROS generation; intracellular ROS (reactive oxygen species) stimulated by LPS was measured by detecting fluorescent intensity of Carboxy 2,7-dichlorofluorescein diacetate (DCFH-DA). Briefly, 1.5 × 106 J774 Φ cells/ml grown in phenol red-free DMEM medium (starvation medium) for 24 h were pre-incubated with DCFH-DA (10 μM) and LPS (1 μg/ml) and CFn, LE, LE-CH-CFn-SDC, at 37°C for 30 min in the dark. (b) Cellular binding of LPS to J774 Φ by flow cytometry; Cellular Binding study was performed using Flow-cytometry Briefly J774 Φ cells (2 × 106 cells/well) were incubated with 1 μg/mL of FITC-labeled LPS in the absence or presence of formulations (LE-CH, LE-CH-CFn-SDC) at 37°C for 1 h.
Cellular Binding of LPS
The effect of LPS in macrophages are generally mediated by binding and uptake of LPS to the macrophages (25) leading to release of various inflammatory mediators which proceed into various symptoms such as LPS induced organ damage and finally death. So cellular binding study was performed to gain a mechanistic insight on the inhibition of NO and TNF-α production by developed formulations. In this study J774 MΦ cell lines was treated with FITC-labeled LPS (1 μg/ml) in the presence of 10% FCS in the presence and absence of formulations (LE-CH, LE-CH-CFn-SDC). The shifting of curve to the right indicated the binding of LPS to the cell surface. On the other hand, addition of blank (LE-CH (125 μg/ml)) and drug loaded formulation (LE-CH-CFn-SDC) lead the curve to shift to the left, when compared to LPS-FITC alone as shown in (Fig. 3b) demonstrating that LE-CH suppress binding of FITC-labeled LPS to the cells. Suppression in binding of LPS was probably due to electrostatic and/or hydrophobic interaction, which would subsequently have inhibitory effects on NO and TNF-α production. Extent of curve shifting was almost similar with drug-loaded formulations as compared to blank formulation. Since this study was done for, 30 min the influence of CFn as such, in influencing cellular binding is ruled out.
IN VIVO STUDIES
Effect of Formulation on In Vivo Viability of E. coli on Intraperitoneal Administration
CFn demonstrated the highest antimicrobial activities and therapeutic efficacies when LE-CH-CFn-SDC was administered. In fact, there were significant (P < 0.05) differences in the results for the quantitative bacterial cultures when the data obtained for the LE-CH-CFn-SDC (i.p), LE-CFn and groups were compared with those obtained for the CFn treated group respectively In addition, these formulations also reduced microbial load in RES organ like liver, spleen and lung (Table IV).
Effect of Formulations on E. coli Induced Mortality
The efficacy of optimized formulation loaded with CFn or CFn ionic-complex (i.p.) in reducing mortality of E. coli-induced peritonitis in a rat model has been performed. In this study, the rate of survival in control group (saline treated) was 30% (Table V). For groups treated with single-drug regimens of CFn (10 mg/kg i.p) given immediately after challenge had a better outcome with respect to controls (P < 0.05). Specifically, survival rates were 100%, 90%, and 70% in the groups treated with LE-CH-CFn-SDC, LE-CFn and CFn respectively by intraperitoneal route in accordance with previous reports (26) (Table V).
Effect of Formulation on E. coli Induced LPS and Cytokine Level
Over production of cytokines specifically IL-6 and TNF-α are key mediators in LPS induced sepsis and septic shock and increase the risk of mortality in the subjects, therefore cytokine levels can effectively indicate the risk of mortality in sepsis . The mean peak levels of endotoxin, TNF-α and IL-6 at 6 h were 0.450 ng/ml, 953.61 pg/ml and 344.20 pg/ml respectively in the positive control group. CFn (i.p.) treated group showed two fold reduction in plasma endotoxin level (0.275 ng/ml), TNF-α ((341.66 pg/ml) pg/ml) and IL-6 (160.77 pg/ml). Treatment with, LE-CFn-SDC, and septic mice resulted in highest reduction in plasma endotoxin (0.225 ng/ml), TNF α (225.52 pg/ml) and IL-6 (101.70 pg/ml). The administration of CFn in lipid based emulsion by parenteral route not only produced high antimicrobial activities and the strongest reduction in plasma endotoxin and TNF-α levels, resulting in the higher survival rates (11) (more than 90%) (Table V).
