Abstract
Fucoxanthin is rich in seaweed and considered as effective anti-cancer drug because of powerful antioxidant properties. The objective of this study was to investigate the role of fucoxanthin on apoptosis, invasion and migration of glioma cells. Firstly, fucoxanthin showed obvious cytotoxicity against human glioma cancer cell line U87 and U251, however, there was no inhibitory effect on normal neuron. And then, fucoxanthin induced apoptotic cell death showed by the condensation of chromatin material stained with Hoechest 33342, and reduced mitochondrial membrane potential via DIOC6(3) staining, and enhanced apoptosis by annexin V-FITC/SYTOX Green double staining on U87 and U251 cell lines. Transmission electron microscopy and western blotting were used to determine ultrastructure of U87 cell and expression of proteins related to apoptosis. A scratch wound healing assay and the expression of matrix metalloproteinases (MMPs), and a tans-well assay were used to investigate cell migration and invasion, respectively. Additionally, we uncovered upstream signaling Akt/mTOR and p38 pathways induced by incubation U87 and U251 cell lines with fucoxanthin that mediated cell apoptosis, migration and invasion by using PI3K and p38 inhibitors. Moreover, incubation of fucoxanthin obviously reduced the weight and volume of glioma mass of U87 cells in nude mice. Furthermore, we also examined the glioma mass of U87 cells by hematoxylin-eosin staining, TUNEL assay and western blot, and these outcomes in vivo consistently confirmed that above results in vitro. Taken together, these findings suggest that fucoxanthin augments apoptosis, and reduces cell proliferation, migration and invasion, and reveals a potential mechanism of fucoxanthin-mediated Akt/mTOR and p38 susspression in human glioblastoma cell line.
Similar content being viewed by others
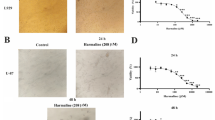
Avoid common mistakes on your manuscript.
Introduction
Gliomas comprise primary central nervous (CNS) system tumors, which are derived from progenitor of glial origin and microglia. Glial origin encompasses ependymal cells, oligodendrocytes and astrocytes, which could transform to ependymomas, oligodendroglioma and astrocytomas, respectively [1]. Astrocytomas are the most common type of primary CNS neoplasm which can be classified grade I to IV in term of a histological/prognostic grading system. According to the classification, the human glioma U87 and U251 cell lines are the kind of Grade IV gliomas, which is known as glioblastoma multiforme (GBM) [2]. As most serious malignant astrocytomas, GBM grows uncontrolled, and invades into neighboring brain structures and metastasizes distantly [3]. Although combine surgical resection with radiotherapy or chemotherapy, it difficulty achieves effective at curing primary due to poor prognosis. Therefore, the development of novel treatment strategies for induction of glioma cell death may be a new line of research into the treatment of glioma.
Fucoxanthin is a major carotenoid extraction from seaweeds and its molecule formula comprises an unusual allenic bond and conjugated carbonyl group [4–5]. Because of these special functional groups, fucoxanthin represents antioxidant activity [6–8], induction of apoptosis in cancer cells [9]. Additionally, current researches focus on the effect of fucoxanthin on inhibition migration and invasion of metastatic tumors. It has been demonstrated that fucoxanthin reduces the ability of invasion and metastasis of melanoma cells and osteosarcoma cells in vitro and in vivo [10–11]. Unfortunately, few reports have been claimed the effect of fucoxanthin on glioma cells, particularly in the category of underlying mechanisms of glioma growth inhibition.
Apoptosis is the term of programmed cell death which is the critical action target on cancer treatment induced by drugs or chemicals [12]. Apoptosis is roughly divided into two types according to the different inductive factor: intrinsic and extrinsic apoptosis [13]. The former class of apoptosis is well known as mitochondrial apoptosis which is characterized by depolarization of membrane potential and activation of caspase family [14]. It has been reported that many botanical drugs display the killing of glioma cells by inducing activation of pro-apoptotic proteins and reduction of anti-apoptosis related proteins [15, 16].
Likewise, the invasion and migration are major causes of malignant glioma U87 and U251-associated mortality. Previous studies have proposed that prevention of tumor exacerbation not only inhibits the proliferation and growth but also blocks the metastasis of glioma cells [17]. Various inspections predict that some drugs have the potential capability to prevent invasion and migration of U87 and U251 cells [18]. Fucoxanthin has effects against tumors due to various pharmacological activity, however, the inhibitory effect of fucoxanthin on U87 and U251 cells remains unclear. Hence, we used human glioma U87 and U251 cells as the cell model to examine potential effects of fucoxanthin on apoptosis, invasion and migration of human glioblastoma cell lines, and investigate the possible molecular targets of anti-caner of fucoxanthin in the present study.
Materials and Methods
Materials
(3-(4,5-dimethylthiazol-2-yl)-2,5-diphenyltetrazolium) bromide (MTT) and fucoxanthin were purchased from Sigma Chemical Company (St. Louis, MO). Hoechst 33342 dye was purchased from Beyotime Biotechnology Inc (Nantong, China). All other chemicals and reagents were of analytical grade. PI3K inhibitor (LY294002) and p38 inhibitor (SB203580), primary polyclonal antibodies against Bcl-2, Bax, caspase-3, caspase-9, PARP, anti-Akt, anti-phospho-Akt, anti-mTOR, anti-phospho-mTOR and secondary antibodies (goat anti-rabbit and rabbit anti-mouse) were purchased from Cell Signaling Technology (Danvers, MA, USA). Primary rabbit polyclonal anti-p38, anti-phospho-p38, anti-ERK, anti-phospho-ERK, anti-JNK, anti-phospho-JNK, anti-MMP-2, anti-MMP-9 and anti-uPA were purchased from Affinity Biosciences (Affinity, Cincinnati, OH, USA).
Cell Line and Animals
The human giloma U87 and U251 cell lines were purchased from the Chinese Academy of Medical Sciences (Beijing, People’s Republic of China). Nude mice (male 5-week-old BALB/c) were obtained from Vitalriver, Beijing, China. The experimental protocol was approved by the Animal Care and Use Committee of General Hospital of Beijing Military Region (approved number: 2015 − 121), and all the procedures were conducted as per the institutional guidelines. Animals were kept in separate cages at room temperature (25 °C), with free access to food and water under a 12 h light–dark cycle for 1 week before the experiment.
Cell Culture
U87 and U251 Cells were cultured in high-glucose Dulbecco’s modified Eagle’s medium (DMEM) (DMEM, Invitrogen, Valencia, CA, USA) supplemented with 10 % foetal bovine serum, 100 μg/mL streptomycin and penicillin (HyClone, Utah,USA) and maintained at 37 °C in an incubator supplemented with an atmosphere containing humidified 5 % CO2 plus 95 % air.
