Abstract
Phosphatase and tension homolog located on chromosome ten (PTEN) is a tumor suppressor as it negatively regulates activation of Akt. Mutation or deletion of PTEN has been found in as high as 80% of glioblastomas, which harbor aberrant cell signaling passing through the phosphatidylinositol-3-kinase (PI3K) and Akt (PI3K/Akt) survival pathway. Glioblastoma cells without functional PTEN are not easily amenable to apoptosis. We investigated the possibility of modulation of signal transduction pathways for induction of apoptosis in human glioblastoma T98G (PTEN-harboring) and U87MG (PTEN-deficient) cell lines after treatment with the combination of all-trans retinoic acid (ATRA) and interferon-gamma (IFN-γ). Treatment with ATRA plus IFN-γ stimulated PTEN expression and suppressed Akt activation in T98G cells, whereas no PTEN expression but Akt activation in U87MG cells under the same conditions. Pretreatment of U87MG cells with the PI3K inhibitor LY294002 could prevent Akt activation. Interestingly, ATRA plus IFN-γ could significantly decrease cell viability and increase morphological features of apoptosis in both cell lines. Combination of ATRA and IFN-γ showed more efficacy than IFN-γ alone in causing apoptosis that occurred due to increases in Bax:Bcl-2 ratio, mitochondrial release of cytochrome c, and caspase-3 activity. Luciferase reporter gene assay showed that combination of ATRA and IFN-γ significantly down regulated transcriptional activity of the nuclear factor kappa B (NF-κB), a survival signaling factor, in U87MG cells. Thus, combination of ATRA and IFN-γ caused significant amounts of apoptosis in T98G cells due to suppression of the PI3K/Akt survival pathway while the same treatment caused apoptosis in U87MG cells due to down regulation of the NF-κB activity. Therefore, the combination of ATRA and IFN-γ could modulate different survival signal transduction pathways for induction of apoptosis and should be considered as an effective therapeutic strategy for controlling the growth of both PTEN-harboring and PTEN-deficient glioblastomas.
Similar content being viewed by others
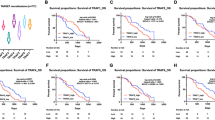
Avoid common mistakes on your manuscript.
Introduction
Glioblastoma is the most aggressive and malignant brain tumor in humans [14]. The patients survive less than 12 months after diagnosis of glioblastoma [28]. Due to inability of the synthetic cytotoxic medicines to cross the blood-brain-barrier and also their severe side effects, chemotherapy with the synthetic agents could not yet dramatically improve the median survival of the glioblastoma patients [28, 29, 31]. Nowadays, research is substantially focused on chemotherapy with the natural cytostatic medicines [12, 20] such as retinoids that can control growth of cancer cells through promoting differentiation and inhibiting angiogenesis, tumor invasion, and growth factor pathways. All-trans retinoic acid (ATRA) exerts growth suppression in leukemia [2, 9], breast cancer [26], prostate cancer [21], and ovarian cancer [27]. It alone or in combination with another natural agent such as interferon-gamma (IFN-γ) controls the growth of cancer cells due to upregulation of Bax, down regulation of Bcl-2, and increase in caspase 3 activity [10, 26].
The phosphatase and tension homolog located on chromosome ten (PTEN), which encodes a cytoplasmic phosphatase with both protein and lipid phosphatase activity, is frequently mutated or deleted at chromosome 10q23 in glioblastomas [18]. As PTEN serves as a tumor suppressor, deficiency or loss of its function encourages cell proliferation in many cancers such as breast cancer, prostate cancer, melanoma, and brain tumors [6]. Mutation or deletion of PTEN may occur in as high as 80% of human glioblastomas. PTEN dephosphorylates phosphatidylinositol-3-kinase (PI3K), which otherwise causes phosphorylation of protein kinase B (PKB), also known as Akt (short for ‘acutely transforming retrovirus Akt-8’), to promote tumor cell survival and proliferation [15, 16, 19, 24]. Transfection of wild-type PTEN cDNA into the glioblastoma cell lines containing mutated or deleted PTEN could induce cell cycle arrest at the G1 phase and elicit astrocytic differentiation [1]. The inhibition of the PI3K/Akt survival signaling could be demonstrated in glioblastoma cells harboring intact and functional PTEN [30]. The decreased PTEN level and increased Akt activity have been associated with more aggressive tumor behavior and reduced survival time in glioblastoma patients [7].
There has not yet been any specific report for the modulatory effects of ATRA plus IFN-γ on survival signal transduction pathways in PTEN-harboring and PTEN-deficient glioblastoma cell lines. We designed this investigation to examine the suppression of the survival pathways for mediation of apoptosis in glioblastoma T98G (PTEN-harboring) and U87MG (PTEN-deficient) cells after treatment with ATRA plus IFN-γ. Our data showed that combination of ATRA and IFN-γ induced apoptosis in these two cell lines due to suppression of two different survival pathways.