Pharmacokinetics and Bio-Distribution Studies of CFn Loaded Lipid Emulsion by i.p Route
On administration of LE-CFn-SDC and LE-CH-CFn-SDC the serum concentration of CFn, was significantly higher at all time points compared to CFn solution and was detectable up to 24 h. (Fig. 4a). The half-life (t1/2) of CFn with LE-CH-CFn-SDC and LE-CFn-SDC was 6.4 and 5.9 h respectively as compared to CFn solution (2.16 h). The AUC of CFn was 37.52 μg.h/ml and 15.39 μg.h/ml with LE-CH-CFn-SDC and LE-CFn-SDC respectively, which is significantly higher than CFn solution (5.66 μg.h/ml). The elimination rate constant (kel) of CFn was found to be 0.14 h−1 and 0.11 h−1 for LE-CFn-SDC and LE-CH-CFn-SDC respectively which is significantly lower than CFn solution (0.43 h−1).
(a) Serum concentration vs. time profile of intraperitoneally injected CFn (10 mg/kg) delivered in solution (filled diamond), LE-CFn-SDC (filled squares) and LE-CH-CFn-SDC (filled triangle) lipid based emulsion. (b) Tissue distribution of intraperitoneally injected CFn (10 mg/kg) delivered in solution, LE-CFn-SDC and LE-CH-CFn-SDC lipid based emulsion at 2 h (c). Tissue distribution of intraperitoneally injected CFn (10 mg/kg) delivered in solution, LE-CFn-SDC and LE-CH-CFn-SDC lipid based emulsion at 6 h. Each value is average of four determinations (n = 4); (mean ± SD).
The experimental results indicated alteration in bio-distribution of CFn when delivered through lipid-based nanoemulsion when given by i.p. route. When CFn, was administered the amount of CFn in liver, spleen, lung and abdominal fluid at 2 h was found to be 7.92 ± 0.93 μg/g, 3.45 ± 0.34 μg/g, 5.74 ± 0.87 μg/g and 0.42 ± 0.03 μg/ml respectively. When, LE-CFn-SDC was administered i.p, the amount of CFn recovered at 2 h was almost 2.5 and 4 times in liver (19.12 ± 0.60 μg/g) and spleen (13.78 ± 1.35 μg/g) while it was 1.5 and 2.5 times in lung (7.90 ± 0.69 μg/g) and abdominal fluid (1.02 ± 0.12 μg/ml) respectively when compared to CFn solution.
The amount of CFn recovered after treatment with LE-CH-CFn-SDC at 2 h was 4.5 and 3 times in liver (34.19 ± 1.20 μg/g) and spleen (10.27 ± 1.10 μg/g) respectively. Nanoemulsion also enhanced CFn amount to almost 1.2 and 2.5 times in lung (7.82 ± 0.77 μg/g) and abdominal fluid (1.89 ± 0.22 μg/ml) respectively compared to plain CFn as shown in Fig. 4b.
There was a gradual reduction in CFn recovery in different tissues at 6 h however; LE-CFn was able to maintain higher level of CFn compared to CFn solution. At 6 h the amount of CFn recovered in liver, spleen, lung and abdominal fluid was 0.03 ± 0.01, 0.19 ± 0.05, 0.07 ± 0.02 μg/g and 0.01 ± 0.01 μg/ml respectively. The CFn recovery with LE-CFn-SDC at 6 h was almost 70 and 11 times in liver (2.11 ± 0.67 μg/g) and spleen (1.21 ± 0.85 μg/g) respectively while it was 7 and 67 times in lungs (0.54 ± 0.05 μg/g) and abdominal fluid (0.67 ± 0.08 μg/ml) respectively compared to plain CFn.
