Abstract
Denture stomatitis is a common inflammatory disorder of the palatal mucosa amongst denture wearers. The pathological changes are induced by Candida albicans biofilm on the fitting surface of the upper denture, and different individuals experience different levels of disease. C. albicans is known to produce secreted aspartyl proteinases (SAPs) to aid adhesion, invasion and tissue destruction. We hypothesised that differential expression and activity of SAPs from denture stomatitis isolates results in different levels of disease amongst denture wearers. We selected C. albicans isolates from asymptomatic controls and three different severities of disease [Newton’s type (NT) 0, I, II and III]. We assessed biofilm formation and proteinase activity for each biofilm and investigated the transcriptional profile of SAPs 1, 2, 5, 6 and 8 from early (12 h) and mature (24 h) biofilms. There were no significant differences between isolates with respect to biofilm formation, whereas proteinase activity normalised to biofilm growth was significantly increased in the diseased groups (p < 0.0001). Proteinase activity correlated strongly with SAP expression (p < 0.0001). SAP8 expression was the greatest, followed by SAP5, 6, 2 and 1. The diseased groups showed the greatest levels of SAP expression, with significant differences also observed between the groups (p < 0.005). All SAPs except SAP5 were expressed in greater amounts in the mature biofilms compared to early biofilms. Overall, this study suggests that SAP activity in biofilms determined in vitro may help to explain differences in disease severity. SAP8 has been shown for the first time to play a prominent role in biofilms.
Similar content being viewed by others
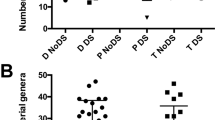
Avoid common mistakes on your manuscript.
Introduction
The wearing of intra-oral prostheses is commonly associated with denture stomatitis. Candida species and bacteria coaggregate as biofilms on the denture surface and have the ability to cause damage to the oral mucosa, which is typified by inflammation and hyperplasia of the denture bearing tissue. This can, in immunocompromised patients, cause a burning sensation, discomfort, pain and disseminating infection; however, in the majority of cases, the individual is symptom free and unaware of a problem [1]. There are various factors that influence the onset and severity of denture stomatitis, such as the denture material (cleanliness, base material, trauma, duration of wear and its age), biological factors (cellular immunity, salivary flow, dietary factors, oral microbiota and pH of denture plaque) and smoking [2–4].
Denture stomatitis is a disease of predominantly fungal origin, with Candida albicans the most frequently isolated yeast from the oral cavities of these patients [5, 6]. Candida albicans is also the predominant biofilm forming yeast pathogen due to its ability to form hyphae, a key determinant of these complex consortia [7]. Candidal biofilms are clinically problematic as they are intrinsically resistant to antimicrobial agents [8]. In addition to this, one of the key virulence factors of C. albicans is the production of hydrolytic enzymes, which are utilised in the process of host tissue invasion and liberation of nutrients [9]. Secreted aspartyl proteinases (SAPs) are well-characterised examples of proteins related to adhesion and hydrolytic activity towards mucosal tissue [9–12]. These are encoded by a particularly prominent and diverse family of SAP genes, which play a major role in the pathogenesis of candidiasis. These are co-ordinately regulated depending on the environmental conditions and cellular morphology, that is, yeast or hyphae, which are also important in C. albicans biofilm development [7, 13, 14]. Two recent studies of C. albicans isolates from diabetic patients have shown that SAP production was increased in comparison to strains isolated from healthy controls [9, 14]. These data indicate that the biological properties of the isolates within different patients play an important role in disease outcome.
It was our hypothesis that proteinase activity is associated with biofilm formation and that differential expression of SAP’s within the biofilm may be related to the severity of disease within different patients. It was therefore the aim of this in vitro study to quantify both proteinase expression at a biochemical level from clinical strains isolated from patients with different levels of disease and SAP expression at different phases during biofilm development. This study demonstrates for the first time a correlation between disease severity and in vitro biofilm-related proteinase expression and biofilm phase-specific expression of SAP’s, particularly SAP8.