Materials and methods
Materials
Human glioblastoma cell lines T98G and U87MG were purchased from the American Type Culture Collection (ATCC, Manasas, MD, USA). ATRA and human recombinant IFN-γ were purchased from Sigma Chemical (St. Louis, MO, USA). Antibodies against PTEN and phospho-Akt and the PI3K inhibitor 2-(4-morpholinyl)-8-phenylchromone (LY294002, an Eli Lilly inhibitor) were bought from Cell Signaling Technologies (Danvers, MA, USA). Cytochrome c antibody was obtained from BD Biosciences (San Jose, CA, USA). Bax and Bcl-2 antibodies were purchased from Santa Cruz Biotechnology (Santa Cruz, CA, USA). Monoclonal α-spectrin antibody was received from Affiniti (Exeter, UK). The plasmid vector pNF-κB-luc was bought from Clontech (Mountain View, CA, USA). Lipofectamine-2000 transfection reagent was obtained from Invitrogen (Carlsbad, CA, USA). Luciferase reporter gene assay kit was purchased from Promega (Madison, WI, USA).
Cell culture
Human glioblastoma cell lines T98G and U87MG were grown in 1xRPMI 1640 medium containing 10% fetal bovine serum (FBS) and 1% penicillin and streptomycin. Cells were seeded in 75-cm2 flasks and incubated at 37°C in a humidified atmosphere with 5% CO2. Cells with about 75% confluency were starved in 1xRPMI 1640 with 0.5% FBS for 6 h and kept in this low-serum medium during all the treatments. Then cells were treated with 5 μM ATRA alone, 5 ng/ml IFN-γ alone, and 5 μM ATRA plus 5 ng/ml IFN-γ for 18 h. For a time-course, treatments were also carried out for different time points: 0.5, 1, 2, 3, 5, and 18 h. After all the treatments, the cells were harvested for assessment of apoptosis and analysis of specific pathways involved.
Determination of residual cell viability
After treatment with ATRA and IFN-γ alone and in combination, the trypan blue dye exclusion test was performed to evaluate the residual cell viability [23]. Viable cells maintained membrane integrity and did not take up trypan blue. Cells with compromised cell membranes took up trypan blue and were counted as dead. At least 600 cells were counted in four different fields and the number of residual viable cells was calculated as percentage of the total cell population.
Wright staining for morphological features of apoptosis
The cells from all treatments were harvested and washed in PBS, pH 7.4, and sedimented onto the microscopic slides using the Centra CL2 centrifuge (IEC, Needham Heights, MA, USA) at 1,000 rpm for 5 min. Cells were fixed and then stained with Wright stain, as we reported previously [4]. Cellular morphology was examined by optical microscopy to assess apoptosis. Cells with the characteristic of reduction in cell volume, condensation of the chromatin, and/or the presence of cell membrane blebbing, were considered apoptotic. At least 600 cells were counted in each treatment and the percentage of apoptotic cells was calculated.
Protein extraction and Western blotting
After the treatments, cells were lyzed in a buffer composed of 50 mM Tris–HCl, pH 7.4, 0.1 mM phenylmethylsulfonyl fluoride (PMSF), and 5 mM EGTA for extraction of cellular proteins. Concentration of total proteins was determined colorimetrically using Coomassie-Plus protein assay reagent (Pierce, Rockford, IL, USA). The samples were mixed with an equal volume of 2 × loading buffer [125 mM Tris–HCl, pH 6.8, 4% SDS, 20% glycerol, 200 mM 1,4-dithio-dl-threitol (DTT), and 0.02% bromophenol blue], boiled for 5 min, and loaded (40 μg/lane) onto the 4–20% gradient gels for the sodium dodecyl sulfate-polyacrylamide gel electrophoresis (SDS-PAGE). After SDS-PAGE, the gels were blotted to Immunobilon-P nylon membrane. The blots were blocked in 5% non-fat milk, 0.1% Tween, Tris–HCl, pH 7.8, for 2 h at room temperature. Then the blots were incubated with a specific primary IgG antibody for 2 h at room temperature or overnight at cold room followed by alkaline horseradish peroxidase-conjugated secondary IgG antibody for 1 h. Blots were developed using the enhanced chemiluminescence (ECL) or ECL-Plus reagents (Amersham Pharmacia, Buckinghamshire, UK). The ECL autoradiograms were scanned on a PowerLook Scanner (Umax Technologies, Fremont, CA, USA) using Photoshop software (Adobe Systems, Seattle, WA, USA) and optical density (OD) of each band was determined using Quantity One software (Bio-Rad, Hercules, CA, USA).
