Abstract
Physiologic concentration in amniotic fluid (AF) of several metabolites has not been established with certainty. In this study, we initially assayed purines, pyrimidines, and amino compounds in 1,257 AF withdrawn between the 15th and the 20th week of gestation from actually normal pregnancies (normal gestations, normal offspring). Results allowed to determine physiologic reference intervals for 45 compounds. In these AF, not all purines and pyrimidines were detectable and uric acid (238.35 ± 76.31 μmol/l) had the highest concentration. All amino compounds were measurable, with alanine having the highest concentration (401.10 ± 88.47 μmol/l). In the second part of the study, we performed a blind metabolic screening of AF to evaluate the utility of this biochemical analysis as an additional test in amniocenteses. In 1,295 additional AF from normal pregnancies, all metabolites fell within the confidence intervals determined in the first part of the study. In 24 additional AF from women carrying Down’s syndrome-affected fetuses, glutamate, glutamine, glycine, taurine, valine, isoleucine, leucine, ornithine, and lysine were different from physiologic reference values. One AF sample showed phenylalanine level of 375.54 μmol/l (mean value in normal AF = 65.07 μmol/l) and was from a woman with unreported phenylketonuria with mild hyperphenylalaninemia (serum phenylalanine = 360.88 μmol/l), carrying the IVS 4 + 5 G-T and D394A mutations. The fetus was heterozygote for the maternal D394A mutation. An appropriate diet maintained the mother phenylalanine in the range of normality during pregnancy, avoiding serious damage in fetal and neonatal development. These results suggest that the metabolic screening of AF might be considered as an additional biochemical test in amniocenteses useful to highlight anomalies potentially related to IEM.
Similar content being viewed by others
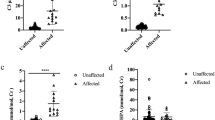
Avoid common mistakes on your manuscript.
Introduction
The analysis of low molecular weight compounds in biological fluids is of high clinical value in the screening/diagnosis of inborn errors of metabolism 1(IEM) [1–3]. For instance, the determination of circulating and/or excreted purines, pyrimidines, amino acids, and organic acids is a crucial analytical tool to diagnose several IEM, some of which have benign outcome if diagnosed at a very early stage. For this reason, the number of national programs of neonatal screenings of IEM is continuously increasing worldwide. What metabolites have to be determined and, therefore, what IEM have to be selectively screened are certainly not simple to decide, and it is mainly dictated by some major factors: (i) the possibility of reducing/eliminating the damages caused to the newborn if the IEM is precociously diagnosed; (ii) the availability of suitable analytical methods allowing the simultaneous assay of a broad number of compounds related to the IEM of interest; (iii) when these methods are available, the cost for the health care system [4].
If the progresses in the metabolic neonatal screenings have certainly been impressive, the same cannot be affirmed in the case of the metabolic prenatal screenings of IEM. At present, no large-scale studies carried out by measuring metabolites in amniotic fluid (AF) and applying the same criteria used in the neonatal screenings (large size of samples screened, broad number of analytes assayed, population with unknown risk for IEM included in the screening) have been performed. Furthermore, actual concentrations of purines, pyrimidines, and amino acids in AF of normal pregnancies are still uncertain, mainly because the ranges of variability of these metabolites have been obtained in a limited number of samples [5–9]. Even recent studies reporting the analysis of AF by in vitro magnetic resonance spectroscopy to evaluate fetal disturbances [10–12], and studies reporting values of organic acids in AF of normal pregnant women [13], have been obtained in a restricted number of subjects. In such a delicate clinical setting, it is mandatory to have reliable physiologic reference values for each compound under study that would then allow to individuate samples falling out of these intervals, thus indicating possible fetal anomalies including IEM.