Materials and Methods
Culture Conditions and Standardisation
Twelve C. albicans clinical isolates were selected for this study. These included strains from patients with different levels of denture stomatitis [15], that is, Newton’s Type I [NT1 localised erythematous (n = 3)], Newton’s Type II [NT2 diffuse erythematous (n = 3)], Newton’s Type III [NT3 hyperplastic granular (n = 3)] and baseline control strains [NT0 asymptomatic carriage (n = 3)], which were collected from a previous denture stomatitis study [3]. All working stocks of C. albicans were maintained at 4°C on Sabouraud (SAB [Oxoid, Cambridge, UK]) agar and stored in Microbank® vials (Pro-Lab Diagnostics, Cheshire, UK) at −80°C. All isolates were prepared for biofilm studies by propagating in yeast peptone dextrose (YPD) medium [1% w/v yeast extract, 2% w/v peptone, 2% w/v dextrose (Oxoid)], washing by centrifugation and resuspending in RPMI-1640 (Sigma, Poole, UK).
Biofilm Formation
Candida albicans biofilms were formed as previously described [16]. Briefly, yeast cells were standardised to 1 × 106 cells/mL in RPMI-1640, which was dispensed into either a 24-well tissue culture plate for biomass and proteinase activity assays (1 mL) or into a 250 mL vent-capped polystyrene tissue culture-treated flask (Corning, Life Sciences, Oneonta, NY, USA) for gene expression analysis (50 mL). These were incubated statically for 1 h to allow cell adhesion, then placed on a rocking platform and incubated for a total of 12 and 24 h at 37°C, depending on the assay.
Quantification of Biofilm Formation
The capacity for each isolate to form biofilms was assessed using a biomass assay previously described by our group and adapted from the original method of Christensen [17, 18]. Following biofilm formation in 24-well tissue culture plates, the supernatants were removed for subsequent proteinase assays, the biofilms washed and then air-dried. One hundred microlitres of 0.5% (w/v) crystal violet solution was then added for 5 min. The solution was then removed by carefully rinsing the biofilms under running water until excess stain was removed. These were de-stained by adding 100 μL of 95% (v/v) ethanol into each well. The ethanol was gently pipetted to completely solubilise the crystal violet for 1 min. Subsequently, the ethanol was transferred to a new 96-well microtitre plate and absorbance read at 570 nm in a microtitre plate reader (FLUOstar OPTIMA, BMG Labtech, Aylesbury, Buckinghamshire, UK). The absorbance values are proportional to the quantity of biofilm biomass. All strains were tested in quadruplicate on three independent occasions.
Proteinase Activity
Candida albicans isolates were tested for proteinase activity using a spectrophotometric method, as described elsewhere [19]. The supernatants removed during the biomass assay were used for this analysis. Briefly, 100 μL of supernatant was mixed with 400 μL of 0.1 M sodium citrate buffer containing 1% bovine haemoglobin (Sigma, Poole, UK). Baseline controls for each inoculum were also prepared identically except for the addition of 500 μL of trichloroacetic acid [TCA (Fisher, Loughborough, UK)], which prevents the hydrolysis of haemoglobin. All samples were incubated for 1 h at 37°C and proteolytic activity halted with the addition of 500 μL of TCA. The solution was incubated on ice for 1 h and transferred to a microtitre plate reader and the absorbance level at 280 nm read (FLUOstar OPTIMA). Proteinase activity was calculated by a change in optical density readings between the experimental sample and its corresponding negative control. All strains were tested in quadruplicate on three independent occasions.
Nucleic Acid Preparation
Total RNA was extracted and purified as previously described by our group [16]. Briefly, biofilm material was disaggregated and removed from the flasks using sterile cell scrapers and placed into 2-mL screw cap vials (Stratech, Amsterdam, Netherlands). Excess medium was removed by centrifugation at 8,000×g for 2 min. RNA was extracted by mechanical disruption in TRIzol® (Invitrogen, Paisley, UK) and purified using an RNeasy MinElute clean up kit (Qiagen, Crawley, UK), as per the manufacturer’s instructions. RNA was quantified and the quality determined using a NanoDrop spectrophotometer™ (ND-1000, ThermoScientific, Loughborough, UK). cDNA was subsequently synthesised with MMLV reverse transcriptase (Invitrogen, Paisley, UK) using a MyCycler PCR machine (Bio-Rad Laboratories) and then stored at −70°C until required. All procedures following nucleic acid extraction and purification were performed inside a PCR Workstation (Labcare, Clevedon UK) with laminar vertical airflow to prevent external DNA contamination. All equipment was UV irradiated prior to use and filter tips used throughout for all procedures to avoid sample contamination.
