Abstract
In this paper, the effects of atmospheric turbulence on the performance of a hybrid dense wavelength division modulation (DWDM) of free space optical (FSO) communication using advanced modulations are presented. Enhancement in the capacity of transmission and bit rates of the optical networks is obtained by DWDM technology based on FSO communication systems. Proposed modulations are included Return-to-Zero Differential Phase Shift Keying (RZ-DPSK) and Non-Return-to-Zero Differential Phase Shift Keying (NRZ-DPSK) that each of them increases spectrum efficiency. Furthermore, the optical power budget of the proposed system is analytically calculated. Variation of Bit Error Rate and Max quality factor of the designed system with different path distance and input power are obtained considering outdoor atmosphere channel model. As a result, the RZ-DPSK modulation has better performance in comparison to NRZ-DPSK modulation when the atmospheric condition is considered.
Similar content being viewed by others
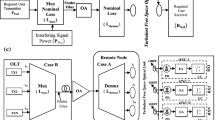
Avoid common mistakes on your manuscript.
1 Introduction
In optical networks, Dense Wavelength Division Multiplexing (DWDM) systems have different applications in modern communication networks. The DWDM technology increases the bandwidth and capacity of the communication system at the same time by using several wavelength that have been transmitted on a Single Mode Fiber (SMF) without other optical fiber. Bit rate and channel spacing of DWDM systems are considered to increase the capacity and bandwidth of the optical communication systems (Kartalopoulos 1999; Marciniak 2007; Winzer and Essiambre 2006; Ali et al. Feb. 2015). Free space optical communication (FSO) can be defined as a type of optical transmission technology which transmitted signal and data through air, water or vacuum. Development of FSO technology has several advantages such as high security, simple design, high speed and low interference. FSO technology for this features is taken into consideration. Also, this technology can be used in several application such as inter-satellite, deep space link, last mile accesses networks and backup links. Therefore, investigation the DWDM technology with FSO communication can be increased the speed of data transmission and bandwidth in high bit rates and long distance links (Killinger Oct. 2002; Hranilovic 2005; Ciaramella et al. Dec. 2009; Li et al. 2016). In addition to the bandwidth and capacity of the networks, spectrum efficiency of transmission is one of the significant parameters in design of lightwave systems. To minimize linear and nonlinear effects over transmission, an advanced optical modulation formats are needed. A modulation format with narrow optical spectrum has capable to increase the spectrum efficiency of link. On–Off Keying (OOK) modulation is not suitable for next generation of optical networks. Intensity modulations such as Differential Phase Shift Keying (DPSK) and Quarter Phase Shift Keying (QPSK) have suitable performance for higher data rates and spectrum efficiency of DWDM systems (Kahn and Ho Jun. 2004; Haris 2008; Chen 2020; Wang and Chen 2020). These modulations formats have inherent − 3 dB better receiver sensitivity by using balanced detection (Xu 2019; Zhao 2019; Li 2018; Wang 2017). Also, atmospheric condition is fundamental problem in propagation of light at free space. So, several optimization agolrithms are proposed (Xia 2017; Shen 2016; Chen 2016; Hu 2015; Xu and H.-l.J.S.C. Chen, 2014). For example Zhang groups proposed learning algorithm (Zhang 2020; Zhang, et al. 2020a). In addition colony optimization (Zhao, et al. 2020a) and whale optimization (Tu 2021) and so are applied by research groups (Shan, et al. 2020; Yu 2021, 2020; Hu et al. 2020; Zhao 2014). Environmental effects such as pressure and atmosphere turbulence can bring variable refractive index during transmission of data. Variable refractive index makes spatial and temporal changes of beam intensity in receiver (Garg Jul. 2013). In the other words, the performance of FSO systems are affected by atmospheric conditions (Kumar and Singh 2014; Failed 2020).
In (Tripathi et al. 2020; Sharma et al. 2021), two DWDM-FSO system with considering atmospheric turbulence have been presented. the proposed system (Tripathi et al. 2020) investigated based on FSO, passive optical network (PON) and radio over free space based on DWDM configuration. By using suitable modulation, the system can transmit 12 wirless channels at 2.5 Gb/s data rate. Despite of good performance of the presented system, but the power budget of the system has not be calculated.