In this study, we initially determined reliable confidence intervals of 45 different metabolites (purines, pyrimidines, creatinine, N-acetylaspartate, methylmalonic acid, malonic acid, and amino group-containing compounds) in 1,257 AF samples from actually normal pregnancies, obtaining convincing reference values also for compounds with still unknown concentrations in normal AF (several purines, most of pyrimidines, NAA, several amino group-containing compounds). This result for such a broad spectrum of analytes might be useful to evidence possible anomalous metabolic profile of AF samples potentially related to more than 40 relevant IEM. A group of ten AF from Down’s syndrome-affected fetuses was also analyzed in this first part of the study. During the second part of the study, using these physiologic reference values and applying the criteria adopted in the neonatal setting (broad spectrum of analytes, population with unknown risk for IEM), we undertook a screening in AF from women who underwent amniocentesis at the second trimester of pregnancy, in which the analysis of these 45 metabolites was performed. After 1,681 additional AF analyzed in this second part, we recorded one AF with abnormal phenylalanine concentration that was due to a previously undiagnosed case of maternal mild hyperphenylalaninemia. Results obtained in this blind metabolic prenatal screening are presented.
Materials and methods
Chemicals
HPLC-grade tetrabutylammonium hydroxide, used as the pairing reagent in the separation of purines and pyrimidines, was supplied from Nova Chimica (Cinisello Balsamo, Milano, Italy) as a 55% water solution. Ortophtalaldehyde (OPA) and 3-mercaptopropionic acid (MPA), used as the reagents to obtain fluorescent-derivatized amino compounds, were purchased from Sigma-Aldrich (St. Louis, Mo, USA), as well as ultrapure standards of purines, pyrimidines, standard and non-standard amino acids, amino compounds, N-acetylaspartate (NAA), malonic acid (MA), and methylmalonic acid (MMA). HPLC-grade acetonitrile, methanol, and tetrahydrofurane were purchased from J.T Baker (Mallinckrodt Baker, Deventer, Netherland). All other chemicals (trifluoroacetic acid, sodium borate, sodium acetate, KH2PO4, Na2HPO4) were of the highest purity available from commercial sources.
Patient selection and processing of amniotic fluid and blood samples
In the first part of the study, AF samples were obtained from 1,750 consecutive pregnant women aging 30–44 years (mean = 38 ± 4 years), who underwent amniocentesis at the second trimester of pregnancy (15–20 weeks of gestation) for cytogenetic-based diagnosis. Amniocenteses were carried out at the Department of Prenatal Diagnosis of the Fetal-Maternal Medical Centre “ARTEMISIA”, Rome, Italy, between September and December 2009. Of these 1,750 AF samples, 210 were not assayed because mothers did not give consent to perform the biochemical analyses and 68 because mothers were carrying fetuses suffering from morphological anomalies, growth retardation, and any cytogenetic disorder different from Down’s syndrome. The remaining 1,472 samples, after having obtained the fully informed consent from each pregnant woman, were analyzed for the HPLC determination of purines, pyrimidines, and amino group-containing compounds.
In the second part of the study (June 2010–February 2011), in AF samples of all fully consenting women who underwent amniocentesis at the second trimester of pregnancy (15–20 weeks of gestation) for cytogenetic-based diagnosis at the same Medical Centre, the analysis of purines, pyrimidines, and amino group-containing compounds was performed in 1,681 AF samples. The aim was to test the feasibility of prenatal diagnosis of IEM based on the clinical-biochemical criteria adopted in the neonatal setting (analysis of several metabolites in biological fluid, large number of samples screened, population with unknown risk for IEM included in the study). The study was approved by the Ethic Review Board for human studies of Catania University.