On administration of LE-CH-CFn-SDC the amount of CFn recovered was almost 84 and 10 times in liver (2.51 ± 0.21 μg/g) and spleen (1.97 ± 0.13 μg/g) respectively while it was 30 and 95 times in lung (2.11 ± 0.22 μg/g) and abdominal fluid (0.95 ± 0.19 μg/ml) respectively compared to CFn solution as shown in Fig. 4c. After i.p. administration, the distribution of CFn with LE-CFn-SDC was rapid in spleen and liver, while the distribution in lung increased after 1 h of injection. However, the chitosan being an integral part of LE-CH-CFn-SDC further improved CFn level in liver and spleen and maintained higher level of CFn for prolonged period. Interestingly, the disappearance of CFn loaded into LE-CH-CFn-SDC lipid emulsion from peritoneal cavity was slower than CFn in LE-CFn-SDC (Fig. 4b and c).
The lower Kel of CFn delivered through lipid emulsion compared to CFn solution indicates their slow clearance from the body, as is supported by the improved half-life and AUC0–t, AUC0–∞ and AUMC0–∞.
DISCUSSION
Antimicrobial therapy is widely used for treatment of abdominal peritonitis; however, the release of LPS due to such treatment is a problem with current antimicrobial therapies. The increase in LPS differs according to the type of antimicrobial agent used. However, the relation between CFn induced LPS release and its effect on inflammatory cytokines was not clear. Against this background, we performed an experimental study to observe the action of CFn on microbial load, production of LPS and inflammatory cytokines associated with E. coli induced peritonitis in vivo. In this work, we have developed a CFn-SDC complex loaded chitosan coated nanoemulsion for therapeutic intervention of sepsis. There was significant improvement in lipophilicity of CFn on ionic complexation as partition coefficient of CFn-SDC (4.934) in chloroform was almost increased to 25 times, when compared to free CFn (0.176) as reported previously by our group (9). This lead to higher entrapment of drug and modification of release behavior from nanoemulsions as reported previously (9).
In PBS solution, dissolution kinetics of CFn-SDC complex was biphasic as described in earlier reports (27) and followed first order kinetics. The solid CFn-SDC hydrophobic ion pair complex rapidly dissolves in the solution up to the solubility limit. Once in solution, anions (e.g., Cl−) exchange with the hydrophobic organic ions leading to further increase in free drug concentration in the aqueous phase.
The pharmaceutical parameters of the formulated emulsion such as globule size and size distribution was in accordance to their application for parenteral administration (i.e. <300 nm) (28). The imparted positive charge on nanoemulsion was due to the presence of amino groups present in chitosan, which results in reversal of zeta potential in formulations. Due to innate physicochemical properties of PF-68 and phospholipids, the interfacial film formed was strong enough to prevent droplet coalescence upon any physical and thermal conditions. This interface is further reinforced by the presence of Chitosan. PF-68 also prevented unfavorable interactions between free fatty acids present in soya oil and cationic agents by forming a tough layer at the interface (18).
The significance of chitosan coated nanoemulsion in septic shock can be inferred from in vitro interaction studies that clearly provide evidences that it interact electrostatically with oppositely charged LPS as shown by change in zeta potential values (13). Chitosan coated lipid based emulsion with or without CFn suppresses free LPS release in microbial culture when compared to CFn solution. There was dose dependent reduction in free endotoxin release as CFn concentration increased (i.e. 1 to 4 μg/ml) in formulations loaded with CFn. This observation was in accordance with previous findings that showed binding of CFn to PBP3 protein at lower dose, leading to increase in free LPS release in contrast to at higher concentrations of CFn where it binds with PBP1a and PBP2 protein leading to conglomeration of the bacteria reducing free endotoxin release (29). It is also evident that when surface of lipid emulsion was modified with chitosan (cationic polymer) the barrier properties of outer membrane of bacteria was disturbed via charge interactions and increase permeability of different antimicrobial agents, resulting in their decreased MIC values (21). Interestingly, LE-CH and LE-CH-CFn-SDC improves antimicrobial efficacy and at the same time decreases release of free LPS as compared to free drug (CFn). This empirical data provides evidence that after killing of bacteria, this formulation is either able to mop up or neutralize the free LPS. It may be apparent that due to polycationicity, the LE-CH based formulation has ability to interact with LPS as described previously (13,22).