A FSO/fiber communication system for enhancement the operation of the proposed system based on OOK modulation formats has been designed (Elsayed and Yousif 2020). The presented communication system has good performance in the weak turbulence condition, but, in addition to the small distance, the power budget of the system has not be considerd.
Several system have been proposed for enhancement of the DWDM-FSO configuration under turbulence condition with differen modulation schems (Elsayed and Yousif 2020; Elsayed 2021a, 2021b).
In this paper a DWDM-FSO system for two different modulation schemes are presented. This paper not only investigate the proposed system under Outdoor atmospheric channel model, but also can calculate the optical power budget off the system. The novelty of the presented DWDM-FSO system is that by considering two advanced on–off keying modulation formats under modelling of free space channel, we can calculate the power budget of system analytically and optimization the parameters of the system under this issue.
The paper is organized as follows: In Sect. 2 and 3, description of modulation technique and outdoor atmospheric channel model with optical power budget are presented. The proposed system is expressed in Sect. 4. Finally simulation results and conclusion are presented in Sect. 5 and Sect. 6, respectively.
2 Description of modulation techniques
Digital modulation can be exposed by the phase of the optical carrier and referred to optical Phase Shift Keying (PSK). Figure 1a shows the configuration of Non Return to Zero Differential Phase Shift Keying (NRZ-DPSK) in lightwave systems that consist of two parts consisiting of transmitter and receiver which these parts used in transmitter and receiver of the proposed system, recpectively. In the NRZ-DPSK transmitter, is a precoder modulation which has one-bit delay composes the NRZ signal. This signal which known as DPSK electrical signal is driven to a Mach-Zehender Modulator (MZM).
The output of MZM is an NRZ-DPSK optical signal. After FSO channel and demultiplxing, we need o demodulation of inpout signals that modulated. At receiver, the signal can be detected in two ways such as direct and balanced detection. The receiver consisiting of several devices as two couplers, phas shift, time delay, two photodetector and band pass filter that coused to demodulated the oupout signal of the FSO link. This method can optimization of the signal that multiplexing in input. The The main advantage of DPSK modulation instead of OOK is − 3 dB receiver sensitivity improvement. The optical spectrum and generated bits of NRZ-DPSK modulation after MZM at 193.1 THz frequency are shown in Fig. 1b and c, respectively (Haris 2008; Elsayed 2021c; Liu and Kao 2009). It is obvious that the output power of NRZ-DPSK modulation formats is near − 10 dB.
To overtake long distance and high capacity of transmission link, Return to Zero Differential Phase Shift Keying (RZ-DPSK) modulation is proposed. Figure 2a shows the configuration of RZ-DPSK modulation. This modulation unlike the NRZ-DPSK, usually needs two MZM, one for pulse carving and the other for phase modulation. After generation of DPSK electrical signal similar to NRZ-DPSK modulation, the signal is driven to the second MZM as known as a phase modulator. The balanced detection is used in RZ-DPSK as same as NRZ-DPSK. The optical spectrum and generated bits of RZ-DPSK modulation after second MZM at 193.1 THz frequency are shown in Fig. 2b and c, respectively (Haris 2008; Elsayed 2021c; Liu and Kao 2009). As we can see from Fig. 2b, the output power of RZ-DPSK modulation is − 10 dB less than NRZ-DPSK modulation formats that affected the final DWDM-FSO system.
3 Outdoor atmospheric channel model and optical power budget
In FSO technology, the atmospheric channel is a dynamic and wrapped environmental that affected the propagation of laser optical beam. Optical signals which propagating through atmosphere channel are tender to the atmosphere condition such as fog, rain and haze. During transmission of optical beams through FSO channel, the interaction between the photons and atmosphere molecules caused scattering and absorption of optical photons. For minimized the effect of beam scattering, a laser with very narrow band spectrum can be used. To reduce the atmospheric absorption losses, selecting a wavelength that stay on the optimal transmission windows such as visible and infrared bands is needed. For an FSO link, the receiver optical power Pr is related to optical power Pt, which defines as Eq. (1).
where τod is the optical depth. Partial of transmitted power in optical communication defines as beam transmitted power. The optical depth and atmospheric transmittance are related to the atmospheric attenuation coefficient and the transmission path length Lp that explains as Eq. (2) (Cai et al. 2005; Ghassemlooy et al. 2013; Maral and Bousquet 2009).