All AF samples were subjected to the same handling and processing. Briefly, after collection of the standard volume of AF necessary for the cytogenetic-based analyses (15–20 ml), samples were centrifuged at 180×g at 22°C for 10 min. One milliliter of the residual cell-free AF was properly processed and used to determine the purine, pyrimidine, and amino acid profiles within 24 h from amniocentesis. Samples of AF were deproteinized as described in detail elsewhere [14]. Briefly, AF was centrifuged at 20,817×g at 4°C for 20 min in an Eppendorf 5804 R centrifuge (Hamburg, Germany). This clear supernatant was diluted by adding 0.5 ml of HPLC-grade water and then transferred to a Nanosep® centrifugal device equipped with a filtering membrane of 3-kDa cutoff (Pall Gelman Laboratory, Ann Harbor, MI, USA) and centrifuged at 20,817×g at 4°C. The resulting deproteinized AF samples were directly used for the HPLC determinations of the compounds of interest.
Peripheral venous blood samples were collected from the antecubital vein into a VACUETTE® polypropylene tube, containing serum separator and clot activator (Greiner-Bio One GmbH, Kremsmunster, Austria). After 40 min at room temperature, samples were centrifuged at 1890×g for 10 min, and the obtained sera were diluted with HPLC-grade water (1:3; v:v) and then deproteinized by ultrafiltration as above described. The protein-free sera were directly used for the HPLC determinations of the compounds of interest.
HPLC apparatuses and conditions for the separation of purines, pyrimidines, and amino acids
Four twin HPLC apparatuses (ThermoFisher Italia, Rodano, Milan, Italy), two dedicated to assay purines and pyrimidines and two to assay amino acids, were used. They consisted of a Surveyor quaternary pump system, a Surveyor AS autosampler, and a highly sensitive photodiode array detector equipped with a 5-cm light path flow cell and set up between 200 and 400 nm wavelength. Data acquisition and analysis were performed by a PC using the ChromQuest® software package provided by the HPLC manufacturer.
The synchronous determination of creatinine, purines (hypoxanthine, xanthine, uric acid, inosine, guanosine), pyrimidines (uracil, β-pseudouridine, cytidine, thymine, uridine, thymidine, orotic acid), malonic acid (MA), methylmalonic acid (MMA), and NAA was carried out isocratically on 100 μl of deproteinized AF, as described in detail elsewhere [14]. At the end of the data acquisition of each sample (28 min total), the HPLC column was automatically washed with HPLC-grade water for 3 min at 1.5 ml/min, then cleaned with a mobile phase B (40% CH3OH + 30% CH3CN + 30% H2O) for 15 min at 1.5 ml/min, then washed again with HPLC-grade water for 3 min at 1.5 ml/min, then re-equilibrated with mobile phase A for 10 min at 1.5 ml/min, and lastly stabilized at a flow rate of 1.2 ml/min for 1 min, before a new sample injection (total time between 2 consecutive injections = 60 min). Assignment and calculation of the compounds of interest in chromatographic runs of AF extracts were carried out at 234 (creatinine), 260 nm (purine and pyrimidine compounds), and 206 nm wavelengths (MA, MMA and NAA) by comparing retention times, absorption spectra and areas of peaks with those of peaks of chromatographic runs of freshly prepared ultra-pure standard mixtures with known concentrations. To perform a systematic check of the efficiency of the HPLC column, a freshly prepared standard mixture was assayed every ten AF samples.