In septic shock, severe systemic inflammatory responses are triggered by the interaction of LPS with macrophages via CD14 receptor with the help of TLR4 leading to the progressive release of a variety of pro-inflammatory cytokines, such as IL-8, IL1-β, and IL-6 (30). Reduction in interaction of LPS with macrophages has been reported to reduce severity of sepsis (5,6). However, propensity to decrease free endotoxin is unclear, may be it strongly interacts with endotoxin and forms a complex (22).
There are numerous reports indicating that NO (31) and TNF-α act as the primary mediators of the pathophysiological outcomes observed during septic shock (30,32,33). Lipid based emulsions suppressed production of NO and pro-inflammatory cytokine like TNF-α in murine macrophages J774 cells stimulated with LPS in-vitro. Further, it has also been observed that lipid emulsion especially LE-CH lowers cellular binding of LPS in J774 macrophages cells when they exist together in culture medium even though the pH is neutral or basic. Therefore, it is possible that these emulsions impair cellular signaling because of an interference of the initial step of LPS signaling by reducing the amount of LPS available for binding to CD14 thereby resulting in inhibition of NO and TNF-α.
This interference may be attributed to favorable interaction between LPS and phospholipids either directly (34) or indirectly (35). The LPS-chitosan complex (24,36–38) is anticipated to act as competitor for binding to receptors. Since the lethal effect of endotoxin can be prevented by phospholipids (39), we presume that a lipid-glycolipid interaction must be at least partially responsible for this phenomenon in addition to the effects of phospholipids on molecular targets in sepsis (35,40).
It has been observed that lipid based emulsion are able to circumvent the LPS induced toxicity and can be a effective delivery vehicle for CFn in terms of release (28), efficacy and reduced toxicity due to better tissue distribution and further can have positive influence in nullifying the effect of endotoxin. Phospholipid rich emulsions are also known to enhance triglyceride rich lipoproteins mediated secretion of LPS into the bile via liver parenchymal cells. In this way, Kupffer cells are not activated and the production of cytokines is greatly inhibited (41). Chitosan modified lipid emulsions (LE-CH; i.p.) enhanced survival time and decreased serum and hepatic TNF-α. These results therefore suggests possible interaction of LPS with chitosan in the intraperitoneal cavity. Our in-vitro data correlates well with in-vivo data. LPS complexed with CH shown to possess much lower toxicity in comparison to free LPS in mice at the similar concentration (22). However, there are no reports, that shows ability of LPS to interact with chitosan in-vivo especially in peritoneal cavity. Lipid based emulsion, when loaded with CFn (as CFn-SDC ionic complex) LE-CFn-SDC, LE-CH-CFn-SDC (i.p.) further resulted in improved efficacy of CFn in terms of animal survival, decreased serum and hepatic TNF-α levels in rat model of E. coli induced peritonitis. These results suggest that LE-CH-CFn-SDC (i.p) significantly suppresses lethality, probably resulting from the reduction of tissue injury specifically in liver. In addition, this effect may be due to the synergistic effect of CFn in lipid-based emulsion which rendered better distribution of CFn to infected tissues. We have observed slow and prolonged clearance of CFn delivered through LE-CH-CFn-SDC emulsion and higherserum concentrations up to 24 h as compared to CFn solution. This can be attributed to the fact that, in the developed system CFn-SDC complex has been used within the lipid phase which is surrounded by aqueous film comprising chitosan. That means there are three barriers actively involved in the controlled release of CFn i.e. (a) dissociation of CFn from the complex, since release of drug was controlled by the slow dissociation of CFn and SDC complex at interface (b) diffusion of CFn from oil to aqueous phase and (c) diffusion of CFn from polymeric chitosan film. We anticipate that control release pharmacokinetic profile of the proposed system could be one and/or combination of all the processes. These results are in agreement with the previous report (42).