where γt is total attenuation in m−1 and Lp is transmission path link in m. The attenuation of optical beams in atmosphere channel that generated by scattering and absorption, introduced by gases and aerosols. Also, the atmospheric attenuation coefficient can define as Eq. (3) (Gagliardi and Karp 1995).
where αm1 is molecular absorption coefficient, αa1 is Aerosol absorption coefficient, βm1 is molecular scattering coefficient and βa1 is total scattering coefficient. Due to the experimental amount of αm1, αa1 and βm1 which are insignificant, we can approximate attenuation with Eq. (4) (Willebrand and Ghuman 2002).
where V is visibility in km, λ is a wavelength in nm and q is dispersion distribution quantity. The value of q follows as Eq. (5) (Kruse et al. 1962).
The visibility and attenuation at different weather condition for different wavelengths are shown in Table 1.
To achieve high security, narrowband optical beams are used in FSO links and this is one of the advantages of them. But due to diffraction, the optical beams spread out and leads to beam divergence loss. In a system with low bit rates, the value of divergence is 68 mrad but in systems with high bit rates, the beam divergence is less than 2 mrad. The signal propagation loss can be expressed as Eq. (6) (Gowar 1993).
where λ is wavelength in nm. The performance of FSO link is degraded when the optical beam interaction with atmosphere turbulence causes the scintillation of optical beam. Atmosphere turbulence is an irregular random motion which caused change in refractive index of atmosphere channel. We used the Hufnagel-Valley (HV) method to model the refractive index structure of atmospheric channel. This method that is widely used in optical communication systems is described as Eq. (7) (Wang et al. Jun 2013).
where A is min value of Cn (0) at the ground in m−2/3, and v is rms of wind speed in m/s, that for our proposed system is almost 3.4 × 10–25. For optical communication systems, the power budget can be calculated as the Eq. (8).
The received power includes the splitter, splice loss, connector loss, attenuation loss and FSO loss. The free space loss is given by Eq. (9) (Couch 2013).
Should bear in mind that, number of a splice in (L) km is L-1. Thus, the splice loss is 1 × 0.2 dB = 0.2 dB. Table 2 shows the typical link budget for 10 Gbps bit rate and 2 km path link.
4 Proposed system
Figure 3 shows the configuration of our proposed FSO-DWDM system with 16 channels by 2 km path link. The proposed system is simulated at 10 Gbps bit rate with two modulation formats consist of RZ-DPSK and NRZ-DPSK. This system is similar to the other communication systems, consist of transmitter block, FSO channel and receiver block.
Transmitter block is consist of three main parts such as 1: a transmitter that used 16 channels with 200 GHz frequency spacing and 10 dB input power in 193.1 THz center frequency, 2: subsystem 1 until subsystem 16 that consists of RZ-DPSK and NRZ-DPSK modulations and 3: an optical Ideal Mux. In transmission link, we modeled communication channel by FSO channel with 2 km path link. Receiver block is consist of 1: an optical Ideal Demux and 2: receivers 1 up to 16 use balanced detector for detection of the signals. We analyzed the performance of output results by BER analyzer. The simulation parameters of the proposed system and FSO channel are presented in Table 3.
5 Simulation results
The proposed system in the clear weather condition was simulated. In Fig. 4a and b, the eye diagram of NRZ with direct detection and NRZ-DPSK with balanced detection for proposed system are shown. The system is simulated at 10 Gbps and 2 km path link. The maximum quality factor Q = 2.13 and 22.25 is obtained for NRZ and NRZ-DPSK modulation at 10 Gbps bit rate, respectively. Also, the minimum BER is 1.5 × 10–2 and 2.53 × 10–110 for NRZ and NRZ-DPSK modulation.
Similarly, for RZ with direct detection and RZ-DPSK with balanced detection, the maximum quality factor Q is 2.17 and 21.28. Also, the minimum BER is 1.39 × 10–2 and 5.36 × 10–101, respectively. Figure 5a and b shows the eye diagram for RZ and NRZ-DPSK modulations. Results show RZ and NRZ modulations with direct detection are not suitable for the proposed system. It is obvious that when eye diagram is in best condition (for example Fig. 4b), so the Q and BER are in good condition. For example the NRZ-DPSK modulation has good condition than RZ-DPSK, so exactly the Q and BER parameters have very good operation that it is very obvious.