Western blotting for determination of mitochondrial release of cytochrome c into cytosol
For determining any mitochondrial release of cytochrome c into cytosol after the treatments, we isolated mitochondrial and cytosolic fractions. Briefly, cells from each treatment were harvested, washed once with ice-cold PBS, and gently lyzed for 1 min in ice-cold lysis buffer (250 mM sucrose, 1 mM EDTA, 0.05% digitonin, 25 mM Tris–HCl, pH 6.8, 1 mM DTT, 1 μg/ml leupeptin, 1 μg/ml pepstatin, 1 μg/ml aprotinin, 1 mM benzamidine, and 0.1 mM PMSF). Lysates were centrifuged at 12,000 × g at 4°C for 3 min to obtain the pellet (fraction containing mitochondria) and the supernatant (fraction containing cytosolic extract without mitochondria). Both the mitochondrial and cytosolic fractions were analyzed by Western blotting using an antibody against cytochrome c.
Transfection of pNF-κB-luc and luciferase reporter gene assay for NF-κB activity
We transfected the plasmid vector pNF-κB-luc (Clontech, Mountain View, CA, USA) into T98G and U87MG cells for monitoring the activation or inactivation of NF-κB survival signal transduction pathway due to treatment with ATRA plus IFN-γ. The plasmid vector pNF-κB-luc (5.0 kb) contains a region (27–66) of four tandem repeats of the NF-κB binding sequence (GGGAATTTCCx4) fused to a region (73–221) of TATA-like promoter from the herpes simplex virus-thymidine kinase (HSV-TK) promoter to drive the transcription of the region (223–1912) of the firefly luciferase (luc) reporter gene from Photinus pyralis. Binding of intracellular NF-κB to its consensus DNA sequence on the vector induces transcription of the luciferase reporter gene. One day prior to transfection of the vector, the cells were subcultured to 90% confluency. Cells were transfected with pNF-κB-luc using Lipofectamine-2000 transfection reagent (Invitrogen, Carlsbad, CA, USA) in 1 × RMPI 1640 containing 0.5% FBS for 24 h. The transfected cells were treated with ATRA plus IFN-γ for 5 h and lyzed by addition of 100 μl of lysis buffer and sonication. Then, 20 μl aliquot was mixed with 100 μl of assay reagent and vortexed just before reading fluorescence in a luminometer.
Statistical analysis
Results were analyzed using StatView software (Abacus Concepts, Berkeley, CA, USA) and compared using one-way analysis of variance (ANOVA) with Fisher’s post hoc test. Data were presented as mean ± standard error of mean (SEM) of separate experiments (n ≥ 3). Significant difference from control value was indicated by * (p < 0.05) or ** (p < 0.001).
Results
Changes in expression of PTEN in glioblastoma cells after the treatments
We used Western blotting to detect the changes in expression of the tumor suppressor PTEN in both T98G and U87MG cell lines following the treatments. The expression of 55 kD PTEN was detected in T98G cells (Fig. 1) but not in U87MG cells (data not shown). The uniform expression of 42 kD β-actin was used as a loading control on the Western blots (Fig. 1). Compared with control T98G cells, treatment (18 h) with ATRA alone did not change the level of PTEN expression while treatment (18 h) with IFN-γ alone significantly (p < 0.001) induced PTEN expression (Fig. 1a). The significant induction of PTEN expression remained unaffected when the cells were treated ATRA plus IFN-γ for 18 h (Fig. 1a). Pretreatment (1 h) of the cells with the PI3K inhibitor LY294002 did not affect the significant induction of PTEN expression by combination of ATRA and IFN-γ. Further, we investigated the time-course of induction of PTEN in T98G cells after treatment with the combination of ATRA and IFN-γ for seven different time points (Fig. 1b). Results indicated that treatment of T98G cells with combination of ATRA and IFN-γ induced PTEN expression time-dependently and significant (p < 0.05) induction occurred in 2 h, reached peak level at 5 h, and maintained peak level even at 18 h (Fig. 1b). So, we decided to treat the cells for 18 h in subsequent experiments for assessment of apoptosis. Induction of PTEN expression could suppress the PI3K mediated activation of the oncoprotein Akt so as to facilitate the occurrence of apoptosis in glioblastoma cells after treatment with combination of ATRA and IFN-γ.