This may be advantageous in the localized infection i.e. E. coli induced peritonitis or abdominal sepsis. These formulations resulted in facilitated distribution in the organs of RES specifically liver and spleen (major bacterial reservoirs in case of intra-abdominal sepsis) as demonstrated in pharmacokinetic studies. LE-CFn-SDC and LE-CH-CFn-SDC demonstrated significant enhancement of bioavailability of CFn compared to CFn solution after i.p. injection indicating advantage of formulation in improving drug concentrations in blood. These data are in agreement with the previous studies by Tilney (1971) (43), who reported enhancement in plasma levels of drug on i.p. administration. This may be attributed to prevention of rapid distribution of CFn to tissues (specifically to non-RES organs and adipose tissues) surrounding intraperitoneal cavity resulting in low plasma concentration of CFn in plasma. In case of chitosan coated nanoemulsions (that behaved like nanocapsules of less than 300 nm) as reported previously in case of liposome’s (44), may bypass entrapment by lymph nodes and can be drained into blood via thoracic lymph channels from lymphatic circulations. Chitosan coated nanoemulsion might also pass directly through peritoneal surface of diaphragm and enter lymphatic’s between phrenic muscle bundles and empty into the diaphragmatic plexus (43) ultimately leading to blood thereby increasing plasma concentration of drug. It has also been shown that particles injected into the peritoneal cavity may also pass directly through the fenestrated basement membrane of mesothelial cells on the peritoneal surface of the diaphragm, enter lymphatic’s between phrenic muscle bundles and empty into the diaphragmatic plexus (45).
Moreover, the cationic nanoemulsions are anticipated to possess excellent capabilities to overcome various extracellular and intracellular barriers to improve the plasma drug concentration. Cationic chitosan provides sufficient positive charges in the nanoemulsion to be directed onto negatively charged cell membranes which collectively facilitates the internalization of nanoemulsion (46,47). Intraperitoneally delivered cationic nanoemulsion might get absorbed directly via peritoneum and are anticipated to exhibit higher cell binding affinity and might have translocated within, through migration of PECs (peritoneal exudates macrophage cells).
CFn when administered in formulations LE-CFn-SDC and LE-CH-CFn-SDC (i.p.) displayed a strong synergistic activity with formulation against E. coli induced septicemia in rats. It reduces microbial count in serum as well as in tissues i.e. liver and spleen due to improved pharmacokinetics of CFn and at the same time reduced the levels of both circulating LPS and TNF-α in serum leading to reduced mortality, which highlights dual advantages of antimicrobial and antiendotoxin activity of CFn in cationic lipid based emulsion.
Our studies, indicate that chitosan coated lipid based emulsions could be positioned as nano sized delivery system with huge potential to treat lethal implications of endotoxin shock by suppression of macrophage activation. It is found to be mainly acting via two mechanisms (i) direct interaction of LPS with modified lipid emulsion either through electrostatic and/or lipid-lipid interaction (ii) suppression of LPS binding to cells and thus meddling in activation of macrophage (39).
CONCLUSION
Cationic nanoemulsions have re-emerged as an attractive option for delivery of drugs specifically for the management of sepsis caused by gram –ve organisms. Optimized nanoemulsion also altered the pharmacokinetics of drug in plasma as well as in tissues. It specifically accumulates in liver and spleen as they are the major organs in which the abscesses are present leading to reduction in bacterial burden and improved survival in animal models. The prototype delivery system was experimentally found to be useful in reducing bio-burden during infection and ameliorating severity of sepsis by mopping up the LPS thereby reducing the secretion of inflammatory cytokines. Its application as intracorporeal agent for therapeutic intervention of sepsis in clinical settings is in offing. This is CSIR-CDRI Communication Number 8636.