To study the performance of the proposed system under the influence of different atmospheric condition, attenuation of the different condition is applied to the proposed system. Figure 6a and b shows the diagram of a maximum quality factor versus time for NRZ-DPSK and RZ-DPSK at 10 Gbps, respectively. Results for two modulations show suitable performance for the proposed system. However, the NRZ-DPSK modulation for clear air has a higher maximum quality factor and the RZ-DPSK modulation shows better performance at the unclear atmospheric condition. Also, Table 4 shows the maximum quality factor and minimum BER for the different atmospheric condition at 10 Gbps. It is obvious, because the eye diagram of NRZ-DPSK has good condition, so Q and BER have very good operation in compare with RZ-DPSK modulation format.
To demonstrate the performance of the proposed system, Fig. 7 has been brought. Figure 7a and b show the Min log BER versus of path distance and input power. Results demonstrate that by increasing the value of path distance, the Min BER is increased (Yeh et al. 2019). In the other hand increment the input power reduces the Min BER but enhances the insertion loss of the system. Finally, it can be shown the obtained results for free space optical communication can be used in WDM (Zuo et al. 2017), and advanced applications (Zuo et al. 2015; Zhang et al. 2020b; Zhang et al. 2020c; Zhang et al. 2019a; Liu et al. 2020; Niu et al. 2020; Niu et al. 1998), such as transceiver front end (Liu et al. 2020), multicarrier communication systems (Niu et al. 2020), mmWave UAV Communications (Zhao et al. 2020b), chaotic systems (Wang and Chen 2019), micro-Doppler signatures (Yang et al. 2019), wideband-tunable optoelectronic oscillator (Zhang et al. 2021), fast sliding-mode speed controller (Qu et al. 2021), quantum key distribution (Wang 2021), MBR performance (Sun et al. 2021), and also several materials can be used such as Organic Dye (Zhang et al. 2020d), NaClO (Zhang et al. 2019b), membrane (Sun et al. 2019), graphite (Dai, et al. 2021), carbon based material (Li et al. 2020; Yang et al. 2020; Liu et al. 2021a; Yan et al. 2021) and so on (Liu et al. 2021b; Hou et al. 2021).
6 Conclusion
In this paper, the performance of DWDM-FSO system under outdoor atmospheric channel model is presented. The proposed system is simulated at 10 Gbps bit rate and 2 km path link with different modulation type, NRZ-DPSK and RZ-DPSK. The mathematical model to study the attenuation loss, atmospheric loss and refractive index structure are used to model the outdoor atmospheric channel. Also, the optical power budget was calculated for the proposed system. Results show that the NRZ-DPSK and RZ-DPSK modulations for clear air condition have same and suitable performance, and Min BER for two modulations is less than 10–100. But, in unclear atmospheric weather condition the RZ-DPSK modulation has better performance in comparison to NRZ-DPSK modulation.
Data availability
All data included in this paper are available upon request by contact with the contact corresponding author.
References
Al-Gailani, S.A., Salleh, M.F.M., Salem, A.A., Shaddad, R.Q., Sheikh, U.U., Algeelani, N.A., Almohamad, T.A.: A Survey of Free Space Optics (FSO) communication systems, links, and networks. IEEE Access 9, 7353–7373 (2020)
Ali, R., Ali, M.S., Mir, T., Shabir, B.: Analytical review of advance optical modulation formats. Electr. Electron. Eng.: Int. J. (ELELIJ) 4, 743–749 (2015)
Cai, J.X., Davidson, C.R., Foursa, D.G., Liu, L.: Experimental comparison of the RZ-DPSK and NRZ-DPSK modulation formats. In: Optical Fiber Communication Conference, Vol. 4 (2005)
Chen, H.-L., et al.: An efficient hybrid kernel extreme learning machine approach for early diagnosis of Parkinson’s disease. Neurocomputing 184, 131–144 (2016)
Chen, H., et al.: Multi-population differential evolution-assisted Harris hawks optimization: framework and case studies. Futur. Gener. Comput. Syst. 111, 175–198 (2020)
