Abstract
Purpose
To develop a unique in vitro aqueous fluid-capacity-limited dissolution system for the kinetic assessment of respirable aerosol drug particles from inhaler products.
Methods
Aerosol particles of 5 inhaled corticosteroids (ICSs) from 7 inhaler products were collected in the aerodynamic 2.1–3.3 or 4.7–5.8 μm on the filter membranes using the Andersen cascade impactor. Each filter membrane was then placed onto the donor compartment of the Transwell® system, where addition of 0.04ml aqueous fluid initiated aerosol ICS dissolution and permeation across its supporting membrane at 37°C and ∼100% humidity.
Results
The % profiles of dissolution and permeation were apparent first-order or pseudo-zero-order, reaching varying 1.9–95.0% by 5 h. Their kinetics overall conformed to the ICS aqueous solubility. With increasing aerosol mass, however, the profiles decelerated, attributed to undissolved ICSs left by the limited dissolution fluid capacity. The profiles could be also product-specific, as beclomethasone dipropionate aerosols from QVAR® dissolved faster than those from VANCERIL®, whereas fluticasone propionate aerosols from two different inhaler products exhibited comparable profiles. The 2.1–3.3 μm aerosols dissolved faster than the 4.7–5.8 μm aerosols.
Conclusions
Aerosol ICS dissolution into the limited aqueous fluid volume differed kinetically due to ICS solubility and aerosol mass, size, formulation and/or generation.
Similar content being viewed by others
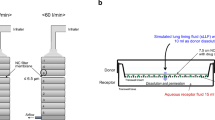
Avoid common mistakes on your manuscript.
INTRODUCTION
For oral solid dosage forms, it is well accepted that their product performance assessments should include testing of dissolution, given that their active pharmaceutical ingredients (APIs) must be dissolved for gastrointestinal absorption and thus therapeutic actions (1,2). Several in vitro dissolution testing methods are available in the compendia for use in the selection of APIs and formulations, product quality control, and between-products assessment for biopharmaceutical equivalence or difference (2,3). Meanwhile, despite much lower drug mass, compared to oral solid dosage forms, this biopharmaceutical concept of API dissolution being prerequisite for cellular uptake and/or absorption is also true for most orally inhaled dosage forms, yet on the lung surface with a limited lining fluid volume following aerosol deposition. Two most popular inhaled dosage forms are pressurized metered dose inhalers (pMDIs) and dry powder inhalers (DPIs), both of which deliver their APIs, along with formulation excipients, onto the lung surface in a solid or semi-solid form. Hence, the lung cellular disposition of such inhaled molecules (i.e., APIs) can be considered as a sequential cascade of aerosol deposition onto, and API dissolution into, the limited volume of the lung lining fluid (10–30 ml in humans), preceding cellular uptake and/or absorption (4). In this context, evidence exists in the literature that the aqueous solubility values of APIs for inhaled dosage forms are considerably diverse and possibly altered by formulation excipients and/or via aerosol generation (4–8). As a result, the kinetics of dissolution for such aerosol particles could differ from those for the APIs, and in certain cases, rate-determining in their lung biopharmaceutics following inhalation (6–8).
Given this logical involvement and possible importance of the dissolution kinetics of inhaled aerosol drugs in lung biopharmaceutics, Davies and Feddah (9) reported in vitro dissolution profiles of three inhaled corticosteroid (ICS) aerosol particles generated and collected from commercial pMDIs and DPIs. It was innovative in the use of the Andersen cascade impactor (ACI), a compendial apparatus to determine aerodynamic particle size distributions for inhaler products, to collect ICS aerosol particles from inhalers onto filter membranes. This aerosol collection was followed by dissolution profile determination using a custom-made flow-through dissolution system, similar to the USP Dissolution Testing Apparatus 4 (2). Unfortunately, as the ICS aerosols were collected by filtration at the exit of the USP induction port prior to the ACI entry, the collected aerosol particle sizes were left largely unknown, not necessarily respirable, and likely inconsistent between products. Moreover, the use of the flow-through dissolution system provided rather favorable flowing fluid dynamics that were unlimited in volume for dissolution. On the contrary, the lung lining fluid is known to be fairly stationary and limited in volume only by 10–30 ml in humans, which should serve the maximum dissolution capacity for deposited drugs (4,6,8). Therefore, ideally, the method should enable the kinetic assessments of dissolution for defined-size and respirable aerosol drug particles generated from inhaler products, into the limited volume of the stationary fluid, like actual aerosol particle dissolution on the lung surface.