Cell Viability Assay
The effect of fucoxanthin on the viability of glioma U87, U251 cells and normal neurons were determined by using MTT assay, which was based on the reduction of a tetrazolium salt by mitochondrial dehydrogenase in viable cells. Cells of U87 and U251 were seeded on a 96-well plate at a density of 4000 cells/well were treated 16 h later with 6.25, 12.5, 25, 50, 75, 100 μM of fucoxanthin. Normal neurons were planted on a 96-well plate at a density of 1.5 × 104 cells/well were treated 3 days later with 25 and 50 μM of fucoxanthin. After incubation for 12, 24 and 48 h, MTT stock solution (50 µL, 2 mg/mL) was added to each well to yield a total reaction volume of 150 µL. 4 h later, the supernatants were aspirated. The formazan crystals in each well were dissolved in DMSO (150 µL), and the absorbance at 540 nm was read on a scanning multi-well spectrophotometer [19].
Hoechst 33342 Staining Assay
Glioma U87 and U251 cells were treated with fucoxanthin at a concentration of 25, 50 μM after seeding on the coverslips for 16 h. After additional 24 h incubation at 37 °C, U87 and U251cells were washed with PBS and then fixed with 4 % paraformaldehyde for 20 min at room temperature. The DNA-specific fluorescent dye Hoechst 33342 (1.5 µL of 10 mg/mL stock) was added to coverslips and the fixed cells were incubated for 10 min at 37 °C. The stained cells were visualized under a fluorescence microscope (Leica, Germany). The degree of nuclear condensation was evaluated and the apoptotic cells were quantified.
Cell Apoptosis Assay
Apoptosis was explored by staining U87 and U251 cells with Annexin V-FITC/SYTOX Green according to the manufacturer’s recommendations (BD Biosciences, San Diego, CA, USA). Briefly, five hundred thousand cells were seeded into 100 mm dishes incubating for 12 h, and then cells were treated with fucoxanthin (25, 50 μM) for 24 h, and harvested and resuspended at a destiny of 1 × 105 cells/mL with PBS. After 5 μL of Annexin V-FITC was added and mixed well, 5 μL of SYTOX Green was added and mixed well, and the mixture was incubated for 15 min in the dark at room temperature. The stained cells were analyzed with flow cytometry (BD Biosciences, San Diego, CA, USA). The percentage of early and late apoptotic cells was assessed based on histograms generated using CellQuest and ModFit software (Becton Dickinson).
Mitochondrial Membrane Potential (∆ψm)
The U87 and U251 cells were seeded in a 24-well plate at 2 × 105 cells/well and incubated for 12 h. The U87 cells were treated with fucoxanthin (25, 50 μM) for 24 h at 37 °C. After adding 40 nM (final concentartion) of DIOC6(3) solution for 30 min, ∆ψm was analyzed by flow cytometer (BD Biosciences, San Diego, CA, USA).
Transmission Electron Microscopy Analysis
Transmission electron microscopy (TEM) was conducted to observe the ultrastructure of U87 cells after incubation with fucoxanthin. U87 cells were treated with 50 μM fucoxanthin for 24 h, then harvested and fixed for 1 h with 2.5 % glutaraldehyde in a Sörensen 0.1 M phosphate buffer (pH 7.4) at 4 °C. After fixation for 30 min with 1 % osmium tetroxide and deshydratation, cells were embedded into epon. Ultrathin sections were obtained using a Reichert ultracut S ultramicrotome and mounted on copper grids. Sections were stained with uranyl acetate for 15 min, and lead citrate for 3 min and were examined Jeol 100 CX II transmission electron microscope at 60 kV (Tokyo, Japan).
Scratch Wound Healing Assay
The U87 and U251 cells were seeded at a density of 1 × 105 cells/well in 6-well plates, and incubated for 12 h. The cell monolayer was scraped in a straight line using a 200-μL pipette tip and the cells were washed three times with PBS. The cells were incubated in free media and various concentrations of fucoxanthin (25 and 50 μM) for 18 h. The control group was treated with an equal volume of solvent (DMSO) in culture medium. Images were captured under phase-contrast microscope (Leica, Germany). ImageJ2x software (Rawak Software, Inc. Germany) was used to analyse the relative migration distances.
Trans-Well Invasion Assay
U87 and U251 cells were resuspended at a destiny of 2 × 104 cells/well with medium containing 1 % FBS. Trans-well chambers (Corning Life Sciences), which precoated with 100 μL Matrigel (BD Biosciences, Bedford, MA, USA) diluted with culture medium. Medium contained 20 % FBS in the lower chambers served as the chemoattractant. Fucoxanthin (25, 50 μM) was added in the lower chambers. Non-invading cells were removed with cotton swabs after 24 h, while invading cells were dyed with 0.1 % crystal violet (Amresco, Inc., Solon, OH, USA). Washed the trans-well chambers with the water and captured images by using Image-Pro Plus software under a phase-contrast microscope (magnification, 200×). Crystal violet in the invading cells was dissolved with 500 μL of 33 % acetic acid. The absorbance of crystal violet dissolved in acetic acid was measured at 570 nm using a microplate reader.
Trans-Well Migration Assay
U87 and U251 cells were resuspended at a destiny of 2 × 104 cells/well with medium containing 1 % FBS. Medium contained 20 % FBS in the lower chambers served as the chemoattractant. Fucoxanthin (25, 50 μM) was added in the lower chambers. Non‑invading cells were removed with cotton swabs after 24 h, while invading cells were dyed with 0.1 % crystal violet. Washed the trans-well chambers with the water and captured images by using Image-Pro Plus software under a phase-contrast microscope (magnification, 200×). Crystal violet in the invading cells was dissolved with 500 μL 33 % acetic acid. The absorbance of crystal violet dissolved in acetic acid was measured at 570 nm using a microplate reader.
Western Blot Analysis
Cells were lysed on ice for 30 min in 100 µL lysis buffer (120 mM NaCl, 40 mM Tris (pH 8), and 0.1 % NP-40) and centrifuged at 13,000×g for 15 min. Supernatants were collected and the protein concentrations were determined. Aliquots containing 40 µg of protein were boiled for 5 min and electrophoresed on 10 % SDS–polyacrylamide gels. Proteins were transferred to nitrocellulose membranes, which were subsequently incubated with a primary antibody (dilution of 1:1000) overnight at 4 °C. The membranes were further incubated with horseradish peroxidase-conjugated anti-immunoglobulin-G (Pierce, Rockford, IL, USA). Protein bands were detected using an enhanced chemiluminescence western blotting detection kit (Amersham, Little Chalfont, Buckinghamshire, UK).
In Vivo Tumour Xenograft Study
Five-week-old female nude mice, 20–25 g body weight (BALB/c-nude; Vitalriver, Beijing, Beijing, People’s Republic of China) were housed into individually ventilated cages, and free accessed to food and drinking water. U87 cell (5 × 106 cells) dissolved in 0.1 ml PBS was injected subcutaneously into the abdominal. Tumor sizes were measured with a caliper and calculated as tumor size (mm3) = 1/2 × length × width2.