Western blotting to examine the levels of PTEN in glioblastoma cells. (a) Levels of 55 kD PTEN expression in T98G cells. Treatments (18 h): control, 5 μM ATRA, 5 ng/ml IFN-γ, 5 μM ATRA + 5 ng/ml IFN-γ, and 20 μM LY294002 (1-h pretreat) + 5 μM ATRA + 5 ng/ml IFN-γ. Western blots were developed using the ECL reagent. (b) Time-dependent increase in levels of 55 kD PTEN expression in T98G cells following treatment with ATRA plus IFN-γ. Western blots were developed using the ECL-Plus reagent
Time-course for changes in activation of Akt in glioblastoma cells after treatment with combination of ATRA and IFN-γ
Activation of Akt occurs due to its phosphorylation by PI3K activity. We performed Western blotting to examine the changes in levels of activation of Akt (i.e., phosphorylation of Akt or phospho-Akt) and total Akt in glioblastoma cells after treatment with combination of ATRA and IFN-γ for different time-points (Fig. 2). Almost uniform levels of expression of 42 kD β-actin in both T98G and U87MG cell lines were used as loading controls on the Western blots (Fig. 2). Western blotting with an antibody capable of specifically detecting phosphorylation of Akt at Ser-473 showed no phosphorylation of Akt in T98G cells (Fig. 2a). Thus, activation of PI3K/Akt survival pathway did not occur in T98G cells and total Akt level remained almost unaltered after the treatment of cells with combination of ATRA and IFN-γ (Fig. 2a). In contrast, treatment with combination of ATRA and IFN-γ continued to increase 60 kD phospho-Akt level in U87MG cells during early time-points (0.5–5 h) but tended to decrease its level at 18 h (Fig. 2b). Pretreatment of U87MG cells with the PI3K inhibitor LY294002 could prevent activation of Akt, indicating the involvement of PI3K activity in this process. The total Akt level was not affected in U87MG cells after the treatments.
Western blotting to examine the activation of Akt (formation of phospho-Akt) in glioblastoma cells. Treatments (18 h): 5 μM ATRA + 5 ng/ml IFN-γ, and 20 μM LY294002 (1-h pretreat) + 5 μM ATRA + 5 ng/ml IFN-γ. Western blots were developed using the ECL reagent. (a) Time-course of formation of any phospho-Akt and also level of total Akt in T98G cells. Activation of Akt was not detected in T98G cells. (b) Time-course of formation of phospho-Akt and also level of total Akt in U87MG cells. Activation of Akt occurred increasingly to some extent up to 5 h and tended to decrease at 18 h. Pretreatment of cells with the PI3K inhibitor LY294002 prevented activation of Akt, indicating a role for PI3K in this process
Decrease in residual cell viability and increase in apoptosis in glioblastoma cells after the treatments
We determined the amounts of residual cell viability and apoptosis in both T98G and U87MG cell lines after the treatment with ATRA and IFN-γ alone and in combination (Fig. 3). Treatment with ATRA alone did not significantly alter residual cell viability, IFN-γ alone significantly decreased (p < 0.05) residual cell viability, and the combination of ATRA and IFN-γ caused the highest decrease (p < 0.001) in residual cell viability in both cell lines (Fig. 3a). The results suggested that combination of ATRA and IFN-γ could work synergistically to cause cell death in both T98G and U87MG cells.
Decrease in cell viability and increase in apoptosis in glioblastoma cells following different treatments. Treatments (18 h): control, 5 μM ATRA, 5 ng/ml IFN-γ, and 5 μM ATRA + 5 ng/ml IFN-γ. (a) Determination of amounts of residual cell viability based on trypan blue dye exclusion test. (b) Wright staining to examine morphological features of apoptosis. (c) Determination of amounts of apoptosis based on morphological features
Wright staining was used to evaluate the morphologic features of apoptosis in T98G and U87MG cells after the treatments (Fig. 3b). Apoptosis with characteristic morphologic changes such as cell shrinkage, membrane blebbing, chromatin condensation, and/or formation of membrane-bound apoptotic bodies occurred to some extent after treatment with IFN-γ alone but mostly after treatment with the combination of ATRA and IFN-γ in both cell lines (Fig. 3b). The apoptotic cells were counted to determine the percentage of apoptosis (Fig. 3c). Compared with the control cells, treatment with combination of ATRA and IFN-γ caused apoptosis most effectively (p < 0.001) in both cell lines, regardless of their different PTEN contents. In subsequent experiments, we examined the molecular basis of occurrence of apoptosis in T98G and U87MG cells.
Apoptosis occurred with increase in Bax:Bcl-2 ratio
Western blotting was performed to examine the changes in levels of expression of pro-apoptotic Bax and anti-apoptotic Bcl-2 proteins in course of apoptosis in both T98G and U87MG cell lines after the treatments (Fig. 4). Treatment with IFN-γ alone and combination of ATRA and IFN-γ increased the levels of Bax expression and decreased the levels of Bcl-2 expression in both glioblastoma cell lines (Fig. 4a). We performed densitometric analysis to quantitate the levels of expression of Bax and Bcl-2 to determine the alterations in Bax:Bcl-2 ratio (Fig. 4b). Compared with control cells, treatment with IFN-γ alone and combination of ATRA and IFN-γ caused significant (p < 0.001) increase in the Bax:Bcl-2 ratio in both cell lines (Fig. 4b), suggesting a commitment of the cells to apoptosis via mitochondria-dependent pathway.