Quantitative PCR
All primers were designed based on the sequence data obtained from the Candida Genome Database (CGD) website (http://www.candidagenome.org), which were applied to a web-based primer design software program Primer3 (http://frodo.wi.mit.edu/primer3/). Primers were checked for specificity to C. albicans using the NIH-BLAST for short or exact nucleotide sequences (http://www.nlm.nih.gov/BLAST/). Table 1 provides details of the oligonucleotides primer sequences that were synthesised by Eurogentec (Southampton, UK). The expression of each gene was assessed by quantitative RT-PCR using SYBR® GreenER™ (Invitrogen, Paisley, UK), according to the manufacturers’ instructions. Three independent replicate samples from each strain for each parameter were analysed in triplicate using an MxProP Quantitative PCR machine and MxProP 3000 software (Stratagene, Amsterdam, Netherlands) and gene expression normalised to the housekeeping gene ACT1 [20], according to the 2−ΔΔCT method [21]. PCR amplification efficiencies of all target genes were optimised prior to analysis.
Statistical Analysis
To investigate differences in SAP expression by real-time PCR and SAP enzymatic activity by the different NTs, because of the proportional nature of the data, both the expression data and enzymatic data were transformed to natural logarithm values (Ln), and ANOVA with Bonferroni tests was then applied to the transformed data. To investigate the differences in levels of SAP expression and activity between the early and mature biofilms, Levene’s tests were used to determine the variance of the data, the appropriate t test was selected for each comparison and this was performed on the Ln transformed data. A Bonferroni correction was applied to each analysis to correct for multiple testing. Statistical power computations indicated that with alpha set at 0.05 and an effect size of >3 (mean/standard deviation) group sizes of 3 would be sufficient to see significant differences between the mean values.
To investigate the relationship between the SAP expression and SAP enzymatic activity in the 4 NTs, a series of bivariate Spearman correlations (Rho) were computed on the original proportional data. Since each different SAP (n = 5) was tested individually against SAP activity and not against each other, a less conservative correction was required for the repeated tests. Therefore, a Bonferroni correction to alpha (0.01) was applied before calculating the statistical power of the effect size (Rho2) of the correlations instead of computing the power of 5 regressors collectively. To test whether the NTs influenced these correlations, a series of partial correlations were calculated. To do this, it was necessary to utilise the Ln transformed data. The post hoc statistical power of each correlation was calculated as described above. SPSS (Version 11, Chicago, USA) was used for these analyses and GraphPad Prism (Version 4, La Jolla, USA) for the production of the figures.
Results
Proteinase Activity within Biofilms and Correlation with Disease Subtype
The biofilms of isolates pertaining to each disease subtype showed similar levels of overall biofilm biomass after 24 h growth. These ranged from Ab570 levels of 1.476–1.511. No significant differences were observed between each group. However, comparison of the normalised proteinase activity of these isolates showed significant differences between the groups (p < 0.0001). All three diseased groups displayed significant increases in proteinase activity (NT1 = 0.052, p < 0.05; NT2 = 0.0535, p < 0.01; NT3 = 0.0574, p < 0.001) compared to the baseline control group (0.0444). A highly significant difference was also observed between the proteinase activity of NT1 and NT3 (p < 0.001) (Fig. 1).
Proteinase activity of mature biofilms from strains isolated from different levels of disease. Proteinase activity was determined spectrophotometrically and normalised to the levels of biofilm biomass from isolates (n = 3) representing NT0, NT1, NT2 and NT3. Significant differences were observed between each group (p < 0.0001), and as the level of disease increased compared to the baseline isolates (*p < 0.05, **p < 0.001; ***p < 0.001)
The Spearman correlations showed that there was a very strong correlation between enzyme activity and expression of all five SAPs (p < 0.0001 and statistical power >96%). The partial correlation on the Ln transformed data adjusting for the different NTs showed that for SAP1, SAP2 and SAP8, there was a very strong correlation with SAP enzyme activity. The statistical power for the effect size of these correlations was >84%. SAP5 and SAP6 no longer correlated with SAP activity in this analysis (Table 2).