Ciaramella, E., Arimoto, Y., Contestabile, G., Presi, M., D’Errico, A., Guarino, V., Matsumoto, M.: 1.28 Terabit/s (32x40 Gbit/s) WDM transmission system for free space optical communications. IEEE J. Select. Areas Commun. 27, 1639–1645 (2009)
Couch, L.W.: Digital & Analog Communication Systems, 8th edn. Prentice-Hall, New Jersey (2013)
Dai, Z., et al.: Semiconductor flexoelectricity in graphite-doped SrTiO3 ceramics. Ceram. Int. 47(5), 6535–6539 (2021)
Elsayed, E.E.: Design and Analysis of 1.28 Terabit/s DWDM Transmission System for Free Space Optical Communication (2021)
Elsayed, E.E.: Modified OOK in DWDM-FSO Systems Under Atmospheric Turbulence Channel and Interchannel Crosstalk (2021)
Elsayed, E.E.: Modeling and Performance Enhancement of Hybrid Fiber Network-PON/DPPM-DWDM-FSO Communications under Atmospheric Turbulence Conditions (2021)
Elsayed, E.E., Yousif, B.B.: Performance evaluation and enhancement of the modified OOK based IM/DD techniques for hybrid fiber/FSO communication over WDM-PON systems. Opt. Quant. Electron. 52(9), 1–27 (2020)
Gagliardi, R.M., Karp, S.: Optical Communications, 2nd edn. Wiley, New York (1995)
Garg, N., Kumar, S.: Design of free space optical communication link with Mach-Zehnder optical modulator for long distance. Computing, Communications and Networking Technologies (ICCCNT), pp. 1–5 (2013)
Ghassemlooy, Z., Popoola, W., Rajbhandari, S.: Optical Wireless Communications. CRC Press, Boca Raton (2013)
Gowar, J.: Optical Communication Systems, 2nd edn. Prentice-Hall, New Jersey (1993)
Haris, M.: Advanced Modulation Formats for High-Bit-Rate Optical Networks, USA (2008)
Hou, Z., et al.: Direct ink writing of materials for electronics-related applications: a mini review. Front. Mater. 8, 91 (2021)
Hranilovic, S.: Wireless Optical Communication Systems. Springer, New York (2005)
Hu, L., et al.: An efficient machine learning approach for diagnosis of paraquat-poisoned patients. Comput. Biol. Med. 59, 116–124 (2015)
Hu, J., et al.: Orthogonal learning covariance matrix for defects of grey wolf optimizer: Insights, balance, diversity, and feature selection. Knowl.-Based Syst. 213, 106684 (2020)
Kahn, J., Ho, K.: Spectral efficiency limits and modulation/detection techniques for DWDM Systems. IEEE J. Sel. Top. Quantum Electron. 10, 259–272 (2004)
Kartalopoulos, S.: Introduction to DWDM Technology. SPIE Opt. Eng. Press and IEEE Press, USA (1999)
Killinger, D.: Free space optics for laser communication through the air. Opt. Photonics News 13, 32–42 (2002)
Kruse, P.W., McGlauchlin, L.D., McQuistan, R.B.: Elements of Infrared Technology: Generation, Transmission, and Detection. John Wiley & Sons, New York (1962)
Kumar, N., Singh, T.: 250 Gbit/s optical CDMA over FSO communication system. Optik Int. J. Light Electron Opt. 125, 4538–4532 (2014)
Leibrich, J., Wree, C., Rosenkranz, W.: CF-RZ-DPSK for suppression of XPM on dispersion-managed long-haul optical WDM transmission on standard single-mode fiber. IEEE Photonics Technol. Lett. 14, 155–157 (2002)
Li, M., Li, B., Zhang, X., Song, Y., Chang, L., Chen, Y.: Investigation of the phase fluctuation effect on the BER performance of DPSK space downlink optical communication system on fluctuation channel. Opt. Commun. 366, 248–252 (2016)
Li, C., et al.: Developing a new intelligent system for the diagnosis of tuberculous pleural effusion. Comput. Methods Programs Biomed. 153, 211–225 (2018)
Li, Y., et al.: Point defect model for the corrosion of steels in supercritical water: Part I, film growth kinetics. Corrosion Sci. 163, 108280 (2020)
Liu, X., Kao, Y.-H.: Chirped RZ-DPSK based on single Mach-Zehnder modulator and its nonlinear transmission performance. IEEE Photonics Technol. Lett. 17, 1531–1533 (2009)
Liu, Y., et al.: Development of 340-GHz transceiver front end based on GaAs monolithic integration technology for THz active imaging array. Appl. Sci. 10.21, 7924 (2020)