The simultaneous determination of 29 primary amino group-containing compounds, including aspartate (Asp), glutamate (Glu), argininosuccinate (Arg-succ), asparagine (Asn), α-aminoadipic acid (AAAA), serine (Ser), glutamine (Gln), histidine (His), S-adenosylmethionine (SAM), glycine (Gly), threonine (Thr), citrulline (Cit), arginine (Arg), alanine (Ala), carnosine (Carn), taurine (Tau), γ-aminobutyrate (GABA), tyrosine (Tyr), S-adenosylhomocysteine (SAH), l-cystathionine (Cystat), valine (Val), methionine (Met), NH3, tryptophane (Trp), phenylalanine (Phe), isoleucine (Ile), leucine (Leu), ornithine (Orn), lysine (Lys), plus the internal standard norvaline (Norval), was performed using the precolumn derivatization of the sample with a mixture of OPA and MPA. Briefly, stock solutions of 625 mmol/l OPA in methanol and of 250 mmol/l sodium borate, pH 9.8, were prepared. OPA solution was stored at 4°C in the dark and used for a week with no loss of efficiency, nor development of undesired absorbing products. Ten milliliters of the derivatization mixture composed of 25 mmol/l OPA (400 μl of the stock solution), 1% MPA, 237.5 mmol/l sodium borate, pH 9.8 (9.5 ml of the sodium borate stock solution) was prepared daily and placed in the autosampler. The automated precolumn derivatization of the samples with OPA-MPA was carried out at 24°C, according to the injector program illustrated in Table 1. After derivatization, 25 μl was loaded onto the HPLC column (Hypersil C-18, 250 × 4.6 mm, 5 μm particle size, thermostated at 21°C) for the subsequent chromatographic separation. Separation was carried out at a flow rate of 1.2 ml/min using two mobile phases having the following composition: mobile phase A = 24 mmol/l CH3COONa + 24 mmol/l Na2HPO4 + 1% tetrahydrofurane + 0.1% trifluoroacetic acid, pH 6.5; mobile phase B = 40% CH3OH + 30 CH3CN + 30% H2O. The form of the step gradient used for the separation was as follows: 2 min to 100% A; 3 min to 90% A; 10 min to 78% A; 10 min to 55% A; 8 min to 48% A; 5 min to 35% A; 2 min to 20% A; hold 5 min 20% A. Washing steps to clean the column before a new injection were as follows: 3 min 100% HPLC-grade H2O (flow rate 1.5 ml/min); 10 min 20% A (flow rate 1.5 ml/min); 3 min 100% HPLC-grade H2O (flow rate 1.5 ml/min); 2 min 100% A (flow rate 1.5 ml/min); 1 min 100% A to stabilize the flow rate at 1.2 ml/min. During the derivatization process of a new sample (6 min), the column was equilibrated with 100% A (1.2 ml/min). The total time between two consecutive injections was of 70 min. Assignment and calculation of the derivatized amino compounds in chromatographic runs of AF extracts were carried out at 338 nm wavelengths by comparing retention times and areas of peaks with those of peaks of chromatographic runs of freshly prepared ultra-pure standard mixtures with known concentrations. To perform a systematic check of the efficiency of the HPLC column, a freshly prepared standard mixture was assayed every ten AF samples.
Statistics
All variables were skewed and, therefore, were log-transformed to approach Gaussian distribution before to calculate confidence intervals at a probability level of 95% and before application of parametric tests. Values recorded in Down’s syndrome fetuses were compared with those in controls with a multivariate test using the SAS/STAT package (SAS Institute Inc., Cary, NC, USA). A cluster analysis was conducted by the Ward’s minimum variance criterion. In addition, the mean values of the different parameters in AF of Down’s syndrome fetuses were compared with those calculated in the AF of actually normal pregnancies using the z-test. A value of P < 0.05 was considered significant.
Results
Of the 1,472 AF donors, enrolled in the first part of the study between September and December 2009, 205 could not be contacted to effect the follow-up about pregnancies, labors, deliveries, and offspring, necessary to include each sample in the group of actually normal pregnancies. Ten of these 1,472 AF samples were from fetuses affected by Down’s syndrome and were treated as a separate group of data. Therefore, means, standard deviations, and confidence intervals of the metabolites of interest in actually normal pregnancies were obtained from 1,257 AF samples. Table 2 reports the specific indications for amniocentesis in these 1,257 women.