Accordingly, this study was aimed to develop a more unique dissolution testing method for aerosol drug particles generated from commercial inhaler products, with a focus on collection of defined-size and respirable particles and their dissolution in the limited volume of the stationary aqueous fluid. The compendial ACI was employed, as by Davies and Feddah (9), to collect aerosol drug particles, yet in the defined aerodynamic diameters of 2.1–3.3 and 4.7–5.8 μm by impaction on the filter membranes placed on the Stage 4 and 2, respectively. Then, these filter membranes with the deposited aerosol drugs were placed on the donor compartment of the Transwell® permeable support system, where addition of the limited 0.04 ml aqueous fluid initiated aerosol particle dissolution and drug permeation across its supporting membranes; the Transwell® system was used in this study as a testing apparatus for such aerosol drug particle dissolution on its donor compartment. A total of 7 commercial inhaler products of 5 ICSs (as listed in Table I) were tested upon various drug mass deposition, in order to pursue the hypothesis that the kinetics of dissolution for these aerosol drug particles depend on not only the ICS aqueous solubility but also the ICS deposited mass, aerosol size, formulation, dosage form and/or generation.
MATERIALS AND METHODS
Commercial Inhaler Products and ICS Analysis
Table I lists 7 commercial inhaler products of 5 ICSs tested in this study along with their certain physicochemical and formulation properties. While these are packaged in either pMDIs or DPIs, the formulations are varied in physical presence of ICS (i.e., in suspension, solution or powder), excipient and metered dose. The molecular weights of the ICSs are almost consistent within a range of 431–521 Da. However, their reported aqueous solubility values differ by maximum 1,000-fold, ranging from 0.13 to 140 μg/ml. All the products were purchased through the VCU Health System Pharmacy and used prior to the expiration dates, except VANCERIL® pMDIs that were used after expiration due to the product withdrawal from the US market; it was confirmed however, that their API, BDP was negligibly degraded, and aerosol particle size distribution remained unaltered, compared to that prior to the expiration (data not shown). Table II summarizes analytical methods for quantification of each of the ICSs to determine the profiles of dissolution and permeation in this study, as developed and validated in-house.
ICS Aerosol Particle Collection on Membrane Filters by Impaction
Fig. 1 schematically shows the collection of ICS aerosol particles in the defined aerodynamic diameter range of 2.1–3.3 μm onto the PVDF filter membranes by impaction following generation from each of the inhaler products listed in Table I. An 8-stage, non-viable Andersen cascade impactor (ACI Mark II; Thermo Electron Corporation, Franklin, MA) with stainless steel collection plates was used, coupled with the USP induction port and the mouthpiece adaptor tailored to each inhaler. These were assembled, together with a pump (General Electric Company, Fort Wayne, IN), which was adjusted to operate at 28.3 ± 0.2 l/min of the airflow rate. During this ACI assembly, the stainless steel collection plate on the Stage 4 only was turned upside down, such that six polyvinylidene difluoride (PVDF) filter membranes (Durapore®; 25 mm in diameter; 0.22 μm pore; Millipore Corporation, Billerica, MA) were placed, as shown in Fig. 1. As this maintained the nozzle-to-plate distance of the conventional calibrated ACI configuration, these filter membranes enabled the collection of the 2.1–3.3 μm aerosol particles at 28.3 l/min (13). The ICS aerosols were generated from the pMDIs and DPIs with various actuations and doses, respectively, and introduced into the ACI for their deposition on the filter membranes. In one experiment, the PVDF membranes were placed on the Stage 2 to collect the 4.7–5.8 μm aerosol particles.
Impaction collection of inhaled corticosteroid (ICS) aerosol particles in the 2.1–3.3 μm aerodynamic diameters from commercial inhaler products onto 6 PVDF filter membranes using the Andersen cascade impactor (ACI). The stainless steel collection plate of the Stage 4 was turned upside down to place the PVDF membranes, while maintaining its size cut-off at 28.3 l/min of the airflow rate. The magnified schematics show the ICS aerosol collection on the membranes placed at the Stage 4. Deposited ICS aerosols are shown as solid triangles.
Dissolution and Permeation Profiles of ICS Aerosol Particles
Following ICS aerosol deposition, the ACI was disassembled, and each of the filter membranes was placed, with the deposited drug face down, onto the semi-permeable polyester supporting membrane (25 mm in diameter; 0.4 μm pore) on the donor compartment of the Transwell® insert (Corning, Inc., Acton, MA), as shown in Fig. 2. The Transwell® base contained 1.4 ml of phosphate-buffered saline (PBS; pH 7.4) for the FN, TA and BD aerosols and distilled deionized water (DDW; pH 7.0) for the FP and BDP aerosols. The latter medium was chosen for FP and BDP, as their solubility values were unaltered from those in PBS, yet providing analytical simplicity in their HPLC-MS/MS (data not shown). Immediately, 0.04 ml of PBS or DDW was added over the PVDF membrane on the donor compartment to initiate the ICS aerosol particle dissolution and the Transwell® supporting membrane permeation. The entire Transwell® systems were then placed in an incubator (NAPCO Model 5410; Precision Scientific, Inc., Chicago, IL) maintained at 37°C and near 100% relative humidity. An aliquot (0.5 ml) of sample was taken from the receptor compartment at different time points during the 5 or 10 h period; the receptor compartment was immediately replenished with 0.5 ml of fresh and pre-warmed PBS or DDW each post-sampling, in order to maintain its 1.4 ml volume. At the end of each experiment, the donor compartment was thoroughly washed with 1.0 ml admixture of 60% acetonitrile (CH3CN) and 40% DDW to recover the ICSs remaining to be dissolved and permeated. All the samples were analyzed for ICS quantification by the validated methods shown in Table II, and the profiles of dissolution and permeation were determined, as described below.