Western blotting to examine alterations in levels of Bax and Bcl-2 proteins in glioblastoma cells. Treatments (18 h): control, 5 μM ATRA, 5 ng/ml IFN-γ, and 5 μM ATRA + 5 ng/ml IFN-γ. (a) Representative Western blots show levels of Bax, Bcl-2, and β-actin in T98G and U87MG cells following treatments. Western blots were developed using the ECL-Plus reagent. (b) Determination of Bax:Bcl-2 ratio in T98G and U87MG cells. An increase in Bax:Bcl-2 following a treatment indicated a commitment of cells to apoptosis
Mitochondrial release of cytochrome c into the cytosol and activation of caspase-3
An increase in Bax:Bcl-2 ratio is known to promote mitochondrial release of cytochrome c into the cytosol for activation of cysteine proteases for apoptosis. We separated the mitochondrial and cytosolic fractions for Western blotting to assess mitochondrial release of cytochrome c into the cytosol (Fig. 5). Treatment of T98G and U87MG with IFN-γ alone or combination of ATRA and IFN-γ triggered mitochondrial release of cytochrome c into the cytosol (Fig. 5a). Compared with control cells, significant (p < 0.001) decrease in cytochrome c level in mitochondria occurred in T98G cells after treatment with IFN-γ alone or combination of ATRA and IFN-γ but in U87MG cells only after treatment with combination of ATRA and IFN-γ (Fig. 5b). Analysis of cytosolic fractions showed concurrent significant (p < 0.001) increase in cytochrome c level in the cytosol in both T98G and U87MG cells after treatment with IFN-γ alone or combination of ATRA and IFN-γ (Fig. 5c), indicating that mitochondrial release of cytochrome c into the cytosol could promote apoptotic process. Notably, the mitochondrial release of cytochrome c into the cytosol was more after treatment with the combination of ATRA and IFN-γ than after treatment with IFN-γ alone. Cytosolic cytochrome c helps activation of caspase-9 that in turn activates caspase-3. Further, we performed Western blotting to assess caspase-3 activity in the cleavage of 45 kD inhibitor of caspase-3-activated DNase (ICAD) to 40 kD ICAD fragment (Fig. 6). Treatment of T98G and U87MG cells with the therapeutic agents caused ICAD cleavage (Fig. 6a). Compared with control cells, treatment with ATRA and IFN-γ alone or in combination significantly generated 40 kD ICAD fragment in both T98G and U87MG cells (Fig. 6b). Since ICAD is a molecular chaperone of CAD, the fragmentation of ICAD may release and translocate CAD to the nucleus for apoptotic DNA fragmentation.
Western blotting to examine mitochondrial release of cytochrome c into the cytosol in glioblastoma T98G and U87MG cells. Treatments (18 h): control, 5 μM ATRA, 5 ng/ml IFN-γ, and 5 μM ATRA + 5 ng/ml IFN-γ. (a) Representative Western blots show levels of mitochondrial cytochrome c and cytosolic cytochrome c and β-actin following treatments. Western blots were developed using the ECL-Plus reagent. (b) Determination of percent decrease in mitochondrial cytochrome c level. (c) Determination of percent increase in cytosolic cytochrome c level. Treatment of cells with IFN-γ alone or ATRA + IFN-γ caused mitochondrial release of cytochrome c into the cytosol
Western blotting to examine caspase-3 activity in the formation of ICAD fragment in glioblastoma T98G and U87MG cells. Treatments (18 h): control, 5 μM ATRA, 5 ng/ml IFN-γ, and 5 μM ATRA + 5 ng/ml IFN-γ. (a) Representative Western blots show levels of 40 kD ICAD fragment following treatments. Western blots were developed using the ECL-Plus reagent. (b) Determination of percent increase in ICAD fragment. Treatment of cells with ATRA and IFN-γ alone or in combination caused formation of ICAD fragment. Combination of ATRA and IFN-γ was the most effective in increasing ICAD fragment, indicating the highest increase in caspase-3 activity
Luciferase reporter gene assay to determine activity of NF-κB after combination therapy
The activation of the pro-survival transcription factor NF-κB could lead to inhibition of apoptosis. We used luciferase reporter gene assay to measure any changes in transcriptional activity of NF-κB in both T98G and U87MG cells after treatment with the combination of ATRA and IFN-γ (Fig. 7). The glioblastoma cells were transfected with the NF-κB plasmid containing an insertion of luciferase reporter gene and also with the control plasmid. Then cells were treated with the combination of ATRA and IFN-γ. Luciferase reporter gene activities were measured to assess any activity of NF-κB. The results showed that activity of NF-κB was very low in T98G cells and treatment with combination of ATRA and IFN-γ did not significantly decrease activity of NF-κB in T98G cells. However, treatment with combination of ATRA and IFN-γ very significantly (p < 0.001) decreased activity of NF-κB in U87MG cells, so as to allow them to succumb to apoptosis.