The tumor-bearing mice were randomly divided into two groups (n = 6/group) and treatment of mouse with fucoxanthin was initiated on the day after cell injection. Fucoxanthin was dissolved in soybean oil and 1 g/kg or soybean oil by oral gavage every day for 28 days. Tumor sizes and body weights were measured two or three times weekly. On day 28, all mice were euthanized. Primary tumors were dissected out for measurement of weight and volume, and related proteins with western blot, and staining with hematoxylin and eosin (H&E) and terminal deoxynucleotidyl transferase-mediated dUTP nick-end labeling (TUNEL) using a commercial kit (Beyotime Biotechnology Inc, Nantong, China).
Statistical Analysis
All measurements were performed in triplicate and all values are expressed as the mean ± the standard error. The results were subjected to an analysis of variance (ANOVA) using Tukey’s test to analyze differences between means. In each case, a P value <0.05 was considered statistically significant.
Results
Fucoxanthin Inhibites Cell Proliferation of Human Glioblastoma Cells
The effect of fucoxathin on inhibition of U87 and U251 cells growth were detected by MTT assay, which was conducted in a concentration- and time-dependent manner. The MTT data revealed that treatment U87 and U251 cells with 25, 50, 75 and 100 µM of fucoxanthin significantly decreased the level of cell viability incubation for 12, 24 and 48 h, compared with blank control and control groups (Fig. 1a, b). It is noteworthy that fucoxanthin had no suppression on the cell viability of normal neurons which indicated that the drug could be developed into the clinical chemicals because of avirulence for human normal neurons. Additionally, the lower concentration of fucoxanthin (6.25 and 12.5 µM) was not cytotoxic towards glioma cells and 25 µM is the middle concentration to initiate the anti-proliferation of fucoxanthin. Based on these results, 25 and 50 µM were chosen as the optimal concentrations of fucoxanthin for further investigation.
Fucoxanthin attenuates glioblastoma cells generation. Fucoxanthin was added to a U87 and b U251 cells at a concentration of 6.25, 12.5, 25, 50, 75 and 100 μM, and after 12, 24 and 48 h cell viability was determined by the MTT assay. c The normal neurons were exposed to culture medium containing various concentrations of fucoxanthin for 72 h. *Significant difference from control (p < 0.05). Each bar represents the mean ± SEM in triplicate experiments
Fucoxanthin Induces Apoptotic Progress in Human Glioblastoma Cells
To evaluate the toxic effect of U87 and U251 cells on apoptosis induced by fucoxanthin, the cellular nuclei were stained with hoechst 33342 and then assessed by microscopy. Observation with microscopy, fracted nuclei were both viewed in fucoxanthin-treated U87 and U251 cells at 25 and 50 µM compared to intact nuclei observed in control cells (Fig. 2a) and the apoptotic rates were 14.32 and 17.00 at 25 µM, and 28.36 and 27.31 at 50 µM, respectively. Similarly, the expression of H2AX, a hallmark of chromosome damage, was increased which indicated that fucoxanthin impaired U87 and U251 cells via apoptosis initiation (Fig. 2d). In addition, depolarization of mitochondrial potential (∆ψm) is indispensable part involved in apoptotic program and we examined whether fucoxanthin caused apoptosis via disruption of mitochondria normal function. DiOC6(3) is a cyanine dye that suggests ∆ψm change by shifting its fluorescence emission from blue to yellow [20]. As shown in Fig. 2b, the maximum absorption wavelength was blue shift after treatment U87 and U251 cells with fucoxanthin by showing reduced GEO means suggesting the disruption of ∆ψm and destroyed the mitochondrial function, and effect of 50 µM was better than that of 25 µM concentration. Impaired mitochondrial membrane releases the cytochrome c into cytoplasm which activates the apoptosis progression [21]. As shown in the Fig. 2c, apoptosis was detected in the fucoxanthin-incubated cells by performing Annexin V-FITC/SYTOX Green double staining which could distinguish different stage of apoptotic cell. Positive staining of annexin means the early apoptosis by binding of phosphatidylserine which changes the location from the inner to outer of cell membrane at the beginning of the apoptosis [22]. SYTOX Green then binds to DNA when membrane permeability is increased caused by further development of apoptosis [23]. The degree of early and late apoptosis of U87 and U251 were 2.16 and 12.42 %, and, 12.64 and 22.97 % at the lower concentration of fucoxanthin, respectively, and it increased to 3.62 and 20.13 %, and, 16.76 and 23.97 % at the higher concentration which were significant difference compared with that of control groups (Fig. 2c). To further understand the mechanism of fucoxanthin-induced apoptotic process, we explored the expressions of proteins related to mitochondrial apoptosis. Firstly, we examine the changes of protein expression between Bcl-2 and Bax, which are anti-apoptotic protein and pro-apoptotic protein. As shown in Fig. 2d, fucoxanthin showed an increment in Bax expression and a decrement in Bcl-2 expression compared with the control cells both in U87 and U251 cells. The balance of Bcl-2/BAX dominates the switch which turns on or off the apoptosis [24]. Notably, fucoxanthin initiated the activation of apoptotic pathway via Bax folded expression and it transduces the signal to the downstream-caspase system [25]. As expected, the enhanced activation of cleaved-PARP, caspase-9 and -3 was showed in fucoxanthin-treated groups (Fig. 2e). Likewise, similar result was also obtained by electron microscopy. Transmission electron microscope (TEM) could find some changes of ultrastructure, including organelle and chromosome [26]. We visualized the phenomenon of early and late apoptosis after treatment of U87 cells with fucoxanthin by means of electron microscope. The middle picture displayed the chromosome condensation which suggested that fucoxanthin initiated the apoptotic process as well as the early stage of apoptosis after incubation for short period (Fig. 2f). And then, the enriched chromatin was moved to the edge of nuclear membrane shown in right one, which was the indicator of advanced period of apoptosis. These results suggest that fucoxanthin promotes cell death by induction apoptosis via mitochondrial pathway.