Dissolution and permeation for the defined-size aerosol particles of inhaled corticosteroids (ICSs) in the Transwell® system. The PVDF filter membrane with the deposited ICS was placed, with the deposited drug face down, onto the donor compartment. Then, 0.04 ml of aqueous fluid was added over the filter membrane to initiate ICS dissolution and permeation into the 1.4 ml receptor fluid, while the entire system was maintained at 37°C and near 100% relative humidity inside the incubator.
Transwell® Membrane Permeation Profile of ICS Following Solution Application
To determine the profile of ICS’s diffusive permeation across the Transwell® supporting membranes, unhindered by dissolution, 0.04 ml of 60 μg/ml flunisolide (FN, Sigma-Aldrich, St. Louis, MO) solution in PBS was directly applied onto the PVDF filter membrane (without drug deposits) placed on the donor compartment of the Transwell® insert. An aliquot (0.5 ml) of sample was then withdrawn at different times for 5 h, while the Transwell® system was placed inside the incubator. The samples were analyzed by the methods shown in Table II, enabling the profile determination of FN permeation across the Transwell® membranes, unhindered by dissolution.
Profile and Data Analysis
Cumulative ICS mass dissolved and permeated into the receptor compartment at a given sampling time was calculated from the product of the sample concentration and the receptor compartment volume (1.4 ml) plus that of the concentrations in previously taken samples and their volumes (0.5 ml). Initial ICS mass deposited on each of the PVDF filter membranes was determined from the total ICS masses recovered from the donor and receptor compartments by the end of each experiment. Accordingly, the dissolution and permeation profile of each Transwell® experiment was described as % of ICS mass dissolved and permeated into the receptor compartment over initial ICS mass deposited as a function of time. The profiles were then collectively expressed as mean % profiles with standard deviation (SD) from triplicate experiments. When applicable, the apparent half-life of the profile was determined, assuming the first-order kinetics reaching asymptotes. The statistical profile comparison employed two-way analysis of variance (ANOVA), where p < 0.05 was considered to be significant.
Cumulative % mass of flunisolide (FN) appeared into the receptor compartment as a function of time (a) following solution application at 2.4 μg and aerosol deposition at 2.3 μg and (b) following aerosol deposition at various 2.3–18.8 μg; these aerosols were generated from AEROBID® pMDI and collected in the 2.1–3.3 μm aerodynamic diameters. Data represent mean ± SD (n = 3). The profiles shown in (a) were statistically superimposed (p > 0.05; ANOVA).
Cumulative % mass of (a) triamcinolone acetonide (TA), (b) budesonide (BD) and (c) fluticasone propionate (FP) dissolved and permeated into the receptor compartment as a function of time following 2.1–3.3 μm aerosol particle deposition at different masses from AZMACORT® pMDI, PULMICORT® TURBUHALER® DPI and FLOVENT® HFA pMDI, respectively. Data represent mean ± SD (n = 3).
Cumulative % mass of various inhaled corticosteroids (ICSs) dissolved and permeated into the receptor compartment as a function of time following 2.1–3.3 μm aerosol particle deposition at comparable 2.3–2.9 μg from respective inhaler products. Data represent mean ± SD (n = 3). Key: (○) flunisolide (FN) at 2.3 ± 0.1 μg from AEROBID® pMDI, (▲) triamcinolone acetonide (TA) at 2.5 ± 0.3 μg from AZMACORT® pMDI, (□) budesonide (BD) at 2.9 ± 0.2 μg from PULMICORT® TURBUHALER® DPI and (▼) fluticasone propionate (FP) at 2.4 ± 0.5 μg from FLOVENT® HFA pMDI.
Cumulative % mass of (a) fluticasone propionate (FP) and (b) beclomethasone dipropionate (BDP) dissolved and permeated into the receptor compartment as a function of time following 2.1–3.3 μm aerosol deposition at the identical 2.4 and 1.6 μg, respectively, from different inhaler products. Data represent mean ± SD (n = 3). As the profiles shown in (a) were statistically indistinguishable (p > 0.05; ANOVA), a single linear regression line is drawn. In contrast, each of the profiles shown in (b) appeared to be composed of two linear phases separated at 1.5 h, as drawn with the linear regression lines.