SAP Expression is Correlated with Biofilm Phase and Disease Subtype
The expression of SAP1, SAP2, SAP5, SAP6 and SAP8 transcripts was investigated in 12 and 24 h C. albicans biofilms. The expression of the housekeeping gene ACT1 did not differ significantly throughout the experiment between the different NTs or the different time points. Expression of these genes compared to the housekeeping gene showed a wide variation of expression, with SAP8 showing the greatest maximal range of expression (303-fold), followed by SAP5 (185-fold), SAP6 (42-fold), SAP2 (eightfold) and SAP1 (sixfold). The highest levels of expression for these genes were for the NT3 isolates, and there was a general trend with respect to disease severity and SAP expression. ANOVA showed that there were highly statistically significant differences in the expression of the SAPs by both the early and the mature NT groups of isolates (p < 0.0001). The post hoc test showed that all genes were significantly up-regulated in early biofilms of NT1, NT2 and NT3 strains compared to healthy control strains (p < 0.0001), except for SAP2 for the NT2 isolates (p = 0.375) and for SAP6 for the NT1 isolates (p = 0.304). Significant differences were also observed between each NT (p ≤ 0.005), except for SAP5 between NT1 and NT2 (p = 0.336). In mature biofilms, again significant up-regulation of each gene was also observed in NT1, NT2 and NT3 strains compared to control strains (p < 0.0001), except for SAP2 for NT1 isolates (p = 0.576). Significant differences were also observed between each NT (p ≤ 0.016), except for SAP6 between NT2 and NT3 (p = 1.000) and SAP8 between NT1 and NT2 (p = 0.181), NT1 and NT2 (p = 0.353), and NT1 and NT3 (p = 1.000) (Fig. 2).
SAP expression profiles of early and mature biofilms from strains isolated from different levels of disease. SAP expression (SAP1, 2, 5, 6 and 8) was assessed by qPCR from isolates (n = 3) representing NT0, NT1, NT2 and NT3. The levels of gene expression are represented as fold change compared to the housekeeping gene actin (ACT1). Significant differences (p < 0.0001) were observed between NT0 and the disease groups in early (ψ) and mature (ϕ) biofilms as indicated. Significant differences were also observed between early and mature biofilms (*p < 0.005)
The t tests showed there were also differences in the expression of all of the SAPs when early biofilms were compared with mature biofilm (p < 0.005), except for SAP1 (p = 0.058) for the healthy controls and for SAP6 for the NT3 strains (p = 1.000). SAP5 expression was consistently higher in the early NT isolates, whereas SAP6 and SAP8 expressions were consistently lower in the early biofilms compared with the mature biofilms. Both SAP1 and SAP2 showed increasing expression in the more severe types, but overall the relative levels of expression were low.
Discussion
Denture stomatitis is associated with the presence of candidal biofilms adherent to the upper fitting acrylic surface of the denture [22]. The close proximity of the biofilm and extrapolymeric matrix to the oral mucosa induces localised innate inflammatory responses, accounting for erythema and other clinical symptoms [15]. However, given the clinical spectrum of disease for patients with denture stomatitis, the presence of biofilm alone does not explain the clinical presentation. Clearly, host immune factors are likely to play a role, but we hypothesised that strain-specific factors and the level of biofilm formation may also impact the level of pathophysiology. Independent from host immune factors, we demonstrated using representative C. albicans strains isolated from denture stomatitis patients, a correlation between in vitro biofilm associated proteinase activity and severity of diseased site from which each strain was originally isolated.
Biofilm formation is a fundamental characteristic of C. albicans in the oral cavity [22]. Work from our group and others has shown that its capacity to attach to dentures maintains its retention and provides protection within the oral environment [3, 23, 24]. We therefore evaluated initially the capacity of a panel of strains to form biofilms, albeit in an artificial in vitro environment. All twelve isolates formed robust biofilm structures, which when grouped according to disease severity showed no significant difference to one another (data not shown). We concluded that efficiency of biofilm formation by the clinical isolate per se did not explain disease severity, suggesting the possibility that specific microbial factors secreted from C. albicans within the biofilm may be relevant.