Luciferase reporter gene assay for determination of transcriptional activity of NF-κB in glioblastoma T98G and U87MG cells. Glioblastoma cells were transfected with the plasmid vector pNF-κB-luc prior to treatments. Treatments (18 h): control, and 5 μM ATRA + 5 ng/ml IFN-γ. A decrease in luciferase reporter gene activity following treatment indicated a decrease in NF-κB activity in glioblastoma cells
Discussion
The mutation or deletion of the tumor suppressor PTEN has been found in many tumors including glioblastoma. The inactivation of PTEN plays a critical role in the progression of glioblastoma. Transfection of cancer cells with a plasmid expressing wild-type PTEN could result in cell cycle arrest in vitro and reduced tumorigenicity in vivo [8, 11]. Because human glioblastoma T98G is a PTEN-harboring cell line, it is amenable to many chemotherapeutic agents. In contrast, human glioblastoma U87MG is a PTEN-deficient cell line and thus remains highly resistant to chemotherapy. Our recent study showed that combination of ATRA and IFN-γ effectively could control malignant growth of these two cell lines [10]. In the current study, we examined any changes in PTEN/PI3K/Akt and NF-κB signaling mechanisms in these two glioblastoma cell lines after treatment with combination of ATRA and IFN-γ. This is the first report demonstrating that combination of ATRA and IFN-γ enhanced apoptosis in T98G cells due to upregulation of PTEN for suppression of the PI3K/Akt survival pathway while in U87MG cells due to suppression of the NF-κB survival signaling.
It is known that upregulation of PTEN plays an important role in signal transduction pathway because it then inhibits activation of PI3K and thus prohibits further activation of Akt in cancers including glioblastoma [1, 16, 30]. Our current data showed that treatment of T98G cells with combination of ATRA and IFN-γ increased PTEN expression in a time-dependent manner (Fig. 1). The presence of PTEN and its upregulation following treatment with combination of ATRA and IFN-γ could abolish activation of Akt (i.e., formation of phospho-Akt) in T98G cells (Figs. 1, 2) to make them prone to apoptosis. On the other hand, deficiency of PTEN provided an advantage to PI3K for formation of phospho-Akt in U87MG cells even after treatment of the cells with combination of ATRA and IFN-γ; however, this process could partially be prevented by pretreatment of the cells with the PI3K inhibitor LY294002 (Fig. 2). In fact, the activation of PI3K/Akt survival pathway reflected the deficiency or absence of PTEN in U87MG cells. Because treatment with combination of ATRA and IFN-γ significantly decreased cell viability and increased morphologic features of apoptosis not only in T98G cells but also in U87MG cells though to a lesser extent (Fig. 3), we realized that another important survival signaling mechanism was severely impaired in U87MG cells after the combination therapy.
We observed that treatment with IFN-γ alone or combination of ATRA and IFN-γ caused apoptosis in both T98G and U87MG cells due to significant increases in Bax:Bcl-2 ratio (Fig. 4). These results are similar to the previous studies in leukemia [9], glioblastoma [32], and also in prostate cancer [22] cells. Because Bax overexpression is associated with mitochondrial release of cytochrome c [3, 25], we also examined the mitochondrial release of cytochrome c (Fig. 5). Apoptotic stimuli with IFN-γ alone and combination of ATRA and IFN-γ triggered mitochondrial release of cytochrome c into the cytosol in both T98G and U87MG cells (Fig. 5). Cytosolic cytochrome c eventually signals to increase the caspase-3 activity in the final phase of apoptosis [5]. We examined any increase in caspase-3 activity in the generation of ICAD fragment (Fig. 6). Treatment with combination of ATRA and IFN-γ produced more ICAD fragment than treatment with IFN-γ alone (Fig. 6), indicating the greater efficacy of the combination therapy for apoptotic execution of both T98G and U87MG cells.
The specific inhibitor of PI3K prevents growth of glioblastoma [25] and also PTEN suppresses the PI3K/Akt survival pathway in tumors [3]. Because PTEN was upregulated in T98G cells following treatment with the combination of ATRA and IFN-γ, induction of apoptosis occurred in T98G cells due to suppression of the PI3K/Akt survival pathway. However, we also wanted to explore the suppression of any other survival signaling mechanism by combination of ATRA and IFN-γ in PTEN-deficient U87MG cells for induction of apoptosis. It has been reported previously that NF-κB plays an important role as an anti-apoptotic transcription factor [13, 17]. Therefore, any decrease in transcriptional activity of NF-κB following a therapeutic treatment would make the cells prone to apoptosis. Our current data from the luciferase reporter gene assay indicated significant decrease in the transcriptional activity of NF-κB in U87MG cells following treatment with combination of ATRA and IFN-γ (Fig. 7). This finding suggested that combination of ATRA and IFN-γ activated apoptotic process in U87MG through down regulation of NF-κB survival signaling.