Liu, Y., et al.: O-, N-Coordinated single Mn atoms accelerating polysulfides transformation in lithium-sulfur batteries. Energy Storage Mater. 35, 12–18 (2021a)
Liu, M., et al.: Dual-channel membrane capacitive deionization based on asymmetric ion adsorption for continuous water desalination. Electrochem. Commun. 125, 106974 (2021b)
Maral, G., Bousquet, M.: Satellites Communications Systems. Wiley, New York (2009)
Marciniak, M.: 100 GB Ethernet over fibre networks-reality and challenges. In: ICTON Mediterranean Winter Conference, ICTON-MW, pp. 1–6 (2007)
Niu, Z.Q., et al.: A mechanical reliability study of 3dB waveguide hybrid couplers in the submillimeter and terahertz band. J. Zhejiang Univ. Sci 1.1 (1998)
Niu, Z., et al.: The research on 220GHz multicarrier high-speed communication system. China Commun. 17(3), 131–139 (2020)
Qu, S., et al.: Design and implementation of a fast sliding-mode speed controller with disturbance compensation for SPMSM syste. IEEE Trans. Transp. Electrif. (2021)
Shan, W., et al.: Double adaptive weights for stabilization of moth flame optimizer: balance analysis, engineering cases, and medical diagnosis. Knowl.-Based Syst. 214, 106728 (2020)
Sharma, A., Kaur, S., Chaudhary, S.: Performance analysis of 320 Gbps DWDM—FSO system under the effect of different atmospheric conditions. Opt. Quant. Electron. 53(5), 1–9 (2021)
Shen, L., et al.: Evolving support vector machines using fruit fly optimization for medical data classification. Knowl.-Based Syst. 96, 61–75 (2016)
Sun, M., et al.: New insights into the rapid formation of initial membrane fouling after in-situ cleaning in a membrane bioreactor. Process Biochem. 78, 108–113 (2019)
Sun, M., et al.: Effects of NaClO shock on MBR performance under continuous operating conditions. Environ. Sci.: Water Res. Technol. 7(2), 396–404 (2021)
Tripathi, A., Gupta, S., Mandloi, A.: Orthogonally polarized and 60 GHz dual-channel based 18× 2.5 Gb/s DWDM-interleaved hybrid FSO system under atmospheric turbulence. Opt. Quant. Electron. 52(4), 1–12 (2020)
Tu, J., et al.: Evolutionary biogeography-based whale optimization methods with communication structure: towards measuring the balance. Knowl.-Based Syst. 212, 106642 (2021)
Wang, B., Chen, L.L.: New results on the control for a kind of uncertain chaotic systems based on fuzzy logic. Complexity 2019, 1–8 (2019)
Wang, M., Chen, H.J.A.S.C.: Chaotic multi-swarm whale optimizer boosted support vector machine for medical diagnosis. Appl. Soft Comput. 88, 105946 (2020)
Wang, M., et al.: Toward an optimal kernel extreme learning machine using a chaotic moth-flame optimization strategy with applications in medical diagnoses. Neurocomputing 267, 69–84 (2017)
Wang, B., et al.: A kind of improved quantum key distribution scheme. Optik 235, 166628 (2021)
Wang, X., Guo, L., Liu, Y., Zhang, L.: Analysis of atmosphere channel for space-to-ground optical communications. Optik - Int. J. Light Electron Opt. 306, 42–48 (2013)
Willebrand, H., Ghuman, B.S.: Free Space Optics: Enabling Optical Connectivity in Today’s Network. SAMS Publishing, Indianapolis (2002)
Winzer, P.J., Essiambre, R.-J.: “Advanced optical modulation formats. Proc. IEEE 94, 952–985 (2006)
Xia, J., et al.: Ultrasound-based differentiation of malignant and benign thyroid Nodules: An extreme learning machine approach. Comput. Methods Programs Biomed. 147, 37–49 (2017)
Xu, X., H-LJSC, : Chen, Adaptive computational chemotaxis based on field in bacterial foraging optimization. Soft Comput. 18(4), 797–807 (2014)
Xu, Y., et al.: Enhanced Moth-flame optimizer with mutation strategy for global optimization. Inf. Sci. 492, 181–203 (2019)
Xu, C., Liu, X., Wei, X.: Ultra-long haul DWDM transmission with differential phase shift keying dispersion managed soliton. ECOC 1, 1–2 (2002)