Proteinases are key hydrolytic enzymes involved in oral candidosis, reported to be associated with biofilm formation [25]. Therefore, the role of SAPs was explored at the biochemical and molecular levels in the context of biofilms and disease. Tsang and colleagues (2007) [9] previously reported that proteinase production was significantly greater from C. albicans isolated from diseased patients when compared to healthy controls. Moreover, a positive relationship between proteinase activity and biofilm formation was observed in a subset of oral C. albicans isolates associated with disease [14]. In the present study, it was demonstrated that those strains isolated from individuals with overt signs of disease showed significantly greater levels of proteinase activity than healthy individuals’ isolates (control), particularly the NT3 group of biofilm isolates. These were also highly correlated with SAP gene expression. It was previously noted that SAP activity from strains isolated from patients with oral candidosis was inherently higher than asymptomatic control strains from oral candidosis patients [19]. Previous work has also suggested a relationship between genotype and pathogenesis, in which variation of proteinase expression was described between different genotypes [26]. This and the observations herein lend support to the hypothesis that strain-specific factors are more important in causing disease than the presence of biofilm alone [27, 28].
We next evaluated the transcription of key SAP genes to determine whether individual strains from defined disease groupings preferentially up-regulated their SAP expression. Recent studies of aspartyl proteinase mRNA abundance have indicated that the contribution of secreted aspartyl proteinases is low [25]. Instead, these authors indicated that hyphal formation is the predominant cause of damage to tissues. We therefore examined the relative mRNA abundance within strains from each disease group and compared these to non-diseased individuals to determine whether in a controlled biofilm model we could ascertain the contribution of individual SAP genes. Based on the two main enzyme isotype groups and our own in vivo analysis of mRNA abundance in denture sonicate samples (data not shown) [3], we decided to focus on a subset of genes (SAP1, 2, 5, 6 and 8). It was noteworthy that the infrequently described SAP8 was consistently detected in our clinical samples, a gene which clusters with SAPs1–3.
A strong correlation between mRNA and protein expression was demonstrated throughout. Those groups of isolates from the diseased individuals showed the greatest expression levels of each SAP in both early and mature biofilms, which ascended with severity of disease. Of note, the levels of expression of both SAP1 and SAP2 genes that have been consistently linked to adhesion and mucosal destruction were at low levels compared to the other genes [29, 30]. Nevertheless, both genes were significantly correlated with strains isolated from diseased patients. SAP2 is described as the predominant member of the gene family, required for optimal growth on medium containing only protein [31, 32]. It has been shown that a C. albicans triple ∆sap4–6 knockout mutant was inhibited in its ability to use protein as a sole nitrogen source, but can grow on complex media [33]. These data suggest that SAP4–6 may be co-regulated through expression of SAP2, which may be true given the higher levels of SAP5 and SAP6 expression in this study.
SAP5 and SAP6 have been described as being associated with hyphal growth and responsible for invasive candidal infection, due to its association with hyphal development [31, 34], an important factor in biofilm development [7, 35]. A previous clinical study showed that 40% (32/80) of oral infection isolates exhibited SAP6 expression as opposed to 29% (20/69) of carrier isolates [36]. Interestingly, SAP5 showed greater expression during early phase biofilm growth, which suggests that its expression may be phase dependent during the early aggressive stages of adhesion and invasion. In fact, it has recently been reported independently that SAP5 was highly up-regulated in an in vitro reconstituted human epithelial model [25, 35]. Analysis of SAP5 and SAP6 expression to proteinase activity demonstrated an overall correlation with proteinase activity, yet when adjustments were made to the levels of disease then proteinase activity no longer correlated with SAP5 and SAP6 expression. Given the proposed function of these proteins then it is likely that their expression is related to overall growth characteristics of the biofilm. The combined role of SAPs1-6 in virulence has now come into question, particularly with respect to SAP1-3 [37]. In fact, it has been shown that Sap proteins may induce inflammatory responses independently of enzymatic activity and pH [38].