RESULTS
ICS Aerosol Particle Collection on Filter Membranes by Impaction
Table III shows the ICS masses deposited and collected on each PVDF filter membrane placed on the Stage 4 of the ACI following various actuations or doses from the pMDIs or DPIs tested in this study, respectively (Table I). The choice of the number of actuations or doses per experiment was made so that the ICS masses deposited on the filter membranes were compared optimally at the equivalent mass range of 2–3 μg or varied within or across the different ICSs and inhaler products. This was to assess the kinetics of dissolution and permeation, in relation to the specific ICS in question, aqueous solubility, deposited mass, formulation and dosage form, and/or aerosol generation, as studied below. Clearly, the required numbers of actuations or doses to yield the comparable 2–3 μg mass deposition differed between the ICSs and products, due to the differences in the metered dose (Table I) and aerodynamic particle size distribution. Overall, however, the ACI deposition system shown in Fig. 1 enabled 0.4–19.8 μg of the 2.1–3.3 μm ICS aerosol particle collection on the PVDF membranes in a fairly reproducible fashion (≤21% of relative standard deviation).
Dissolution and Permeation Profiles of ICS Aerosol Particles
Effects of Aqueous Solubility and Deposited Mass on the Apparent Profiles
Fig. 3-a shows the cumulative % mass profile of FN appeared into the receptor compartment over 5 h upon aerosol deposition at 2.3 ± 0.1 μg on the PVDF membranes placed on the Stage 4 of the ACI (in the 2.1–3.3 μm aerodynamic diameter), generated from AEROBID® pMDI, compared to that for FN applied as 0.04 ml of 60 μg/ml solution, i.e., 2.4 μg of FN mass. Both profiles were kinetically apparent first-order, reaching almost complete permeation by 5 h (95.0 ± 1.1 and 97.3 ± 0.3%, respectively) with the half-lives of 0.6–0.7 h. Notably, the profiles were statistically indistinguishable (p > 0.05), although the aerosol deposition, in theory, required FN dissolution in the 0.04 ml buffer fluid on the donor compartment prior to membrane permeation. This, in turn, suggested that such FN aerosol particle dissolution was rather spontaneous, complete and thus kinetically insignificant on the donor compartment at this deposited mass. In fact, in calculation, the 0.04 ml buffer fluid on the donor compartment could afford dissolving this 2.3 μg of the FN aerosol particles, by virtue of 140 μg/ml of high aqueous solubility (Table I). However, when the aerosol masses were increased to 10.9 ± 0.5 and 18.8±1.1 μg, the kinetics of FN appearance to the receptor compartment became progressively slower, as shown in Fig. 3-b. This was likely caused by the aqueous fluid-capacity-limited dissolution of the FN aerosol particles prior to the membrane permeation, as the 0.04 ml buffer fluid could no longer dissolve the entire deposited drug particles, thereby leaving the undissolved drug particles on the donor side. Even so, the profiles at these higher aerosol masses still reached near complete permeation by 5 h, presumably since dissolution of such undissolved FN particles efficiently occurred on the donor side, subsequent to the membrane permeation of the dissolved FN into the receptor. Hence, the profiles at these higher aerosol masses indeed involved the kinetics of dissolution, represented by the slower kinetics and longer half-lives, compared to those for FN applied as solution or deposited at the lower mass (Fig. 3-a).
Fig. 4 shows the % profiles of dissolution and permeation for the TA, BD and FP aerosol particles collected also on the Stage 4 of the ACI in the 2.1–3.3 μm aerodynamic diameters at various deposited masses per filter membrane from their respective inhaler products. Given the limited aqueous solubility of each of these ICSs (Table I), the 0.04 ml aqueous fluid on the donor compartment did not completely dissolve the deposited aerosol drugs in most cases, like the FN aerosols at 10.9 and 18.8 μg (Fig. 3-b). As a result, the rates of dissolution and permeation decreased with increasing the deposited masses for each of the ICSs, as also seen in Fig. 3-b. Again, this was attributed to increased undissolved ICSs left on the donor membranes with increasing aerosol mass deposition, which exceeded the dissolution capacities. Hence, at the identical ICS mass deposition, the lower the ICS aqueous solubility was, the greater the undissolved ICS mass remained on the donor side and therefore, the slower the rate of dissolution and permeation became. Indeed, when the profiles were assessed at the best comparable 2.3–2.9 μg aerosol deposition including the FN aerosols, the rank-order of the rate and extent of dissolution and permeation conformed to that of the ICS’s aqueous solubility, as shown in Fig. 5, where the % ICS masses dissolved and permeated into the receptor compartment were strikingly varied, ranging from 1.9% to 95.0% at 5 h. This was surely due to the maximum 1,000-fold difference in the aqueous solubility across these ICSs, provided that their membrane diffusivities were effectively consistent by virtue of similar molecular weights and thus, diffusion coefficients.