Concentrations of purines, pyrimidines, creatinine, MA, MMA, NAA, and amino group-containing compounds in normal AF
Figure 1 reports mean values ± standard deviations of the different compounds detected in 1,257 AF from normal pregnancies between the 15th and the 20th week of gestation. To run all these AF samples, six HPLC columns (three dedicated to purines and pyrimidines and three to amino compounds) and eight guard-columns were used, indicating that the sample preparation and the washing programs at the end of each sample run greatly increased the column life span, thereby reducing the overall analytical costs. In all our AF samples, cytidine, thymine, inosine, guanosine, MA, MMA, and NAA were undetectable with the present method. For the aforementioned compounds, lowest limit of quantifications (LOQ) were 0.08, 0.15, 0.04, 0.08, 0.08, 0.4, 3, and 3 μmol/l, respectively, confirming the values reported originally [14]. In Table 3, the confidence intervals at the 95% level, approximated to obtain easy-to-read values of practical utility, are reported. Mean values of uracil, β-pseudouridine, cytidine, hypoxanthine, xanthine, uridine, inosine, thymidine, and orotic acid (Fig. 1, panel A) ranged between 0.11 μmol/l AF (thymidine) and 1.89 μmol/l AF (uridine), being much lower in AF than in plasma of adults. Differently, creatinine (54.69 ± 13.86 μmol/l AF) and uric acid (238.35 ± 76.31 μmol/l AF) had the highest mean values in AF (Fig. 1, panel B) and were similar to values found in plasma of adults [14–16]. No similarities were observed when comparing the concentrations of purines, pyrimidines, creatinine, MA, MMA, and NAA recorded in AF with those measured in urine [14–16]. To evaluate whether the age of the donors might influence the concentrations in AF of the compounds under evaluation, we divided pregnant women into three different subgroups (30–34, 35–39, 40–44 years). Comparison of these three subgroups (ANOVA) did not reveal significant differences in any of the aforementioned compounds (data not shown).
Concentrations of purines, pyrimidines and creatinine in AF of actually normal pregnancies. Each histogram is the mean value of 1,257 AF samples. Standard deviations are represented by vertical bars. For the sake of clarity, different compounds were divided in the two Panels on the basis of their concentrations in AF (panel A, compounds with low concentration; panel B, compounds with high concentration)
Preliminarily to start the assay of amino group-containing compounds in AF samples, we have performed a validation of the HPLC method used to separate and quantify standard and non-standard amino acids and other NH2-containing compounds, by determining lowest limit of detection (LOD), LOQ, linearity, reproducibility, and recovery. Table 4 summarizes the values of LOD (evaluated with a signal to noise ratio >3), LOQ (calculated with a signal to noise ratio >5), and linearity of the various compounds considered. Values of LOQ were comprised between 0.01 and 0.08 μmol/l, i.e., between 0.25 and 2 nmol/25 μl injected, indicating a good sensitivity of the method for any of the 29 amino compounds considered. Linearity was performed by injecting standard mixtures with the following concentrations: LOQ, 100× LOQ, 500× LOQ, 1000× LOQ, and 5000× LOQ. Results showed that the correlation coefficients of the various compounds ranged between 1 and 0.995, indicating a high linearity of the method in the concentration interval tested. Reproducibility of the method was verified by calculating the coefficients of variation (CV) for both retention times and peak areas of the same standard mixture injected consecutively for five times (intra-assay CVs), as well as of five different standard mixtures injected in five consecutive days (inter-assay CVs). Results (data not shown) indicated minimal intra-assay variations of both peak areas and retention times (CVs ranged between 0.03 and 0.4% for retention times and between 0.2 and 0.6% for peak areas), as well as minimal inter-assay variations of both peak areas and retention times (CVs ranged between 0.06 and 0.8% for retention times and between 0.4 and 1.2% for peak areas). Recovery of the various compounds of interest was carried out by spiking five different AF samples with a standard mixture with known concentration. These spiked samples were processed for protein removal and then assayed by HPLC. Recovery of the amino compounds (data not shown) ranged between 95% (NH3) and 103% (SAM).