In conclusion, this investigation for the first time demonstrated that combination of ATRA and IFN-γ could suppress the PI3K/Akt survival pathway in PTEN-harboring T98G cells whereas the NF-κB survival signaling in PTEN-deficient U87MG cells for induction of significant amounts of apoptosis in both human glioblastoma cell lines. Therefore, this combination therapy can further be explored as an effective therapeutic strategy for controlling the malignant growth of not only PTEN-harboring but also PTEN-deficient glioblastoma in preclinical animal models as well as in clinical settings.
References
Adachi J, Ohbayashi K, Suzuki T, Sasaki T (1999) Cell cycle arrest and astrocytic differentiation resulting from PTEN expression in glioma cells. J Neurosurg 91:822–830
Asou N (2007) All-trans retinoic acid in the treatment of acute promyelocytic leukemia. Intern Med 46:91–93
Cantley LC, Neel BG (1999) New insights into tumor suppression: PTEN suppresses tumor formation by restraining the phosphoinositide 3-kinase/Akt pathway. Proc Natl Acad Sci USA 96:4240–4245
Das A, Banik NL, Patel SJ, Ray SK (2004) Dexamethasone protected human glioblastoma U87MG cells from temozolomide induced apoptosis by maintaining Bax:Bcl-2 ratio and preventing proteolytic activities. Mol Cancer 3:1–10
Das A, Banik NL, Ray SK (2006) Mechanism of apoptosis with the involvement of calpain and caspase cascades in human malignant neuroblastoma SH-SY5Y cells exposed to flavonoids. Int J Cancer 119:2575–2585
Eng C (2003) PTEN: one gene, many syndromes. Hum Mutat 22:183–198
Ermoian RP, Furniss CS, Lamborn KR, Basila D, Berger MS, Gottschalk R, Nicholas MK, Stokoe D, Haas-Kogan DA (2002) Dysregulation of PTEN and protein kinase B is associated with glioma histology and patient survival. Clin Cancer Res 8:1100–1106
Fan X, Aalto Y, Sanko SG, Knuutila S, Klatzmann D, Castresana JS (2002) Genetic profile, PTEN mutation and therapeutic role of PTEN in glioblastomas. Int J Oncol 21:1141–1150
Garzon R, Pichiorri F, Palumbo T, Visentini M, Aqeilan R, Cimmino A, Wang H, Sun H, Volinia S, Alder H, Calin GA, Liu CG, Andreeff M, Croce CM (2007) MicroRNA gene expression during retinoic acid-induced differentiation of human acute promyelocytic leukemia. Oncogene 29:1–10
Haque A, Das A, Hajiaghamohseni LM, Younger A, Banik NL, Ray SK (2007) Induction of apoptosis and immune response by all-trans retinoic acid plus interferon-gamma in human malignant glioblastoma T98G and U87MG cells. Cancer Immunol Immunother 56:615–625
Hwang PH, Yi HK, Kim DS, Nam SY, Kim JS, Lee DY (2001) Suppression of tumorigenicity and metastasis in B16F10 cells by PTEN/MMAC1/TEP1 gene. Cancer Lett 172:83–91
Jendrossek V, Belka C, Bamberg M (2003) Novel chemotherapeutic agents for the treatment of glioblastoma multiforme. Expert Opin Investig Drugs 12:1899–1924
Karin M, Lin A (2002) NF-κB at the crossroads of life and death. Nat Immunol 3:221–227
Kleihues P, Louis DN, Scheithauer BW, Rorke LB, Reifenberger G, Burger PC, Cavenee WK (2002) The WHO classification of tumors of the nervous system. J Neuropathol Exp Neurol 61:215–225
Koul D, Shen R, Bergh S, Sheng X, Shishodia S, Lafortune TA, Lu Y, de Groot F, Mills GB, Yung WK (2006) Inhibition of Akt survival pathway by a small-molecule inhibitor in human glioblastoma. Mol Cancer Ther 5:637–644
Kurose K, Zhou XP, Araki T, Cannistra SA, Maher ER, Eng C (2001) Frequent loss of PTEN expression is linked to elevated phosphorylated Akt levels, but not associated with p27 and cyclin D1 expression, in primary epithelial ovarian carcinomas. Am J Pathol 158:1895–1898
Lee R, Collins T (2001) NFκB and cell survival IAPs call for support. Circ Res 88:262–264
Li J, Yen C, Liaw D, Podsypanina K, Bose S, Wang SI, Puc J, Miliaresis C, Rodgers L, McCombie R, Bigner SH, Giovanella BC, Ittmann M, Tycko B, Hibshoosh H, Wigler MH, Parsons R (1997) PTEN, a putative protein tyrosine phosphatase gene mutated in human brain, breast, and prostate cancer. Science 275:1943–1947