Fucoxanthin induces the activation of glioblastoma cells apoptosis progression. a Apoptotic bodies were observed by fluorescence microscopy in cells stained with Hoechst 33342 dye and quantified. *Significant difference from control (p < 0.05), and #significant difference from lower concentration-treated cells (p < 0.05). b ∆ψm was analyzed by flow cytometer after staining cells with DIOC6(3) (GEO, geometric). *Significant difference from control (p < 0.05), and #significant difference from lower concentration-treated cells (p < 0.05). c Different stages of apoptosis were performed by flow cytometry. *Significant difference from control (p < 0.05), and #significant difference from lower concentration-treated cells (p < 0.05). d, e Cell lysates were electrophoresed and apoptotic proteins were detected by their respective specific antibodies in indicated concentrations. Each bar represents the mean ± SEM in triplicate experiments. f Observation of the microstructure of U87 cells treated with fucoxanthin. Left image untreated cells had a normal nucleus, cytoplasm and organelle morphology. Middle image the early stage of apoptosis had chromosome condensation after treatment U87 cells with 50 μM for 12 h. Right image the late stage of apoptosis moved enriched chromatin to area around nuclear membrane after treatment U87 cells with 50 μM for 24 h
Fucoxanthin Inhibits the Invasion and Migration of Human Glioblastoma Cells
Invasiveness and migration are the pathological signatures of human malignant gliomas, the effects of fucoxanthin on the invasiveness and migration of U87 cells were checked by scratch wound healing assay and trans-well assays [27]. The scratch wound healing assay revealed that both lower and higher concentration of fucoxanthin exposure remarkably restrained the invasion of U87 and U251 cells showing by the reduced healing rate compared to that of untreated-cells (Fig. 3a). Similarly, another outcome of the in vitro, trans-well assay, certified that treatment of U87 and U251 cells with fucoxanthin obviously reduced cells to infiltrate the membranes with (Fig. 3b) or without matrigel (Fig. 3c) compared with untreated groups which indicated that fucoxanthin indeed had ability to control the translocation of U87 cells to adjacent tissue or other parts of body. Besides, we further investigated the mechanisms underlying fucoxanthin-inhibited properties of migration and invasion of glioblastoma cells. It has been proven that the increased degree of matrix metalloproteases (MMPs) expression is closely associated with invasiveness and migration of human gliomas [28]. MMPs promote tumor growth, angiogenesis, metastasis and invasion by degradation of extracellular matrix to break down structure of tissue and cell or provide various growth factors [29]. Therefore, we selected two kind of gelatinase, MMP-2 and MMP-9, as the objective to explore the potential mechanism of fucoxanthin on human glioblastoma cells. As expected, the protein levels of MMP-2 and MMP-9 were undoubtedly suppressed by incubation glioma cells with fucoxanthin at the concentration of 25 and 50 µM (Fig. 3d). The serine protease urokinase-type plasminogen activator (uPA) is upstream signaling and regulates the expression of MMPs to influence the characteristics of malignant glioma cells [30]. As shown in Fig. 3d, we observed that the expression of uPA was reduced by exposure of glioma cells with fucoxanthin suggesting the effect of drug on U87 and U251 cells via inhibition of uPA-MMPs systems. These findings indicate that the application of fucoxanthin inhibits the invasive and migrative ability of glioma cells in vitro.
Fucoxanthin suppresses the invasion and migration of glioblastoma cells. a Images of the scratchwound healing assay were captured at 0 and 18 h, and the wound gaps were analyzed by measuring the distance of migrating cells for three regions of each wound. *Significant difference from control (p < 0.05), and #significant difference from lower concentration-treated cells (p < 0.05). b Cells were incubated with indicated concentrations of fucoxanthin for 24 h. Photographs are of the cell invasion through the matrigel membrane stained by crystal violet. c Cells were treated with indicated concentrations of fucoxanthin for 24 h. The representative images of migrate cells at the bottom of the membrane stained with crystal violet were visualized. *Significant difference from control (p < 0.05), and #significant difference from lower concentration-treated cells (p < 0.05). d Cell lysates were electrophoresed and MMP-2/9 and uPA proteins were detected by their respective specific antibodies in indicated concentrations. *Significant difference from control (p < 0.05), and #significant difference from lower concentration-treated cells (p < 0.05)
Fucoxanthin Induces Apoptosis by Inhibition of PI3K/Akt/mTOR Signaling Pathway
It’s well known that apoptosis is closely related to many molecular signaling pathways which are involved in the process of initiation, activation or deactivation. It has been reported that the apoptosis is down-regulated by the PI3K/Akt/mTOR signaling pathway [31]. Moreover, the induction of the programmed cell death, as termed apoptosis, is a promising therapeutic approach and target when the PI3K/Akt/mTOR system is inhibited by drugs treatment [32]. Therefore, we confirmed that whether the effect of fucoxanthin on apoptosis of glioma cells was caused by negative regulation of PI3K/Akt/mTOR pathway. As shown in Fig. 4a, we observed that the expression of phosphorylation of Akt and mTOR was dramatically decreased by treatment of glioma cells with fucoxanthin at the concentration of 25 and 50 µM, and the level of phosphorylated mTOR of glioma cells was notably reduced only at higher concentration of fucoxanthin. These results suggest that fuocoxanthin indeed inhibites the PI3K/Akt/mTOR pathway by reduction of their phosphorylated forms. To further prove the positive effect of fucoxanthin on apoptosis via suppression of PI3K/Akt/mTOR system, we incubated glioblastoma cells with PI3K inhibitor prior to fucoxanthin treatment (50 µM). Undoubtedly, the expressions of active form of Akt/mTOR were lessened by exposure of glioma cells with fucoxanthin or PI3K inhibitor, individually (Fig. 4b). Ulteriorly, the application of LY294002 had the semblable bands with fucoxanthin of western blotting on the expression of Bax, Bcl-2, cleaved-caspase-9, which indicated that the inhibition of PI3K/Akt/mTOR by fucoxanthin exactly induced the activation of apoptosis (Fig. 4b). Eventually, the combination of LY294002 and fucoxathin had more effect on apoptosis related proteins, compared to that of fucoxanthin or PI3K inhibitor. These results demonstrate that the inductive effect of fucoxanthin on apoptosis is performed via suppression of PI3K/Akt/mTOR system.
Fucoxanthin promotes apoptosis via inhibition of PI3K/Akt/mTOR signaling pathway in glioblastoma cells. a Cell lysates were electrophoresed and Akt, p-Akt, mTOR and p-mTOR proteins were detected by their respective specific antibodies in indicated concentrations. *Significant difference from control (p < 0.05), and #significant difference from lower concentration-treated cells (p < 0.05). After treatment with LY294002 for 1.5 h, and with fucoxanthin for 24 h, (b) apoptotic related proteins were detected using their respective specific antibodies in indicated times. *Significant difference from control (p < 0.05), #significant difference from fucoxanthin-treated cells (p < 0.05), and ##significant difference from LY294002-treated cells (p < 0.05)
Fucoxanthin Suppresses the Capability of Invasion and Migration by Inhibition of p38 Signaling Pathway
Previous reports have demonstrated that MMPs-caused invasion and migration of tumor cells are mediated by the family of mitogen-activated protein kinases (MAPKs) [33]. There three classes of MAPK, including extracellular signal-regulated kinases (ERK), stress-activated JUN N-terminal kinases (JNKs) and p38 [34]. Next, we investigated that the whether the diminishment of invasion and migration was facilitated by MAPKs after treatment of cells with fucoxanthin. As illustrated in Fig. 5a, the degree of phosphorylated-p38 expression was markedly decreased in a concentration-dependent manner. Conversely, fucoxanthin abnormally increased the protein levels of phosphorylated-ERK which should promote the cancerous cells to invade or infiltrate into other parts. However, fucoxanthin could not influence the expression of p-JNK/JNK. Therefore, we verified that if the p38 was the upstream target of MMPs which was inhibited by chemical in our article. As expected, the MMPs was significantly reduced by the p38 inhibitor (SB203580) treatment which indicated that the p38-MMPs signaling pathway involved the fucoxanthin-mediated the reduction of disruption of other normal tissue of glioma cells (Fig. 5b). Hence, fucoxanthin negatively regulated the activity of glioma cells on invasion and migration mediated by suppression of activation of p38.