Abbreviations
- CFn:
-
Ciprofloxacin
- CFn-SDC:
-
Ciprofloxacin-sodium deoxycholate surfplex
- CFU:
-
Colony forming units
- CH:
-
Chitosan
- DCF:
-
Carboxy 2,7-dichlorofluorescein
- DLS:
-
Dynamic light scattering
- DMEM:
-
Dulbecco’s modified eagle medium
- EE:
-
Entrapment efficiency
- FBS:
-
Fetal bovine serum
- FITC DCFH-DA:
-
Carboxy 2,7-dichlorofluorescein diacetate
- IL-6:
-
Interleukin-6
- LPS:
-
Lipopolysaccharide
- MIC:
-
Minimum inhibitory concentration
- mV:
-
Milli-volt
- NO:
-
Nitric oxide
- PF-68:
-
Pluronic F-68
- ROS:
-
Reactive oxygen species
- SI:
-
Stimulatory index
- TLR-4:
-
Toll like receptor-4
- TNF-α:
-
Tissue necrosis factor alpha
REFERENCES
Oliva M, Rekha A, Yellin A, Pasternak J, Campos M, Rose G, et al. A multicenter trial of the efficacy and safety of tigecycline versus imipenem/cilastatin in patients with complicated intra-abdominal infections [study ID numbers: 3074A1-301-WW; ClinicalTrials.gov Identifier: NCT00081744]. BMC Infect Dis. 2005;5:88.
Bosscha K, Reijnders K, Hulstaert P, Algra A, Van der Werken C. Prognostic scoring systems to predict outcome in peritonitis and intra–abdominal sepsis. Br J Surg. 1997;84:1532–4.
Xu J, Hoyert DL. Deaths: preliminary data for 2011. Natl Vital Stat Rep. 2012;61:1–64.
McClean KL, Sheehan GJ, Harding GKM. Intraabdominal infection: a review. Clin Infect Dis. 1994;19:100–16.
Cruz DN, Perazella MA, Bellomo R, De Cal M, Polanco N, Corradi V, et al. Effectiveness of polymyxin B-immobilized fiber column in sepsis: a systematic review. Crit Care. 2007;11:R47.
Shimokawa K-I, Takakuwa R, Wada Y, Yamazaki N, Ishii F. Adsorption of various antimicrobial agents to endotoxin removal polymyxin-B immobilized fiber (Toraymyxin®). Part 2: adsorption of two drugs to Toraymyxin PMX-20R cartridges. Colloids Surf B: Biointerfaces. 2013;101:350–2.
Brummelhuis WJ, Joles JA, Stam J, Verrips CT, Braam B. Biological and technical considerations regarding the removal of bacteriotoxins in sepsis with emphasis on toxic shock syndrome toxin 1. Shock. 2012;37:247.
Blot S, De Waele JJ, Vogelaers D. Essentials for selecting antimicrobial therapy for intra-abdominal infections. Drugs. 2012;72:e17–32.
Jain V, Singodia D, Gupta GK, Garg D, Keshava GBS, Shukla R, et al. Ciprofloxacin surf-plexes in sub-micron emulsions: a novel approach to improve payload efficiency and antimicrobial efficacy. Int J Pharm. 2011;409:237–44.
Shukla P, Gupta G, Singodia D, Shukla R, Verma AK, Dwivedi P, et al. Emerging trend in nano-engineered polyelectrolyte-based surrogate carriers for delivery of bioactives. Expert Opin Drug Deliv. 2010;7:993–1011.
Gordon BR, Parker TS, Levine DM, Feuerbach F, Saal SD, Sloan B-J, et al. Neutralization of endotoxin by a phospholipid emulsion in healthy volunteers. J Infect Dis. 2005;191:1515–22.
Buttenschoen K, Radermacher P, Bracht H. Endotoxin elimination in sepsis: physiology and therapeutic application. Langenbeck’s Arch Surg. 2010;395:597–605.
Davydova VN, Bratskaya SY, Gorbach VI, Solov’eva TF, Kaca W, Yermak IM. Comparative study of electrokinetic potentials and binding affinity of lipopolysaccharides–chitosan complexes. Biophys Chem. 2008;136:1–6.
Zhou H, Lengsfeld C, Claffey DJ, Ruth JA, Hybertson B, Randolph TW, et al. Hydrophobic ion pairing of isoniazid using a prodrug approach. J Pharm Sci. 2002;91:1502–11.