Effects of Formulation and Inhaler on the Apparent Profiles
Fig. 6 shows the % profiles of dissolution and permeation for the FP and BDP aerosol particles in the 2.1–3.3 μm aerodynamic diameters, generated from different inhaler products, yet collected at an identical mass of 2.4 and 1.6 μg, respectively. For FP, the profiles were linear, reaching only ≤1.9% by 5 h (Fig. 6-a) and thus exhibiting the pseudo-zero-order kinetics due to its extremely low aqueous solubility (0.14 μg/ml; Table I). Nevertheless, these profiles were statistically superimposed (p > 0.05), which suggested that the FP aerosol particles generated from suspension in the HFA-134a propellant (pMDI) were identical to those from the DPI (crystalline FP) in dissolution. In contrast, as shown in Fig. 6-b, the profiles for the BDP aerosol particles both appeared to be biphasic, as their linear rates slowed down after 1.5 h. Moreover, the QVAR® pMDI exhibited significantly faster and greater dissolution and permeation than the VANCERIL® pMDI during 10 h (p < 0.05), apparently caused by the faster kinetics in the first 1.5 h for QVAR® than VANCERIL®. Indeed, their linear rates were 2.6 ± 0.3 and 1.5 ± 0.2%/h, respectively, while the profiles at ≥1.5 h were shown to be parallel, with their comparable rates at 1.1 ± 0.08 and 1.1 ± 0.03%/h, respectively. Notably, however, any of these rates for the BDP aerosol particles were much faster than the rate for the FP aerosol particles shown in Fig. 6-a, i.e., 0.4 ± 0.1%/h, despite the identical aqueous solubility (at 0.13 μg/ml for BDP and 0.14 μg/ml for FP; Table I). As a result, the BDP aerosol particles from these two different pMDIs enabled much higher 11.1 ± 0.7 and 6.2 ± 0.5% of dissolution and permeation at 5 h than the FP aerosol particles from their inhaler products (∼1.9%; Fig. 6). As FP formulated in, and generated from, DISKUS® DPI was indeed crystalline with the 0.14 μg/ml aqueous solubility, neither of the BDP profiles likely resulted from the crystalline BDP with the 0.13 μg/ml aqueous solubility.
Effects of Particle Size on the Apparent Profiles
Fig. 7 shows the % profiles of dissolution and permeation for the BD aerosol particles collected in the different aerodynamic diameter ranges of 2.1–3.3 and 4.7–5.8 μm on the Stages 4 and 2 of the ACI at the comparable 1.7 ± 0.1 and 1.9 ± 0.1 μg, respectively, generated from PULMICORT® TURBUHALER® DPI. The smaller 2.1–3.3 µm BD aerosol particles exhibited faster and greater dissolution and permeation than the larger 4.7–5.8 µm BD aerosol particles, represented by the half-lives of 1.8 ± 0.1 and 3.9 ± 0.9 h, respectively. This was presumably attributed to the 1.4–2.8 times greater surface area for the smaller-size aerosol particles, which, in theory, should proportionally accelerate their dissolution rates. Indeed, this ratio of the surface area (1.4–2.8) was consistent with 1.6–2.8 estimated as a ratio of their dissolution rates (via a reciprocal of the half-lives).
DISCUSSION
Aerosol drug particles generated from inhaler products may alter their dissolution kinetically (e.g., rate, extent and/or apparent order) from the bulk drug materials due to different crystallinity, wettability and/or solubility by polymorphism, amorphism and/or formulation excipients, as a result of aerosol generation (4–8,14). Such dissolution kinetics could be also dependent upon the aerosol particle size and distribution, as the surface area per unit weight is greater for the smaller-size aerosol particles. Moreover, the lining fluid volume in the lung is quite limited (10–30 ml in humans), such that, in certain cases, this could limit the maximum aerosol drug mass for dissolution, thereby leaving undissolved drugs on the airways. Such scenarios have been speculated since the advent of ICSs with extremely low aqueous solubility, such as fluticasone, mometasone and ciclesonide (4,6,8). In fact, one of these ICSs, FP, was reported to exhibit quite long (4–5 h) mean absorption times from its pharmacokinetics in humans following inhalation, compared to other readily soluble ICSs, e.g., BD and TA (<1 h; 4,6,15). Even so, it still remains inconclusive whether these quite long mean absorption times were indeed caused by incomplete aerosol particle dissolution on the airways, as these pharmacokinetic values were also highly affected and confounded by differences in the lung-regional deposition (6,7). Accordingly, it was of great interest to identify that aerosol drug particles generated from inhaler products dissolved differently from bulk dissolution of the raw materials with known solubility, due to the limited dissolution fluid capacity and aerosol particle mass, size, formulation and/or generation.