p38 kinase involves the fucoxanthin-mediated reduction of invasion and migration of U87 cells. a Cell lysates were electrophoresed and ERK, p-ERK, p38 and p-p38 proteins were detected by their respective specific antibodies in indicated concentrations. *Significant difference from control (p < 0.05), and #significant difference from lower concentration-treated cells (p < 0.05). After treatment with SB203580 for 1.5 h, and with fucoxanthin for 24 h, (b) metastasis related proteins were detected using their respective specific antibodies in indicated times. *Significant difference from control (p < 0.05), #significant difference from fucoxanthin-treated cells (p < 0.05), and ##significant difference from SB203580-treated cells (p < 0.05)
Effects of Fucoxanthin on Glioblastoma Xenografts of U87 Cells in BALB/c-Nude Mice
We had demonstrated that fucoxanthin was capable of inhibition of proliferation, invasion and migration, and induction of apoptosis on U87 cells in vitro. Next, we inspected that whether fucoxanthin had the similar results in vivo. We assessed the anti-cancer capability of fucoxanthin using immune deficient nude mice (BALB/c-nude) as the testing model. Subcutaneous injection of U87 cells significantly increased from 234 mm3 to 2647 mm3 in size within 3 weeks, respectively, exhibiting rapid growth of glioma cells in the host mice (Fig. 6a). However, the rates of tumor growth in fucoxanthin-treated group was conspicuously reduced showing the average tumor volume were 1644 mm3. Additionally, we examined the function of fucoxanthin on U87 cells in vivo by histochemistry and immunohistochemistry methods. H&E staining illustrated that the cell density was declined and the morphology was changed with irregular cell shape, and scattered nuclei showing by light and uneven purple color (Fig. 6b). TUNEL assay certified that apoptosis was appeared after treatment U87 cell with fucoxanthin showing by staining aberrated chromosome with brown color (Fig. 6b). And then, we explored the underlying signaling pathway mediated by fucoxanthin in vivo via extraction proteins from tumor mass of U87 cells. As illustrated in Fig. 6c, fucoxanthin enhanced the expression level of apoptotic-related proteins and inhibited the activation of PI3K/Akt/mTOR and p38 system to accomplish reduction of invasion and migration of U87 tumor. These results denote that administration of these mice with 200 mg/kg/day of fucoxanthin after inoculation of U87 cells significantly reduce of the tumor volume and weight due to the emerging apoptotic cells and decline of invasion and migration in the U87 cells.
Fucoxanthin inhibited U87 xenograft growth in vivo. a U87 cells were injected into the nude mice and tumors were allowed to grow. Subsequently, fucoxanthin (dissolved in soybean oil, 1 g/kg/day body weight) was given by oral gavage daily i.p. for 28 day (n = 6). The control group was given a gavage of soybean (1 g/kg body weight) every day i.p. (n = 6). The changes in tumor weight and growth were shown. *Significant difference from control (p < 0.05), and #significant difference from lower concentration-treated cells (p < 0.05). b Pathological sections derived from tumor mass were examined by HE staining and TUNEL assay. *Significant difference from control (p < 0.05), and #significant difference from lower concentration-treated cells (p < 0.05). c At the end of the experiment, tumor tissues were excised from mice, and the protein lysates extracted from the tumor tissues were assessed by immunoblotting for p-Akt, p-mTOR, p-p38, MMP-2/9, BCL-2, BAX and cleaved-caspases-9, respectively. *Significant difference from control 1 (p < 0.05), #significant difference from control 2 (p < 0.05), and &significant difference from control 3 (p < 0.05)
Discussion
In our study, we applied glioma U87 and U251 cells as a testing model to explore the role of the fucoxanthin on the induction of apoptosis, and suppression of invasion and migration. The main question addressed by this study was whether fucoxanthin could exhibit anti-cancer property on glioma cells and excavated the feasible mechanism that played a pivotal role in the functions of fucoxanthin on U87 and U251 cells. Herein, we proven that fucoxanthin promoted apoptosis through activation of PI3K/Akt/mTOR, and suppressed invasion and migration via involvement of p38/MMPs signaling pathway, leading to the novel chemical strategy of defense of malignant glioma cells.
Gliomas are the most common malignant primary brain tumors with enhanced signal of computed tomography and magnetic resonance image [1], and U87 and U251 cell lines in this study are belonging to GBM which presents irregular growth pattern of cell proliferation and angiogenesis, and robustly invade or infiltrate into the surrounding normal brain tissue or migrate to distant regions of body [35–37]. Although employing resection surgery, radiation or chemotherapy, it ultimately difficult to achieve complete killing the cancer cells, therefore, the new treatment method and therapeutic targets are indeed required [38]. Previous studies have certified that vitamin A or its metabolic intermediates retinoic acid possess pretty effect on treatment of glioma cells in the aspects of inhibition of growth, and induction of apoptosis, cell cycle arrest, and prevention of invasion and migration [39–43]. As carotenoid, fucoxnthin has the similar chemical structure with retinoic acid, sharing the conjugated groups and active hydroxyl groups, and may display the anticipant functions. However, there is rare report about fucoxanthin’s effect on glioma cells, hence, we investigated the foreseeable effect of fucoxanthin on the objective U87 and U251 cells.
Firstly, we explored that fucoxanthin reduced cell viability in a dose-dependent manner via MTT assay, suggesting the inhibitory efficacy of fucoxanthin on glioblastoma cells (Fig. 1a–b). As an added bonus, fucoxanthin did not impair the cell growth of normal neurons. Then, we investigated that whether fucoxanthin reduced cell proliferation via activation of programmed cell death apoptosis. Hoechst 33342 is an indicator of apoptosis which detects chromatin condensations and binds to them [44]. Observation with microscopy, the binding between Hoechst 33342 and apoptotic cells was apparently increased after incubation of U87 and U251 cells with fucoxanthin in concentration-dependent manner (Fig. 2a). In addition, the double-strand breaks of DNA caused by external stress rapidly recruits abundant γ-H2AX which could be visualized by western blotting [45]. As shown in Fig. 2d, the expression of γ-H2AX was obvious accumulation after treatment with higher concentration of fucoxanthin. As a dynamic process, initiation, activation and execution comprise the entire system of apoptosis, and Hoechst 33342 and γ-H2AX are on behalf of primary stage of apoptosis. Next, the activation of apoptosis is characterized by mitochondrial membrane potential (∆ψm) alteration and abnormal expression of apoptotic related proteins [46, 47]. The staining result of DiOC6(3) revealed that the depolarization of ∆ψm of glioma cells were evidently exacerbated by exposure to fucoxanthin which not only displaced the maximum absorption wavelength but also influenced the intensity of fluorescence (Fig. 2b). Later, we analyzed the percentage of different stage of apoptotic cells through flow cytometry, and both early and late apoptosis cells were markedly augmented by fucoxanthin confirmed by SYTOX Green and FITC-Annexin staining, respectively (Fig. 2c). Moreover, the repression of ∆ψm changes the level of apoptotic proteins in mitochondrial and amplifies the permeability of mitochondrial membrane to release of cytochrome c into cytoplasm, and activates the induction of Bax/Bcl-2 ratio and execution of caspase cascade [48]. The expression of Bcl-2 and Bax was significantly declined and added by 50 µM fucoxanthin, individually (Fig. 2d). On the other hand, the pro-apoptotic proteins were remarkably increased by the drug including cleaved-PARP, -caspase-3 and -caspase-9 (Fig. 2e). Furthermore, we visualized the phenomenon of early and late apoptosis after treatment of U87 and U251 cells with fucoxanthin by means of electron microscope. In conclusion, fucoxanthin evoked the mitochondrial apoptosis to implement the inhibitory effect of fucoxanthin on glioblastoma cells.