Yan, X., et al.: A theoretical strategy of pure carbon materials for lightweight and excellent absorption performance. Carbon 174, 662–672 (2021)
Yang, Y., et al.: Omnidirectional motion classification with monostatic radar system using micro-Doppler signatures. IEEE Trans. Geosci. Remote Sensing 58(5), 3574–3587 (2019)
Yang, Y., et al.: Flexible carbon-fiber/semimetal Bi nanosheet arrays as separable and recyclable plasmonic photocatalysts and photoelectrocatalysts. ACS Appl. Mater. Interfaces 12(22), 24845–24854 (2020)
Yeh, C.-H., et al.: Bidirectional free space optical communication (FSO) in WDM access network with 1000-m supportable free space link. Opt. Commun. 435, 394–398 (2019)
Yu, H., et al.: Dynamic Gaussian bare-bones fruit fly optimizers with abandonment mechanism: method and analysis. Eng. Comput. 1–29 (2020)
Yu, C., et al.: SGOA: annealing-behaved grasshopper optimizer for global tasks. Eng. Comput. 1–28 (2021)
Zhang, H., et al.: Fate of NaClO and membrane foulants during in-situ cleaning of membrane bioreactors: combined effect on thermodynamic properties of sludge. Biochem. Eng. J. 147, 146–152 (2019b)
Zhang, B., et al.: A novel 220-GHz GaN diode on-chip tripler with high driven power. IEEE Electron Device Lett. 40(5), 780–783 (2019a)
Zhang, Y., et al.: Boosted binary Harris hawks optimizer and feature selection. Eng. Comput. 25, 26 (2020)
Zhang, J., et al.: On a universal solution to the transport-of-intensity equation. Opt. Lett. 45.13, 3649–3652 (2020b)
Zhang, H., et al.: Degradation of an organic dye by bisulfite catalytically activated with iron manganese oxides: the role of superoxide radicals. ACS Omega 5(29), 18007–18012 (2020d)
Zhang, Y., et al.: Towards augmented kernel extreme learning models for bankruptcy prediction: algorithmic behavior and comprehensive analysis. Neurocomputing 430, 185–212 (2020a)
Zhang, B., et al.: Four-hundred gigahertz broadband multi-branch waveguide coupler. IET Microwaves Antennas Propagation 14(11), 1175–1179 (2020c)
Zhang, J., et al.: Angular velocity measurement with improved scale factor based on a wideband-tunable optoelectronic oscillator. IEEE Trans. Instrument. Measu. 70, 1–9 (2021)
Zhao, X., et al.: Feature selection based on improved ant colony optimization for online detection of foreign fiber in cotton. Appl. Soft Comput. 24, 585–596 (2014)
Zhao, X., et al.: Chaos enhanced grey wolf optimization wrapped ELM for diagnosis of paraquat-poisoned patients. Comput. Biol. Chem. 78, 481–490 (2019)
Zhao, J., et al.: Efficient deployment With geometric analysis for mmWave UAV communications. IEEE Wireless Commun. Lett. 9(7), 1115–1119 (2020b)
Zhao, D., et al.: Chaotic random spare ant colony optimization for multi-threshold image segmentation of 2D Kapur entropy. Knowl.-Based Syst. 216, 106510 (2020a)
Zuo, C., et al.: Transport of intensity phase retrieval and computational imaging for partially coherent fields: the phase space perspective. Opt. Lasers Eng. 71, 20–32 (2015)
Zuo, C., et al.: High-resolution transport-of-intensity quantitative phase microscopy with annular illumination. Sci. Rep. 7.1, 1–22 (2017)
Funding
This research did not receive any specific grant from funding agencies.
Author information
Authors and Affiliations
Corresponding author
Ethics declarations
Conflict of interests
The authors declare that they have no known competing financial interests or personal relationships that could have appeared to influence the work reported in this paper.
Additional information
Publisher's Note
Springer Nature remains neutral with regard to jurisdictional claims in published maps and institutional affiliations.
Rights and permissions
About this article
Cite this article
Alipour, A., Farmani, A. & Mir, A. Analysis of optical power budget in DWDM-FSO link under outdoor atmospheric channel model. Opt Quant Electron 53, 446 (2021). https://doi.org/10.1007/s11082-021-03112-6
Received:
Accepted:
Published:
DOI: https://doi.org/10.1007/s11082-021-03112-6