After having evaluated its suitability, the method to assay amino compounds was successfully applied to determine their concentrations in the same AF samples previously assayed for purines, pyrimidines, creatinine, MA, MMA, and NAA. Figure 2 reports mean values ± S.D. of 29 amino group-containing compounds measured in 1,257 normal AF. Confidence intervals at the 95% level, approximated for the sake of readability and practical utility, are summarized in Table 5. In our normal AF, Asp, Arg-succ, AAAA, Ser, SAM, Citr, Arg, Carn, GABA, SAH, Cystat, Met, NH3, Trp, Ile, and Orn had values below 40 μmol/l AF (minimal and maximal mean values for these compounds = 0.78 μmol/l AF for AAAA and 36.29 μmol/l AF for Arg) (Fig. 2, panel A). Differently, Glu, Asn, Gln, His, Gly, Thr, Ala, Tau, Tyr, Val, Phe, Leu, and Lys had values higher than 50 μmol/l AF (minimal and maximal mean values for these compounds = 51.78 μmol/l AF for Asn and 401.10 μmol/l AF for Ala) (Fig. 2, panel B). These 29 amino compounds were detectable in all AF samples analyzed. The comparison of values we found in AF with those recorded in plasma/serum of adults [17] showed that several standard amino acids and amino compounds (Asp, Asn, Gln, His, Gly, Ala, Tau, Tyr, Val, Met, Phe, Leu, Lys) have similar mean values and range intervals in these two body fluids. Viceversa, comparison in the amino acidic and amino compound compositions of AF and urine [17] did not evidence particular correspondences. To evaluate whether the age of the donors might influence the concentrations in AF of the compounds under evaluation, we divided pregnant women into three different subgroups (30–34, 35–39, 40–44 years). Comparison of these three subgroups (ANOVA) did not reveal significant differences in any of the aforementioned compounds (data not shown).
Concentrations of amino group-containing compounds (including standard and non-standard amino acids) in AF of actually normal pregnancies. Each histogram is the mean value of 1,257 AF samples. Standard deviations are represented by vertical bars. For the sake of clarity, different compounds were divided in the two Panels on the basis of their concentrations in AF (panel A, compounds with low concentration; panel B, compounds with high concentration)
Results of the metabolic prenatal screening with the description of an occasional case report
In the second part of the study (started in June 2010), thanks to the reference values of the metabolites of interest determined in 1,257 AF of actually normal pregnancies, we started a large-scale screening in all consenting women who carried out amniocentesis for cytogenetic-based diagnosis (i.e., including in the study a sample population with unknown risk for IEM). The aim was to evidence eventual anomalous metabolic profiles in AF samples possibly related to IEM, using the same criteria adopted in the neonatal screenings. During the period between June 2010 and February 2011, 1,681 additional AF samples were analyzed to determine their concentrations in purines, pyrimidines, creatinine, MA, MMA, NAA, and amino group-containing compounds. For 313 of these AF, the follow-up to have information about pregnancies, labors, deliveries, and offspring could not be effected. Fifty-nine samples were not assayed because mothers were carrying fetuses suffering from morphological anomalies, growth retardation, and any cytogenetic disorder different from Down’s syndrome. Fourteen AF were from donors carrying fetuses affected by Down’s syndrome and were treated as a separate group of data, in combination with the ten AF samples with the same genetic pathology found in the first part of the study. The remaining 1,295 AF samples were again from actually normal pregnancies. According to the data reported in Tables 6 and 7, the metabolic profiles detected in these additional 1,295 normal AF clearly reflected those found in the first part of the study, in the first set of 1,257 normal AF. It is worth stressing that no one of these additional 1,295 samples from actually normal pregnancies had any value of the 45 compounds under evaluation falling outside its respective confidence interval calculated in the first part of the study and reported in Tables 3 and 5. Therefore, also in these AF, cytidine, thymine, inosine, guanosine, MA, MMA, and NAA were undetectable with the present method, and the 29 amino compounds were detectable in all samples analyzed. Statistical comparison between the metabolic profiles of the two sets of 1,257 and 1,295 AF samples did not reveal any significant difference in any of the metabolites under evaluation.