Maier D, Jones G, Li X, Schonthal AH, Gratzl O, Van Meir EG, Merlo A (1999) The PTEN lipid phosphatase domain is not required to inhibit invasion of glioma cells. Cancer Res 59:5479–5482
Parney IF, Chang SM (2003) Current chemotherapy for glioblastoma. Cancer J 9:149–156
Pasquali D, Chieffi P, Deery WJ, Nicoletti G, Bellastella A, Sinisi AA (2005) Differential effects of all-trans retinoic acid (RA) on Erk1/2 phosphorylation and cAMP accumulation in normal and malignant human prostate epithelial cells: Erk1/2 inhibition restores RA-induced decrease of cell growth in malignant prostate cells. Eur J Endocrinol 152:663–669
Pasquali D, Rossi A, Prezioso D, Gentile V, Colantuoni V, Lotti T, Bellastella A, Sinisi AA (1999) Change in tissue transglutaminase activity and expression during retinoic acid-induced growth arrest and apoptosis in primary cultures of human epithelial prostate cells. J Clin Endocrinol Metab 84:1463–1469
Ray SK, Wilford GG, Crosby CV, Hogan EL, Banik NL (1999) Diverse stimuli induce calpain overexpression and apoptosis in C6 glioma cells. Brain Res 829:18–27
Rong Y, Post DE, Pieper RO, Durden DL, Van Meir EG, Brat DJ (2005) PTEN and hypoxia regulate tissue factor expression and plasma coagulation by glioblastoma. Cancer Res 65:1406–1413
Shingu T, Yamada K, Hara N, Moritake K, Osago H, Terashima M, Uemura T, Yamasaki T, Tsuchiya M (2003) Growth inhibition of human malignant glioma cells induced by the PI3K-specific inhibitor. J Neurosurg 98:154–161
Simeone AM, Tari AM (2004) How retinoids regulate breast cancer cell proliferation and apoptosis. Cell Mol Life Sci 61:1475–1484
Soprano KJ, Purev E, Vuocolo S, Soprano DR (2006) Rb2/p130 and protein phosphatase 2A: key mediators of ovarian carcinoma cell growth suppression by all-trans retinoic acid. Oncogene 25:5315–5325
Stewart LA (2002) Chemotherapy in adult high-grade glioma: a systemic review and meta-analysis of individual patient data from 12 random trials. Lancet 359:1011–1018
Stupp R, Mason WP, van den Bent MJ, Weller M, Fisher B, Taphoorn MJ, Belanger K, Brandes AA, Marosi C, Bogdahn U, Curschmann J, Janzer RC, Ludwin SK, Gorlia T, Allgeier A, Lacombe D, Cairncross JG, Eisenhauer E, Mirimanoff RO, European Organisation for Research, Treatment of Cancer Brain Tumor and Radiotherapy Groups, National Cancer Institute of Canada Clinical Trials Group (2005) Radiodynamic therapy plus concomitant and adjuvant temozolomide for glioblastoma. N Eng J Med 352:987–996
Wang MY, Lu KV, Zhu S, Dia EQ, Vivanco I, Shackleford GM, Cavenee WK, Mellinghoff IK, Cloughesy TF, Sawyers CL, Mischel PS (2006) Mammalian target of rapamycin inhibition promotes response to epidermal growth factor receptor kinase inhibitors in PTEN-deficient and PTEN-intact glioblastoma cells. Cancer Res 66:7864–7869
Wong ML, Kaye AH, Hovens AM (2007) Targeting malignant glioma survival signalling to improve clinical outcomes. J Clin Neurosci 14:301–308
Zang C, Wachter M, Liu H, Posch MG, Fenner MH, Stadelmann C, von Deimling A, Possinger K, Black KL, Koeffler HP, Elstner E (2003) Ligands for PPARγ and RAR cause induction of growth inhibition and apoptosis in human glioblastomas. J Neurooncol 65:107–118
Acknowledgments
This work was supported in part by the R01 (CA-91460 and NS-57811) grants from the National Institutes of Health (Bethesda, MD, USA) and also by a Spinal Cord Injury Research Fund (SCIRF-0803) grant from the State of South Carolina to S.K.R.
Author information
Authors and Affiliations
Corresponding author
Additional information
Special issue in honor of Naren Banik.
Rights and permissions
About this article
Cite this article
Zhang, R., Banik, N.L. & Ray, S.K. Combination of All-trans Retinoic Acid and Interferon-gamma Suppressed PI3K/Akt Survival Pathway in Glioblastoma T98G Cells whereas NF-κB Survival Signaling in Glioblastoma U87MG Cells for Induction of Apoptosis. Neurochem Res 32, 2194–2202 (2007). https://doi.org/10.1007/s11064-007-9417-7
Received:
Accepted:
Published:
Issue Date:
DOI: https://doi.org/10.1007/s11064-007-9417-7