Of the five genes examined, SAP8 expression was the most notable, a member of the gene family that has had minimal study [39]. It was shown to be highly up-regulated in mature biofilms formed by strains isolated from diseased patients and also correlated with proteinase activity. Previous work by Naglik and co-workers (2003) described its association with in vivo oral infection isolates [36]. However, further work from this group indicated that its expression tends to be relatively low [25]. Nevertheless, reports have suggested it does have a role in infection, where it was reported to be highly expressed in deeper sites of infection, sites that may be oxygen depleted [40]. We also know that it is closely related to SAP1 and SAP2, which showed similar expression and proteinase activity correlation profiles. All three of these enzymes are reported to function optimally at low pH [41]. Mature biofilms have a heterogeneous environment, making it highly probable that the redox and pH are variable throughout the layers of the biofilm. Recent studies have shown that SAP activity is modulated by oxygen availability amongst other things [42], so it is plausible that SAP8 expression is optimal in biofilms, but this remains to be determined in vivo. However, its expression profile in strains isolated from patients with denture stomatitis indicates that strain-dependent characteristics may preferentially govern its regulation, to which biofilm growth may be a key factor.
Whilst admittedly there are limitations to this study, such as relatively small group sizes and the absence of a physiologically relevant biofilm system, the controlled experimental parameters in an established biofilm model provide the basis for further detailed investigation. It is recognised that there may be significant differences in expression of virulence-related genes between different model systems, and care must be taken in extrapolating from the in vitro model described to the in vivo situation. Nevertheless, the significant differences in SAP activity between isolates from diseased patients and healthy controls were striking and suggest that SAP activity may play an important role in biofilm-associated denture stomatitis. The potential role of SAP8, a previously poorly defined member of the gene family, is of particular interest as this is the first time that it has been shown to play a prominent role in biofilms.
References
Pereira-Cenci T, Del Bel Cury AA, Crielaard W, Ten Cate JM. Development of Candida-associated denture stomatitis: new insights. J Appl Oral Sci. 2008;16(2):86–94.
Oksala E. Factors predisposing to oral yeast infections. Acta Odontol Scand. 1990;48(1):71–4.
Coco BJ, Bagg J, Cross LJ, Jose A, Cross J, Ramage G. Mixed Candida albicans and Candida glabrata populations associated with the pathogenesis of denture stomatitis. Oral Microbiol Immunol. 2008;23(5):377–83.
Gasparoto TH, Vieira NA, Porto VC, Campanelli AP, Lara VS. Ageing exacerbates damage of systemic and salivary neutrophils from patients presenting Candida-related denture stomatitis. Immun Ageing. 2009;6:3.
Baena-Monroy T, Moreno-Maldonado V, Franco-Martinez F, Aldape-Barrios B, Quindos G, Sanchez-Vargas LO. Candida albicans, Staphylococcus aureus and Streptococcus mutans colonization in patients wearing dental prosthesis. Med Oral Patol Oral Cir Bucal. 2005;10(Suppl 1):E27–39.
McIntyre GT. Oral candidosis. Dent Update. 2001;28(3):132–9.
Ramage G, VandeWalle K, Lopez-Ribot JL, Wickes BL. The filamentation pathway controlled by the Efg1 regulator protein is required for normal biofilm formation and development in Candida albicans. FEMS Microbiol Lett. 2002;214(1):95–100.
Ramage G, Saville SP, Thomas DP, Lopez-Ribot JL. Candida biofilms: an update. Eukaryot Cell. 2005;4(4):633–8.
Tsang CS, Chu FC, Leung WK, Jin LJ, Samaranayake LP, Siu SC. Phospholipase, proteinase and haemolytic activities of Candida albicans isolated from oral cavities of patients with type 2 diabetes mellitus. J Med Microbiol. 2007;56(Pt 10):1393–8.
Marcos-Arias C, Eraso E, Madariaga L, Aguirre JM, Quindos G. Phospholipase and proteinase activities of Candida isolates from denture wearers. Mycoses. 2009.
Naglik JR, Newport G, White TC, Fernandes-Naglik LL, Greenspan JS, Greenspan D, et al. In vivo analysis of secreted aspartyl proteinase expression in human oral candidiasis. Infect Immun. 1999;67(5):2482–90.