Pearson F, Dubczak J, Weary M, Bruszer G, Donohue G. Detection of endotoxin in the plasma of patients with gram-negative bacterial sepsis by the Limulus amoebocyte lysate assay. J Clin Microbiol. 1985;21:865–8.
Mettu SR, Wig JD, Khullar M, Singh G, Gupta R. Efficacy of serum nitric oxide level estimation in assessing the severity of necrotizing pancreatitis. Pancreatology. 2003;3:506–14.
Mooreand SF, MacKenzie AB. NADPH oxidase NOX2 mediates rapid cellular oxidation following ATP stimulation of endotoxin-primed macrophages. J Immunol. 2009;183:3302–8.
Fäldt P, Bergenståhl B, Claesson PM. Stabilization by chitosan of soybean oil emulsions coated with phospholipid and glycocholic acid. Colloids Surf A Physicochem Eng Asp. 1993;71:187–95.
Washington C. The stability of intravenous fat emulsions in total parenteral nutrition mixtures. Int J Pharm. 1990;66:1–21.
Kong M, Chen XG, Xing K, Park HJ. Antimicrobial properties of chitosan and mode of action: a state of the art review. Int J Food Microbiol. 2010;144:51–63.
Liu H, Du Y, Wang X, Sun L. Chitosan kills bacteria through cell membrane damage. Int J Food Microbiol. 2004;95:147–55.
Yermak IM, Davidova VN, Gorbach VI, Luk’yanov PA, Solov’eva TF, Ulmer AJ, et al. Forming and immunological properties of some lipopolysaccharide–chitosan complexes. Biochimie. 2006;88:23–30.
Sun Y, Lu Y, Engeland CG, Gordon SC, Sroussi HY. The anti-oxidative, anti-inflammatory, and protective effect of S100A8 in endotoxemic mice. Mol Immunol. 2013;53:443–9.
Qiao Y, Bai X-F, Du Y-G. Chitosan oligosaccharides protect mice from LPS challenge by attenuation of inflammation and oxidative stress. Int Immunopharmacol. 2011;11:121–7.
Koo JE, Park ZY, Kim ND, Lee JY. Sulforaphane inhibits the engagement of LPS with TLR4/MD2 complex by preferential binding to Cys133 in MD2. Biochem Biophys Res Commun. 2013;434:600–5.
Goldfarb RD, Parker TS, Levine DM, Glock D, Akhter I, Alkhudari A, et al. Protein-free phospholipid emulsion treatment improved cardiopulmonary function and survival in porcine sepsis. Am J Physiol Regul Integr Comp Physiol. 2003;284:R550–7.
Lengsfeld C, Pitera D, Manning M, Randolph T. Dissolution and partitioning behavior of hydrophobic ion-paired compounds. Pharmaceut Res. 2002;19:1572–6.
Mishra PR. An investigation on the approach to target lipopolysaccharide through polymeric capped nano-structured formulation for the management of sepsis. J Biomed Nanotechnol. 2011;7:47–9.
Trautmann M, Heinemann M, Moricke A, Seidelmann M, Lorenz I, Berger D, et al. Endotoxin release due to ciprofloxacin measured by three different methods. J Chemother. 1999;11:248–54.
Van Amersfoort ES, Van Berkel TJC, Kuiper J. Receptors, mediators, and mechanisms involved in bacterial sepsis and septic shock. Clin Microbiol Rev. 2003;16:379–414.
Wang HP, Liu HY, Jia ZJ, Olsen C, Litwin S, Guan GJ, et al. Nitro-oleic acid protects against endotoxin-induced endotoxemia and multiorgan injury in mice. Am J Physiol Renal Physiol. 2010;298:F754–62.
Chaudhry H, Zhou J, Zhong Y, Ali MM, McGuire F, Nagarkatti PS, et al. Role of cytokines as a double-edged sword in sepsis. In Vivo. 2013;27:669–84.