As previously indicated, among the 1,472 AF samples analyzed in the first part of the study, ten were obtained from donors carrying Down’s syndrome-affected fetuses. Results of these ten samples were combined with those obtained in the 14 AF samples from donors carrying fetuses affected by the same genetic disorder and found in the second part of the study. Multivariate analysis indicated that purines and pyrimidines in these 24 AF samples had values comparable to those recorded in AF from normal fetuses. This suggests that no appreciable alteration in energy metabolism, nucleic acid turnover, or rate of protein biosynthesis, capable to cause modifications of purines, pyrimidines, creatinine, MA, MMA and NAA in AF, occurs during the development of fetuses affected by this pathological condition. Differently, multivariate analysis indicated that the profile in amino group-containing compounds of AF from Down’s syndrome-affected fetuses was different from that of AF from actually normal pregnancies. In particular, mean values of Glu, Gln, Gly, Tau, Val, Ile, Leu, Orn, and Lys were significantly different from corresponding mean values recorded in control AF samples (z-test, P < 0.05), as illustrated in Fig. 3. It is worth underlining that the value of Glu in all these pathological AF samples was outside the lower limit of its CI (Table 5), while the value of Gln was outside the upper limit of its CI (Table 5).
Concentrations of Glu, Gln, Gly, Tau, Val Ile, Leu, Orn, and Lys in AF of actually normal pregnancies (controls) and in AF from Down’s syndrome-affected fetuses. Each histogram is the mean value of 1,257 (controls) or 24 (Down’s syndrome-affected fetuses) AF samples. Standard deviations are represented by vertical bars. In comparison with controls, all these amino acids had significantly different values in AF from Down’s syndrome-affected fetuses (P < 0.05)
In an apparently clinically healthy woman of 33 years of age, withdrawal of AF was performed at the 16th week of gestation. Results of all morphological, echographic, and cytogenetic analyses were negative, strongly indicating a normal pregnancy. As a standardized procedure, once received the informed consent of the woman, an aliquot of AF was processed and assayed to determine the concentrations of the compounds of interest. All metabolites were in the normal range with the exception of Phe, the concentration of which was 375.54 μmol/l, i.e., 5.77 times higher than the mean value (65.04 μmol/l) detected in our 1,257 normal AF (see Table 5). Before undertaking further analysis on the fetus, we determined Phe concentration in maternal serum. A value of 360.88 μmol/l serum (equaling Phe found in AF) induced us to hypothesize a case of maternal mild hyperphenylalaninemia (MHPA). Results of the subsequent molecular analyses of the coding and splicing regions of the phenylalanine hydroxylase (PAH) gene carried out on the cDNA extracted from peripheral blood of both parents, as well as on the fetal cDNA extracted from amniocytes, indicated that: (i) the father was carrying in heterozygosis the missense mutation V230I; (ii) the mother was affected by phenylketonuria (PKU) (OMIM 261600) with mild hyperphenylalaninemia (MHPA), carrying in heterozygosis the two mutations IVS 4 + 5 G–T and D394A; (iii) the fetus was carrying in heterozygosis the maternal D394A mutation. During the remaining period of the pregnancy, a low-Phe diet normalized maternal serum Phe (below 120 μmol/l), therefore avoiding any fetal developmental anomaly. The neonate was born healthy.