The system developed in this study (Figs. 1 and 2) was unique with respect to the ICS aerosol particle collection in the defined-size and respirable 2.1–3.3 or 4.7–5.8 μm range, directly generated from commercial inhaler products. Aerosol drug particle dissolution occurred only into the limited 0.04 ml of the stationary aqueous fluid (PBS or DDW) applied on the donor compartment of the 4.9 cm2 Transwell®, after which only dissolved ICS was permeated into the receptor compartment across the supporting membrane. This limited 0.04 ml volume of the dissolution capacity was well maintained hydrostatically with 1.4 ml of the receptor compartment fluid under the sink conditions, as the receptor concentrations never exceeded 20% of the aqueous solubility of each ICS (data not shown). However, in simple calculation, addition of this 0.04 ml aqueous fluid should have resulted in 81.6 μm of the fluid thickness per unit area for aerosol dissolution on the donor compartments. In this sense, as the real lung lining fluid thickness, e.g., in the upper tracheobronchial regions, has been reported to be 10–23 μm or less (16,17), this system was rather in favor of the fluid capacity per unit area for dissolution of deposited drug aerosols, compared to the lung, by a factor of 3.5–8.2. Finally, the entire system was placed inside the incubator maintained at 37°C and near 100% relative humidity, like the airways. All of these were an in vitro attempt to represent aerosol drug particle dissolution into the limited volume of the lining fluid within the lung following deposition.
While the profiles of dissolution and permeation differed considerably between the ICSs, their rank-order was overall shown to conform to that of the aqueous solubility (Fig. 5). However, the kinetics appeared to be further hampered for the ICSs with the low solubility, since the limited 0.04 ml PBS or DDW, the stationary dissolution capacity for aerosol ICS dissolution, appeared to leave undissolved drug particles on the donor compartment. As a result, the profiles were also shown to depend on the ICS aerosol masses, resulting in the lower % dissolution and permeation with increasing aerosol mass (Figs. 3-b and 4). Consistent with this observation, in a satellite experiment, 0.04 ml addition of 100% ethanol enabled much faster and greater % profile for the BD aerosol particles than that of PBS shown in Fig. 4-b, by virtue of the higher BD solubility in ethanol (data not shown). Meanwhile, the profiles were also shown to be altered by formulation and/or aerosol generation. In Fig. 6, the BDP aerosol particles exhibited significantly faster and greater dissolution than the FP aerosol particles, despite the identical aqueous solubility. Moreover, the generations from two different pMDI products (VANCERIL® and QVAR®) further resulted in the different profiles. Finally, the profiles appeared to be also aerosol particle size-dependent, yielding the faster and greater kinetics for the smaller-size aerosols, at least for BD (Fig. 7). Quite recently, Son and McConville (18) also reported aerosol particle size-dependent dissolution kinetics for hydrocortisone collected from the bench-scale formulation in the membrane cassette dissolution system. Taken all together, it was successful in this study to demonstrate that aerosol drug (ICS) particle dissolution into the limited aqueous fluid volume could be kinetically different, depending on not only the ICS solubility but also the aerosol mass, size, formulation and/or generation.
The FP aerosols generated from the pMDI and DPI exhibited indistinguishable profiles of dissolution and permeation following equivalent mass deposition (Fig. 6-a). This suggested that both aerosol drug particles were identical in the dissolution kinetics, as the FP’s crystalline states were maintained upon aerosol generation from suspension in the HFA-134a propellant (pMDI) and powder admixture with lactose (DPI). Indeed, this was consistent with the pharmacokinetic evidence of no real difference between these pMDI and DPI inhalation in humans (19), even though the FP aerosol particle dissolution in the lung was likely rate-limited. In contrast, despite the identical aqueous solubility of the raw drug materials, the BDP aerosol particles exhibited significantly faster and greater dissolution and permeation than the FP aerosol particles (Fig. 6). Moreover, the profiles were biphasic, decelerating their rates at ≥ 1.5 h, while differing between the VANCERIL® and QVAR® pMDIs (Fig. 6-b). In this context, BDP has been shown to form solvate crystals (clathrates) in the presence of CFC and HFA propellants (20), which should have different solubility values from BDP itself. Furthermore, it has been recently reported that its aerosol particles from solution in the HFA propellant, like QVAR®, were initially round and highly porous in shape, dissolving faster in the human bronchial fluid than those from suspension in the CFC propellant (7). Thus, these could explain the BDP’s biphasic profiles in this Transwell® dissolution, caused by the initial formation of at least partially amorphous BDP aerosol particles from these pMDIs, likely followed by the formation of BDP-solvate crystals (clathrates). Even so, it appeared unlikely that this difference of the dissolution kinetics for the BDP aerosol particles from the suspension in the CFC propellant and solution in the HFA propellant caused the difference in their clinical performance. Both dosage forms have been ensured to be clinically bioequivalent, as a result of reduction in the BDP dose for QVAR® by virtue of its smaller aerosol size generation enabling clinically preferable lung-regional deposition (21). In fact, this dose reduction in the clinical study may have resolved the difference in the dissolution kinetics of the BDP aerosols between these two products.