Next, we uncovered the upstream signaling pathway which took participated in the fucoxanthin-mediated apoptosis. It has been reported that PI3K/Akt/mTOR system negatively regulates the programmed cell death in the gliomas, resulting in deterioration of normally pathophysiologic functions. Phosphatidylinositol 3 kinase (PI3K) promotes accumulation of phosphatidylinositol (3–5) triphosphate [PtdIns (3,4,5)P3] by phosphorylation, which is an anchor for the Akt/protein kinase B (PKB/Akt) and 3-phosphoinositide-dependent protein kinase 1 (PDK1) [49–51]. Then, Akt translocates to inner side of the cytoplasmic membrane and becomes the active form by PDK1 phosphorylation [52]. Ultimately, Akt dislodges the inhibitor of mammalian target of rapamycin (mTOR), tuberous sclerosis complex (TSC1/2), leading the activation of mTOR and whole PI3K/Akt/mTOR system is finally activated [53]. More reports have been claimed that the activation of Akt directly or indirectly suppresses the apoptosis by influence the apoptosis related proteins, such as phosphorylation of Bax and caspase-9 [54, 55]. Compared to control group, the accumulation of phosphorylated-Akt and -mTOR was significantly decreased (Fig. 4a), and the degree of apoptosis was enhanced (Fig. 2) by application of fucoxanthin. To further investigate the possible relationship between programmed cell death and PI3K/Akt/mTOR signaling pathway, we utilized the PI3K inhibitor on western blotting. The handle of PI3K inhibitor certified that the activation of apoptosis indeed was caused by the inhibition of PI3K/Akt/mTOR, and the similar results were observed in fucoxanthin-treated cells (Fig. 4b). Of particular importance is the combination of PI3K inhibitor and fucoxanthin induced more level of apoptosis which indicated that simultaneously treatment incorporates or catalyze the capability of LY294002 or fucoxanthin for induction of apoptosis, or fucoxanthin maybe activates programmed cell death not only via restriction of PI3K/Akt/mTOR system but also other potential signaling pathway which could be a more interesting point in future.
Invasion and migration are thorny barriers of treatment of glioma with various methods for therapy. Malignant glioma cells invade ambient area to destroy normal functions of tissue, cells and organelle, or migrate to far away regions to form tissue mass, resulting in disfunction of body’s organs. The matrix metalloproteinases (MMPs) play an important role in tissue remodeling associated with various physiological or pathological processes such as morphogenesis, angiogenesis, tissue repair, invasion and metastasis which is activated by cytokines, growth factors, tumor promoters, physical stress, oncogenic transformation, and cell-matrix and cell–cell interactions [56, 57]. The amplifying impact of MMPs derived from cancer is the beginners of caner pathogenesis and promotes cell proliferation, angiogenesis, invasion and metastasis by the degradation of the extracellular matrix [58]. Therefore, reduction of activity of MMPs is thought to be important target which prevents the tumor spread and migration. More researchers have demonstrated that MMP-2 and MMP-9 modulate the migration and invasion in glioma cells [59, 60]. In our data, fucoxanthin inhibited the invasion and migration via evidenced by scratch wound healing assay and trans-well with or without matrigel (Fig. 3a–c). In addition, the bands of western blotting supported the relationship between fucoxanthin-prohibitive metastasis and the reduced expressions of MMP-2 and MMP-9 (Fig. 3d). As expected, the serine protease urokinase-type plasminogen activator (uPA), upstream signal of MMPs, was down-regulated by fucoxanthin. Currently, many proofs sustain that reductive cell motility and migration is via suppression of p38-MMP-2/9 signaling pathway, suggesting a potential mechanism existing in fucoxanthin-treated glioblastoma cells [61, 62]. The western blots of three isoforms of MAPKs revealed that inducer of fucoxanthin-regulated invasion or spared in glioblastoma is p38 rather than ERK or JNK kinase (Fig. 5a). Then, fucoxanthin exerted approximate outcomes that induced by incubation of glioblastoma cells with p38 inhibitor (Fig. 5b). Analogously with data of PI3K inhibitor, the effect of co-treatment of U87 and U251 cells with fucoxanthin and p38 inhibitor on the p38-MMP-2/9 core proteins had the significant differences with that of only fucoxanthin or SB203580, respectively, suggesting underlying mechanism existing apart from p38-MMP-2/9 central pivot (Fig. 5b). Therefore, prospective studies are exploring deep into the involvement signaling pathway of fucoxanthin independent of p38-MMP-2/9 operating system. In a word, fucoxanthin depresses the activity of p38-MMP-2/9 signal reflection and then lowers the extent of invasion and migration.
Moreover, the tumor xenograft studies shown that the tumor volume and weight were obviously declined reflecting the inhibitory effect of fucoxanthin on the tumor mass of U87 cells (Fig. 6a). Besides, staining histologic sections with H&E dye indicated that inflammation and low cell density were appeared by fucoxanthin compare to that of control cells (Fig. 6b). Moreover, we observed the presence of apoptosis conducted by TUNEL assay (Fig. 6b). Furthermore, we inspected that the molecules such as p-Akt, p-mTOR, p-p38, MMP-2/9 and BCL-2 were all down-regulated, and BAX and cleaved-caspases-9 were both up-regulated in tumor tissues after fucoxanthin treatment which was consistent with in vitro findings.
In summary, we present the first report of anticancer activity of fucoxanthin in glioblastoma cells, and this is also the first evidence that fucoxanthin undertakes a suppresser of cell migration and invasion in vitro and in vivo. In the present study, fucoxanthin significantly promotes apoptosis via inhibition of PI3K/Akt/mTOR signaling pathway, leading to the inhibition of cell proliferation of glioblastoma cells. In addition, p38-MMP-2/9 signaling pathway involves the fucoxanthin-mediated inhibition of invasion and migration of glioma cells. Therefore, as an active ingredient of marine algae with considerable toxicity, fucoxanthin may represent a new anticancer drug that can impair glioma cells and prevent metastasis, and will become an emerging therapeutic agent in the future.