Ruchel R, de Bernardis F, Ray TL, Sullivan PA, Cole GT. Candida acid proteinases. J Med Vet Mycol. 1992;30(Suppl 1):123–32.
Naglik JR, Challacombe SJ, Hube B. Candida albicans secreted aspartyl proteinases in virulence and pathogenesis. Microbiol Mol Biol Rev. 2003;67(3):400–28. (table of contents).
Rajendran R, Robertson DP, Hodge PJ, Lappin DF, Ramage G. Hydrolytic enzyme production is associated with Candida albicans biofilm formation from patients with type 1 diabetes. Mycopathologia. 2010;170(4):229–35.
Newton A. Denture sore mouth: a possible aetiology. Br Dent J. 1962;112:357–60.
Ramage G, Bachmann S, Patterson TF, Wickes BL, Lopez-Ribot JL. Investigation of multidrug efflux pumps in relation to fluconazole resistance in Candida albicans biofilms. J Antimicrob Chemother. 2002;49(6):973–80.
Christensen GD, Simpson WA, Younger JJ, Baddour LM, Barrett FF, Melton DM, et al. Adherence of coagulase-negative staphylococci to plastic tissue culture plates: a quantitative model for the adherence of staphylococci to medical devices. J Clin Microbiol. 1985;22(6):996–1006.
Jose A, Coco BJ, Milligan S, Young B, Lappin DF, Bagg J, et al. Reducing the incidence of denture stomatitis: are denture cleansers sufficient? J Prosthodont. 2010;19(4):252–7.
Kuriyama T, Williams DW, Lewis MA. In vitro secreted aspartyl proteinase activity of Candida albicans isolated from oral diseases and healthy oral cavities. Oral Microbiol Immunol. 2003;18(6):405–7.
Watamoto T, Samaranayake LP, Egusa H, Yatani H, Seneviratne CJ. Transcriptional regulation of drug-resistance genes in Candida albicans biofilms in response to antifungals. J Med Microbiol. 2011;60(Pt 9):1241–7.
Livak KJ, Schmittgen TD. Analysis of relative gene expression data using real-time quantitative PCR and the 2(-Delta Delta C(T)) Method. Methods. 2001;25(4):402–8.
Rautemaa R, Ramage G. Oral candidosis—clinical challenges of a biofilm disease. Crit Rev Microbiol. 2011;37(4):328–36.
Campos MS, Marchini L, Bernardes LA, Paulino LC, Nobrega FG. Biofilm microbial communities of denture stomatitis. Oral Microbiol Immunol. 2008;23(5):419–24.
Ramage G, Tomsett K, Wickes BL, Lopez-Ribot JL, Redding SW. Denture stomatitis: a role for Candida biofilms. Oral Surg Oral Med Oral Pathol Oral Radiol Endod. 2004;98(1):53–9.
Naglik JR, Moyes D, Makwana J, Kanzaria P, Tsichlaki E, Weindl G, et al. Quantitative expression of the Candida albicans secreted aspartyl proteinase gene family in human oral and vaginal candidiasis. Microbiology. 2008;154(Pt 11):3266–80.
Sugita T, Kurosaka S, Yajitate M, Sato H, Nishikawa A. Extracellular proteinase and phospholipase activity of three genotypic strains of a human pathogenic yeast, Candida albicans. Microbiol Immunol. 2002;46(12):881–3.
Costa CR, Jesuino RS, de Aquino Lemos J, de Fatima Lisboa F, Fernandes O, Hasimoto e Souza LK, Passos XS, et al. Effects of antifungal agents in sap activity of Candida albicans isolates. Mycopathologia. 2010;169(2):91–8.
Mores AU, Souza RD, Cavalca L, de Paula e Carvalho A, Gursky LC, Rosa RT, et al. Enhancement of secretory aspartyl protease production in biofilms of Candida albicans exposed to sub-inhibitory concentrations of fluconazole. Mycoses. 2011;54(3):195–201.