Lee CH, Hsiao CC, Hung CY, Lo HC. Combination treatment of parenteral arginine and nitric oxide inhibitor N-G-nitro-L-arginine methyl ester in rats with peritonitis. J Surg Res. 2013;181:99–105.
Takeuchiand Y, Nikaido H. Persistence of segregated phospholipid domains in phospholipid-lipopolysaccharide mixed bilayers: studies with spin-labeled phospholipids. Biochemistry. 1981;20:523–9.
Mueller M, Brandenburg K, Dedrick R, Schromm AB, Seydel U. Phospholipids inhibit lipopolysaccharide (LPS)-induced cell activation: a role for LPS-binding protein. J Immunol. 2005;174:1091–6.
Stefan M, Melnig V, Pricop D, Neagu A, Mihasan M, Tartau L, et al. Attenuated effects of chitosan-capped gold nanoparticles on LPS-induced toxicity in laboratory rats. Mater Sci Eng C. 2013;33:550–6.
Ma P, Liu H-T, Wei P, Xu Q-S, Bai X-F, Du Y-G, et al. Oligosaccharides inhibit LPS-induced over-expression of IL-6 and TNF-α in RAW264.7 macrophage cells through blockade of mitogen-activated protein kinase (MAPK) and PI3K/Akt signaling pathways. Carbohydr Polym. 2011;84:1391–8.
Qiao Y, Ruan Y, Xiong C, Xu Q, Wei P, Ma P, et al. Chitosan oligosaccharides suppressant LPS binding to TLR4/MD-2 receptor complex. Carbohydr Polym. 2010;82:405–11.
Bochkov VN, Kadl A, Huber J, Gruber F, Binder BR, Leitinger N. Protective role of phospholipid oxidation products in endotoxin-induced tissue damage. Nature. 2002;419:77–81.
Jacksonand SK, Parton J. Lysophospholipid acyltransferases in monocyte inflammatory responses and sepsis. Immunobiology. 2004;209:31–8.
Harris HW, Brady SE, Rapp JH. Hepatic endosomal trafficking of lipoprotein-bound endotoxin in rats. J Surg Res. 2002;106:188–95.
Simovicand S, Prestidge CA. Nanoparticle layers controlling drug release from emulsions. Eur J Pharm Biopharm. 2007;67:39–47.
Tilney NL. Patterns of lymphatic drainage in the adult laboratory rat. J Anat. 1971;109:369.
Parker R, Priester E, Sieber S. Comparison of lymphatic uptake, metabolism, excretion, and biodistribution of free and liposome-entrapped [14C] cytosine beta-D-arabinofuranoside following intraperitoneal administration to rats. Drug Metab Dispos. 1982;10:40–6.
Olinand T, Saldeen T. The lymphatic pathways from the peritoneal cavity: a lymphangiographic study in the rat. Cancer Res. 1964;24:1700–11.
Zhao X, Yin L, Ding J, Tang C, Gu S, Yin C, et al. Thiolated trimethyl chitosan nanocomplexes as gene carriers with high in vitro and in vivo transfection efficiency. J Control Release. 2010;144:46–54.
Yin L, Ding J, He C, Cui L, Tang C, Yin C. Drug permeability and mucoadhesion properties of thiolated trimethyl chitosan nanoparticles in oral insulin delivery. Biomaterials. 2009;30:5691–700.
Funding
The author acknowledges the funding received by ICMR (under Adhoc project) and Vikas Jain is acknowledges the support (as senior research fellowship) received from CSIR. New Delhi, India. The author also acknowledges funding from CSIR network project UNDO (BSC 0103).
Author information
Authors and Affiliations
Corresponding author
Rights and permissions
About this article
Cite this article
Jain, V., Shukla, P., Pal, R. et al. Cationic Nanoemulsions Bearing Ciprofloxacin Surf-Plexes Enhances Its Therapeutic Efficacy in Conditions of E. coli Induced Peritonitis and Sepsis. Pharm Res 31, 2630–2642 (2014). https://doi.org/10.1007/s11095-014-1360-0
Received:
Accepted:
Published:
Issue Date:
DOI: https://doi.org/10.1007/s11095-014-1360-0