References
Rao JS (2003) Molecular mechanisms of glioma invasiveness: the role of proteases. Nat Rev Cancer 3:489–501
Dunbar E, Yachnis AT (2010) Glioma diagnosis: immunohistochemistry and beyond. Adv Anat Pathol 17:187–201
Liang C, Yang L, Guo S (2015) All–retinoic acid inhibits migration, invasion and proliferation, and promotes apoptosis in glioma cells. Oncol Lett 9:2833–2838
Sugawara T, Baskaran V, Tsuzuki W, Nagao A (2002) Brown algae fucoxanthin is hydrolyzed to fucoxanthinol during absorption by Caco-2 human intestinal cells and mice. J Nutr 132:946–951
Yan X, Chuda Y, Suzuki M, Nagatat T (1999) Fucoxanthin as the major antioxidant in hifikia fusiformis, a common edible seaweed. Biosci Biotechnol Biochem 63:605–607
Hu T, Liu D, Chen Y, Wu J, Wang S (2010) Antioxidant activity of sulfated polysaccharide fractions extracted from Undaria pinnitafida in vitro. Int J Biol Macromol 46:193–198
Sachindra NM, Sato E, Maeda H, Hosokawa M, Niwano Y, Kohno M, Miyashita K (2007) Radical scavenging and singlet oxygen quenching activity of marine carotenoid fucoxanthin and its metabolites. J Agric Food Chem 55:8516–8522
Heo SJ, Jeon YJ (2009) Protective effect of fucoxanthin isolated from Sargassum siliquastrum on UV-B induced cell damage. J Photochem Photobiol B Biol 95:101–107
Heo SJ, Ko SC, Kang SM, Kang HS, Kim JP, Kim SH, Lee KW, Cho MG, Jeon YJ (2008) Cytoprotective effect of fucoxanthin isolated from brown algae Sargassum siliquastrum against H2O2-induced cell damage. Eur Food Res Technol 228:145–151
Chung TW, Choi HJ, Lee JY, Jeong HS, Kim CH, Joo M, Choic JY, Han CW, Kim SY, Choi JS, Ha KT (2013) Marine algal fucoxanthin inhibits the metastatic potential of cancer cells. Biochem Biophys Res Commun 439:580–585
Rokkaku T, Kimura R, Ishikawa C, Yasumoto T, Senba M, Kanaya F Mori N (2013) Anticancer effects of marine carotenoids, fucoxanthin and its deacetylated product, fucoxanthinol, on osteosarcoma. Int J Oncol 43:1176–1186
Zhou T, Ye L, Bai Y, Sun A, Cox B, Liu D, Li Y, Liotta D, Snyder JP, Fu H, Huang B (2014) Autophagy and apoptosis in hepatocellular carcinoma induced by EF25-(GSH)2: a novel curcumin analog. PloS One 9:e107876
Gao X, Deeb D, Jiang H, Liu YB, Dulchavsky SA, Gautam SC (2005) Curcumin differentially sensitizes malignant glioma cells to TRAIL/Apo2L-mediated apoptosis through activation of procaspases and release of cytochrome c from mitochondria. J Exp Ther Oncol 5:39–48
Zajc I, Hreljac I, Lah T (2006) Cathepsin L affects apoptosis of glioblastoma cells: a potential implication in the design of cancer therapeutics. Anticancer Res 26:3357–3364
Li Q, Lu XH, Cai L, Lu JL, Wu JS, Zhuge QC, Zheng WM, Su ZP (2015) Antiproliferative and apoptosis-inducing activity of schisandrin B against human glioma cells. Cancer Cell Int 15:12
Yang JA, Li JQ, Shao LM, Yang Q, Liu BH, Wu TF, Wu P, Yi W, Chen QX (2015) Puerarin inhibits proliferation and induces apoptosis in human glioblastoma cell lines. Int J Clin Exp Med 8:10132–10142
Zhang FY, Hu Y, Que ZY, Wang P, Liu YH, Wang ZH, Xue YX (2015) Shikonin inhibits the migration and invasion of human glioblastoma cells by targeting phosphorylated beta-catenin and phosphorylated PI3K/Akt: a potential mechanism for the anti-glioma efficacy of a traditional chinese herbal medicine. Int J Mol Sci 16:23823–23848
Nie XH, Ou-Yang J, Xing Y, Li DY, Liu RE, Xu RX (2016) Calycosin inhibits migration and invasion through modulation of transforming growth factor beta-mediated mesenchymal properties in U87 and U251 cells. Drug Des Devel Ther 10:767–779
Carmichael J, DeGraff WG, Gazdar AF, Minna JD, Mitchell JB (1987) Evaluation of a tetrazolium-based semiautomated colorimetric assay: assessment of chemosensitivity testin. Cancer Res 47:936–942
Bedner E, Li X, Gorczyca W, Melamed MR, Darzynkiewicz Z (1999) Analysis of apoptosis by laser scanning cytometry. Cytometry 35:181–195
Dejean LM, Martinez-Caballero S, Manon S, Kinnally KW (2006) Regulation of the mitochondrial apoptosis-induced channel, MAC, by BCL-2 family proteins. BBA-Mol Basis Dis 1762:191–201
Brumatti G, Sheridan C, Martin SJ (2008) Expression and purification of recombinant a nnexin V for the detection of membrane alterations on apoptotic cells. Methods 44:235–240
Lecoeur H, de Oliveira-Pinto LM, Gougeon ML (2002) Multiparametric flow cytometric analysis of biochemical and functional events associated with apoptosis and oncosis using the 7-aminoactinomycin D assay. J Immunol Methods 265:81–96
Korsmeyer SJ, Shutter JR, Veis DJ, Merry DE, Oltvai ZN (1993) Bcl-2/Bax: a rheostat that regulates an anti-oxidant pathway and cell death. Semin Cancer Biol 4:327–332
Pollack M, Phaneuf S, Dirks A, Leeuwenburgh C (2002) The role of apoptosis in the normal aging brain, skeletal muscle, and heart. Ann NY Acad Sci 959:93–107
Sun MG, Williams J, Munoz-Pinedo C, Perkins GA, Brown JM, Ellisman MH, Green DR Frey TG (2007) Correlated three-dimensional light and electron microscopy reveals transformation of mitochondria during apoptosis. Nat Cell Boil 9:1057–1065
Hamel W, Westphal M (2000) Growth factors in gliomas revisited. Acta Neurochir 142:113–138
Nakano A, Tani E, Miyazaki K, Yamamoto Y, Furuyama JI (1995) Matrix metalloproteinases and tissue inhibitors of metalloproteinases in human gliomas. J Neurosurg 83:298–307
Cox G, O’Byrne KJ (2001) Matrix metalloproteinases and cancer. Anticancer Res 21:4207–4219
Mohanam S, Gladson CL, Rao CN, Rao JS (1999) Biological significance of the expression of urokinase-type plasminogen activator receptors (uPARs) in brain tumors. Front Biosci 15:178–187
Saiki S, Sasazawa Y, Imamichi Y, Kawajiri S, Fujimaki T, Tanida I, Kobayashi H, Sato F, Sato S, Ishikawa K, Imoto M, Hattori N (2011) Caffeine induces apoptosis by enhancement of autophagy via PI3K/Akt/mTOR/p70S6K inhibition. Autophagy 7:176–187
Zhang L, Wang H, Zhu J, Xu J, Ding K (2004) Mollugin induces tumor cell apoptosis and autophagy via the PI3K/AKT/mTOR/p70S6K and ERK signaling pathways. Bioch Em Bioph Res Co 450:247–254