Schaller M, Bein M, Korting HC, Baur S, Hamm G, Monod M, et al. The secreted aspartyl proteinases Sap1 and Sap2 cause tissue damage in an in vitro model of vaginal candidiasis based on reconstituted human vaginal epithelium. Infect Immun. 2003;71(6):3227–34.
Schaller M, Schafer W, Korting HC, Hube B. Differential expression of secreted aspartyl proteinases in a model of human oral candidosis and in patient samples from the oral cavity. Mol Microbiol. 1998;29(2):605–15.
Hube B, Monod M, Schofield DA, Brown AJ, Gow NA. Expression of seven members of the gene family encoding secretory aspartyl proteinases in Candida albicans. Mol Microbiol. 1994;14(1):87–99.
White TC, Agabian N. Candida albicans secreted aspartyl proteinases: isoenzyme pattern is determined by cell type, and levels are determined by environmental factors. J Bacteriol. 1995;177(18):5215–21.
Sanglard D, Hube B, Monod M, Odds FC, Gow NA. A triple deletion of the secreted aspartyl proteinase genes SAP4, SAP5, and SAP6 of Candida albicans causes attenuated virulence. Infect Immun. 1997;65(9):3539–46.
Felk A, Kretschmar M, Albrecht A, Schaller M, Beinhauer S, Nichterlein T, et al. Candida albicans hyphal formation and the expression of the Efg1-regulated proteinases Sap4 to Sap6 are required for the invasion of parenchymal organs. Infect Immun. 2002;70(7):3689–700.
Nailis H, Kucharikova S, Ricicova M, Van Dijck P, Deforce D, Nelis H, et al. Real-time PCR expression profiling of genes encoding potential virulence factors in Candida albicans biofilms: identification of model-dependent and -independent gene expression. BMC Microbiol. 2010;10:114.
Naglik JR, Rodgers CA, Shirlaw PJ, Dobbie JL, Fernandes-Naglik LL, Greenspan D, et al. Differential expression of Candida albicans secreted aspartyl proteinase and phospholipase B genes in humans correlates with active oral and vaginal infections. J Infect Dis. 2003;188(3):469–79.
Correia A, Lermann U, Teixeira L, Cerca F, Botelho S, da Costa RM, et al. Limited role of secreted aspartyl proteinases Sap1 to Sap6 in Candida albicans virulence and host immune response in murine hematogenously disseminated candidiasis. Infect Immun. 2010;78(11):4839–49.
Pietrella D, Rachini A, Pandey N, Schild L, Netea M, Bistoni F, et al. The Inflammatory response induced by aspartic proteases of Candida albicans is independent of proteolytic activity. Infect Immun. 2010;78(11):4754–62.
Monod M, Hube B, Hess D, Sanglard D. Differential regulation of SAP8 and SAP9, which encode two new members of the secreted aspartic proteinase family in Candida albicans. Microbiology. 1998;144(Pt 10):2731–7.
Schaller M, Schackert C, Korting HC, Januschke E, Hube B. Invasion of Candida albicans correlates with expression of secreted aspartic proteinases during experimental infection of human epidermis. J Invest Dermatol. 2000;114(4):712–7.
Aoki W, Kitahara N, Miura N, Morisaka H, Yamamoto Y, Kuroda K, et al. Comprehensive characterization of secreted aspartic proteases encoded by a virulence gene family in Candida albicans. J Biochem. 2011;150(4):431–8.
Carvalho AP, Gursky LC, Rosa RT, Rymovicz AU, Campelo PM, Gregio AM, et al. Non-steroidal anti-inflammatory drugs may modulate the protease activity of Candida albicans. Microb Pathog. 2010;49(6):315–22.
Acknowledgments
We would like to acknowledge the Chief Scientist Office (CZG/1/152) for financial support.
Author information
Authors and Affiliations
Corresponding author
Rights and permissions
About this article
Cite this article
Ramage, G., Coco, B., Sherry, L. et al. In Vitro Candida albicans Biofilm Induced Proteinase Activity and SAP8 Expression Correlates with In Vivo Denture Stomatitis Severity. Mycopathologia 174, 11–19 (2012). https://doi.org/10.1007/s11046-012-9522-2
Received:
Accepted:
Published:
Issue Date:
DOI: https://doi.org/10.1007/s11046-012-9522-2