Currently, the USP standards for product performance of orally inhaled dosage forms only concern the testing of delivered dose and aerodynamic particle size distribution (13), and do not include that of dissolution. This is primarily because drug delivery to, and regional deposition within, the lung are believed to far outweigh aerosol drug particle dissolution in controlling the effective dose and its therapeutic effect (22), as seems to be the case even for BDP as discussed above. In fact, among the currently approved products, it was reported that there appears to be no compelling evidence that dissolution of inhaled therapeutics in the lung hampers their pharmacokinetics and/or clinical performance following inhalation in humans (22). Clearly, however, the system developed in this study was not designed to predict such rate-determined dissolution for inhaled aerosol drugs or to test pharmaceutical equivalence or difference in dissolution within or between inhaler products. This was the primary reason that PBS or DDW was employed as its dissolution medium, despite substantial difference in composition from the real lung lining fluid, such as proteins, surfactants and ionic species (23). It was, rather, aimed to kinetically characterize the dissolution of the defined-size and respirable aerosol drug particles, as related to changes in solubility, surface area and structure, particle shape and/or crystal habit, independent from particle size or lung-regional deposition effects. Hence, it would also be useful to assess the benefits of engineered aerosol particles on dissolution (or release), such as liposomes, micelles, microspheres or nanoparticles for inhalation.
CONCLUSIONS
This study developed a unique in vitro aqueous fluid-capacity-limited dissolution system for respirable aerosol drug (ICS) particles generated from commercial inhaler products. Aerosol particles of 5 inhaled corticosteroids (ICSs) from 7 inhaler products were tested by kinetically monitoring their dissolution into the limited 0.04 ml aqueous fluid on the donor compartment of the Transwell® and permeation into its receptor compartment at 37°C and near 100% humidity. The % profiles of dissolution and permeation were apparent first-order or pseudo-zero-order kinetically, reaching varying 1.9–95.0% by 5 h, while their rank-order was overall in line with that of the ICS aqueous solubility (Fig. 5). With increasing aerosol particle mass, however, the profiles were decelerated, attributed to undissolved ICS aerosol particles left by the limited dissolution fluid capacity on the donor compartment (Figs. 3-b and 4). Among them, the FP aerosols from FLOVENT® HFA and DISCUS® exhibited indistinguishable dissolution profiles, as the FP’s crystalline states could be maintained upon aerosol generation from suspension in the HFA-134a propellant (pMDI) and powder admixture with lactose (DPI), respectively. In contrast, the BDP aerosols from QVAR® were shown to dissolve significantly faster and greater than those from VANCERIL®, presumably by virtue of BDP’s amorphism and solvate crystal (clathrate) formation. As predicted from the theory, the 2.1–3.3 μm aerosol particles dissolved faster than the 4.7–5.8 μm aerosol particles. Through this unique dissolution system, the ICS aerosol particle dissolution in the limited volume of aqueous fluid was shown to differ kinetically due to not only ICS solubility but also aerosol mass, size, formulation and/or generation.
Abbreviations
- ACI:
-
Andersen non-viable Cascade Impactor
- ANOVA:
-
analysis of variance
- APCI:
-
atmospheric pressure chemical ionization
- API:
-
active pharmaceutical ingredient
- BD:
-
budesonide
- BDP:
-
beclomethasone dipropionate
- CFC:
-
chlorofluorocarbon
- DDW:
-
distilled deionized water
- DPI:
-
dry powder inhaler
- ESI:
-
electrospray ionization
- FN:
-
flunisolide
- FP:
-
fluticasone propionate
- HFA:
-
hydrofluoroalkane
- HPLC:
-
high performance liquid chromatography
- ICS:
-
inhaled corticosteroid
- LOQ:
-
limit of quantification
- MS:
-
mass spectrometry
- PBS:
-
phosphate-buffered saline
- pMDI:
-
pressurized metered dose inhaler
- PVDF:
-
polyvinylidene difluoride
- SD:
-
standard deviation
- TA:
-
triamcinolone acetonide
- US:
-
United States
- USP:
-
United States Pharmacopeia
- UV:
-
ultraviolet
- VCU:
-
Virginia Commonwealth University
References
Dressman JB, Amidon GL, Reppas C, Shah VP. Dissolution testing as a prognostic tool for oral drug absorption: immediate release dosage forms. Pharm Res. 1998;15:11–22.
General Chapter <711> Dissolution, United States Pharmacopeia and National Formulary, USP 32-NF 27, United States Pharmacopeial Convention, Maryland, 2009, pp. 263–271.
Azarmi S, Roa W, Löbenberg RA. Current perspectives in dissolution testing of conventional and novel dosage forms. Int J Pharm. 2007;328:12–21.
Edsbäcker S, Johansson CJ. Airway selectivity: an update of pharmacokinetic factors affecting local and systemic disposition of inhaled steroids. Basic Clin Pharmacol Toxicol. 2006;98:523–36.
Jashnani RN, Dalby RN, Byron PR. Preparation, characterization, and dissolution kinetics of two novel albuterol salts. J Pharm Sci. 1993;82:613–6.