Westermarck J, Kahari V-M (1999) Regulation of matrix metalloproteinase expression in tumour invasion. FASEB J 13:781–792
Johnson GL, Lapadat R (2002) Mitogen-activated protein kinase pathways mediated by ERK, JNK, and p38 protein kinases. Science 298:1911–1912
Ahn BH, Min G, Bae YS (2006) Phospholipase D is activated and phosphorylated by casein kinase-II in human U87 astroglioma cells. Exp Mol Med 38:55–62
Camphausen K, Purow B, Sproull M, Scott T, Ozawa T, Deen DF, Tofilon PJ (2005) Orthotopic growth of human glioma cells quantitatively and qualitatively influences radiation-induced changes in gene expression. Cancer Res 65:10389–10393
Nakada M, Niska JA, Miyamori H, McDonough WS, Wu J, Sato H, Berens ME (2004) The phosphorylation of EphB2 receptor regulates migration and invasion of human glioma cells. Cancer Res 64:3179–3185
Sheline GE (1986) Tumors of the brain. Springer: Berlin
Tani E, Morimura T, Kaba K, Itagaki T (1980) Preliminary study of the effects of vitamin A on antineoplastic activities of chemotherapeutic agents in glioma. Neurol Med Chir 20:665–677
Yung WA, Lotan R, Lee P, Lotan D, Steck PA (1989) Modulation of growth and epidermal growth factor receptor activity by retinoic acid in human glioma cells. Cancer Res 49:1014–1019
Gumireddy K, Sutton LN, Phillips PC, Reddy CD (2003) All-trans-retinoic acid-induced apoptosis in human medulloblastoma: activation of caspase-3/poly(ADP-ribose) polymerase 1 pathway. Clin Cancer Res 9:4052–4059
Ying M, Wang S, Sang Y, Sun P, Lal B, Goodwin CR, Guerrero-Cazares H, Quinon s-Hinojosa A, Laterra J, Xia S (2011) Regulation of glioblastoma stem cells by retinoic acid: role for Notch pathway inhibition. Oncogene 30:3454–3467
Liang C, Yang L, Guo S (2015) All-trans retinoic acid inhibits migration, invasion and proliferation, and promotes apoptosis in glioma cells in vitro. Oncol Lett 9:2833–2838
Belloc F, Dumain P, Boisseau MR, Jalloustre C, Reiffers J, Bernard P, Lacombe F (1994) A flow cytometric method using Hoechst 33342 and propidium iodide for simultaneous cell cycle analysis and apoptosis determination in unfixed cells. Cytometry 17:59–65
Rogakou EP, Pilch DR, Orr AH, Ivanova VS, Bonner WM (1998) DNA double-stranded breaks induce histone H2AX phosphorylation on serine 139. J Biol Chem 273:5858–5868
Jeong SY, Seol DW (2008) The role of mitochondria in apoptosis. BMB Rep 41:11–22
Green DR, Reed JC (1998) Mitochondria and apoptosis. Science 281:1309–1312
Saraste A, Pulkki K (2000) Morphologic and biochemical hallmarks of apoptosis. Cardiovasc Res 45:528–537
Jiang H, Shang X, Wu H, Gautam SC, Al-Holou S, Li C, Kuo J, Zhang LJ, Chopp M (2009) Resveratrol downregulates PI3K/Akt/mTOR signaling pathways in human U251 glioma cells. J Exp Ther Oncol 8:25–33
Shen J, Zheng H, Ruan J, Fang W, Li A, Tian G, Niu X, Luo S, Zhao P (2013) Autophagy inhibition induces enhanced proapoptotic effects of ZD6474 in glioblastoma. Brit. J Cancer 109:164–171
Cantley LC (2002) The phosphoinositide 3-kinase pathway. Science 296:1655–1657
Harris T (2003) PDK1 and PKB/Akt: ideal targets for development of new strategies to structure-based drug design. IUBMB Life 55:117–126
Manning BD, Cantley LC (2003) United at last: the tuberous sclerosis complex gene products connect the phosphoinositide 3-kinase/Akt pathway to mammalian target of rapamycin (mTOR) signalling. Biochem Soc T 31:573–578
Gardai SJ, Hildeman DA, Frankel SK, Whitlock BB, Frasch SC, Borregaard N, Marrack P, Bratton DL, Henson PM (2004) Phosphorylation of Bax Ser184 by Akt regulates its activity and apoptosis in neutrophils. J Biol Chem 279:21085–21095
Song G, Ouyang G, Bao S (2005) The activation of Akt/PKB signaling pathway and cell survival. J Cell Mol Med 9:59–71
Fata JE, Ho AV, Leco KJ, Moorehead RA, Khokha R (2000) Cellular turnover and extracellular matrix remodeling in female reproductive tissues: functions of metalloproteinases and their inhibitors. Cell Mol Life Sci 57:77–95
Gacko M (2001) Activation mechanisms, biological role and inhibitors of metalloproteases in the extracellular matrix. Postepy Hig Med Dosw 55:303–318
Curran S, Murray GI (2000) Matrix metalloproteinases: molecular aspects of their roles in tumour invasion and metastasis. Eur J Cancer 36:1621–1630
Deryugina EI, Bourdon MA, Luo GX, Reisfeld RA, Strongin A (1997) Matrix metalloproteinase-2 activation modulates glioma cell migration. J Cell Sci 110:2473–2482
Jung SH, Woo MS, Kim SY, Kim WK, Hyun JW, Kim EJ, Kim DH, Kim HS (2006) Ginseng saponin metabolite suppresses phorbol ester-induced matrix metalloproteinase-9 expression through inhibition of activator protein-1 and mitogen-activated protein kinase signaling pathways in human astroglioma cells. Int J Cancer 118:490–497
Zhang Z, Lv J, Lei X, Li S, Zhang Y, Meng L, Xue R, Li Z (2014) Baicalein reduces the invasion of glioma cells via reducing the activity of p38 signaling pathway. PloS One 9:e90318
Aroui S, Najlaoui F, Chtourou Y, Meunier AC, Laajimi A, Kenani A, Fetoui H (2015) Naringin inhibits the invasion and migration of human glioblastoma cell via downregulation of MMP-2 and MMP-9 expression and inactivation of p38 signaling pathway. Tumor Biol doi:10.1007/s13277-015-4230-4
Acknowledgments
This research was supported by Natural Science Foundation of China (NSFC-81271391).
Author information
Authors and Affiliations
Corresponding author
Ethics declarations
Conflict of Interest
The authors declare no conflict of interest.
Rights and permissions
About this article
Cite this article
Liu, Y., Zheng, J., Zhang, Y. et al. Fucoxanthin Activates Apoptosis via Inhibition of PI3K/Akt/mTOR Pathway and Suppresses Invasion and Migration by Restriction of p38-MMP-2/9 Pathway in Human Glioblastoma Cells. Neurochem Res 41, 2728–2751 (2016). https://doi.org/10.1007/s11064-016-1989-7
Received:
Revised:
Accepted:
Published:
Issue Date:
DOI: https://doi.org/10.1007/s11064-016-1989-7