Hochhaus G, Derendorf H, Moellmann H, Talton J. Factors involved in the pulmonary targeting of inhaled drugs. In: Shleimer RP, O’Byrne PM, Szefler SJ, Brattsand R, editors. Inhaled steroids in asthma: Optimizing effects in the airways. New York: Marcel Dekker; 2002. p. 283–307.
Freiwald M, Valotis A, Kirschbaum A, McClellan M, Mürdter T, Fritz P, et al. Monitoring the initial pulmonary absorption of two different beclomethasone dipropionate aerosols employing a human lung reperfusion model. Respir Res. 2005;6:21.
Edsbäcker S, Wollmer P, Selroos O, Borgström L, Olsson B, Ingelf J. Do airway clearance mechanisms influence the local and systemic effects of inhaled corticosteroids? Pulm Pharmacol Ther. 2008;21:247–58.
Davies NM, Feddah MR. A novel method for assessing dissolution of aerosol inhaler products. Int J Pharm. 2003;255:175–87.
Baumann D, Bachert C, Högger P. Dissolution in nasal fluid, retention and anti-inflammatory activity of fluticasone furoate in human nasal tissue ex vivo. Clin Exp Allergy. 2009;39:1540–50.
Krishnaswami S, Möllmann H, Derendorf H, Hochhaus G. A sensitive LC-MS/MS method for the quaitification of fluticasone propionate in human plasma. J Pharm Biomed Anal. 2000;22:123–9.
Wang Y, Hochhaus G. Simultaneous quantification of beclomethasone dipropionate and its metabolite, beclomethasone 17-monopropionate in rat and human plasma and different rat tissues by liquid chromatography-positive electrospray ionization tandem mass spectrometry. J Chromatogr B Analyt Technol Biomed Life Sci. 2004;805:203–10.
General Chapter <601> Aerosols, metered-dose inhalers, and dry powder inhalers, United States Pharmacopeia and National Formulary, USP 32-NF 27, United States Pharmacopeial Convention, Maryland, 2009, pp. 204–224.
Ward GH, Schult RK. Process-induced crystallinity changes in albuterol sulfate and its effect on powder physical stability. Pharm Res. 1995;12:773–9.
Thorsson L, Edsbacker S, Kallen A, Lofdahl CG. Pharmacokinetics and systemic activity of fluticasone via Diskus and pMDI, and of budesonide via Turbuhaler. Br J Clin Pharmacol. 2001;52:529–38.
Widdicombe JH, Bastacky SJ, Wu DX-Y, Lee CY. Regulation of depth and composition of airway surface liquid. Eur Repir J. 1997;10:2892–7.
Song Y, Namkung W, Nielson DW, Lee JW, Finkbeiner WE, Verkman AS. Airway surface liquid depth measured in ex vivo fragments of pig and human trachea: dependence on Na+ and Cl− channel function. Am J Physiol Lung Cell Mol Physiol. 2009 Oct 9. [Epub ahead of print] doi:10.1152/ajplung.00085.2009.
Son Y-J, McConville JT. Development of a standardized dissolution testing method for inhaled pharmaceutical formulations. Int J Pharm. 2009;382:15–22.
Brindley C, Falcoz C, Mackie AE, Bye A. Absorption kinetics after inhalation of fluticasone propionate via the Diskhaler, Diskus, and metered-dose inhaler in healthy volunteers. Clin Pharmacokin. 2000;39:1–8.
Dalby RN, Byron PR. Formulations for delivery of beclomethasone diproprionate by metered dose inhalers containing no chlorofluorocarbon propellants. United States Patent 5202110 (1993).
Leach CL, Davidson PJ, Boudreau RJ. Improved airway targeting with the CFC-free HFA-beclomethasone metered-dose inhaler compared with CFC-beclomethasone. Eur Respir J. 1998;12:1346–53.
Gray VA, Hickey AJ, Balmer P, Davies NM, Dunbar C, Foster TS, et al. The inhalation ad hoc advisory panel for the USP performance tests of inhalation dosage forms. Pharmacopeial Forum. 2008;34:1068–74.
Moss OR. Simulants of lung interstitial fluid. Health Phys. 1979;36:336–40.
Acknowledgments
The authors are grateful to H. Thomas Karnes, Ph.D. (VCU School of Pharmacy, VCU-SOP) for allowing the use of HPLC-MS/MS for FP and BDP assays. This research was funded by the Medical College of Virginia Foundation and VCU-SOP. D.A. acknowledges financial supports from the International Foundation of Ethical Research and VCU-SOP during her graduate study.
Author information
Authors and Affiliations
Corresponding author
Rights and permissions
About this article
Cite this article
Arora, D., Shah, K.A., Halquist, M.S. et al. In Vitro Aqueous Fluid-Capacity-Limited Dissolution Testing of Respirable Aerosol Drug Particles Generated from Inhaler Products. Pharm Res 27, 786–795 (2010). https://doi.org/10.1007/s11095-010-0070-5
Received:
Accepted:
Published:
Issue Date:
DOI: https://doi.org/10.1007/s11095-010-0070-5