Discussion
The fundamental importance of metabolic neonatal screenings for an early diagnosis of IEM, particularly when the IEM-associated symptoms can be nutritionally and/or pharmacologically ameliorated or even abolished, is nowadays a well-acquired concept. Differently, its relevance in the prenatal setting has still to be established. Studies performed with this aim have been conducted either in selected populations of pregnant women at risk for some specific IEM [7, 13] or in a relatively limited number of samples [11, 12, 18], or by measuring a restricted number of analytes [19]. To the best of our knowledge, no results have been reported to date in which metabolic prenatal screenings have been carried out with the same criteria used in the metabolic neonatal screenings: (i) simultaneous determination of several metabolites to obtain a broad spectrum of potentially diagnosable IEM; (ii) high number of samples screened in a population with unknown risk for IEM.
To carry out screening using the aforementioned criteria, reliable reference values of the metabolites under evaluation in AF from actually normal pregnancies must be available. Thanks to the largest sample size of AF from actually normal pregnancies reported to date (n = 1,257), the present study produced suitable reference values for a relatively high number of compounds (n = 45) of different chemical classes (purines, pyrimidines, creatinine, MA, MMA, NAA, standard and non-standard amino acids, and additional amino compounds). In the first part of the study, this allowed to calculate 95% confidence intervals also for those compounds related to several relevant IEM (purines and pyrimidines) for which valid ranges of variability were still unavailable (Tables 3, 5). According to the data obtained during the second part of this study, additional 1,295 AF from actually normal pregnancies gave results for the various metabolites always falling within the reference values illustrated in Tables 3 and 5. This striking result strongly reinforces the validity of our reference values that can reliably be used to monitor the metabolic profile of unknown samples, at least for the 45 compounds considered in this study. Altogether, this set of 2,552 AF assayed represents the largest sample size ever reported in literature in which the metabolic profile, including 45 compounds of different chemical classes, was characterized.
The metabonomic approach to characterize AF composition indicated that fetal anomalies may be accompanied by alterations in the AF metabolic profile [11, 12]. During the first and second part of our study, we had the opportunity to analyze also 24 AF from Down’s syndrome-affected fetuses. Our results (Fig. 3) evidenced that all these specific pathological AF had concentrations of Glu and Gln falling outside the lowest and the highest value, respectively, of their corresponding confidence intervals determined in normal AF. Consequently, a ninefold decrease in the Glu/Gln ratio (0.083) with respect to the value determined in AF from normal fetuses (0.76) was observed (P < 0.001). Since excreted glutamate is also derived by the renal deamination of glutamine, it may be hypothesized that Down’s syndrome-affected fetuses suffer from delayed renal development that ultimately causes decrease in the Glu/Gln ratio in AF. It might also be supposed that if the decrease in the fetal availability of glutamate is persistent throughout the whole period of fetal development, it is responsible for some of the neuronal disturbances characteristics of this pathological state. Furthermore, the analysis of the full amino acid profile revealed significant decrease in the values of several essential amino acids (Val, Ile, Leu, and Lys), as well as in the concentrations of Gly, Tau, and Orn (Fig. 3). The concomitant reduction in the AF levels of Gly and Glu, both involved in the neurotransmission processes, might have cumulative negative effects on the fetal neuronal functions. According to our knowledge, we here reported the largest sample size of AF from Down’s syndrome-affected fetuses ever described, in which the metabolic profile was characterized.
Although it was certainly not in the scope of the screening to diagnose for maternal diseases, the case report of the woman unaware to be affected by PKU with MHPA described in this study, certainly rare and incidentally found, was evidenced just thanks to this metabolic prenatal screening. In fact, the analysis of her AF sample allowed to show abnormally elevated Phe (375.54 μmol/l AF, about six times higher than the value in controls), thus inducing us to carry out the determination of Phe in maternal serum. According to the data in literature, the level of Phe we found in this AF sample, which was equal to that in the maternal blood, was sufficient to cause abnormal fetal development and irreversible damage to newborns [20–22]. Thanks to the anomalous result in the AF metabolic profile, the mother was biochemically and genetically diagnosed. A low-Phe diet allowed her serum Phe to remain within non pathologic limits, i.e. below 120 μmol/l during pregnancy [23–25] ensuring normal fetal development and no postnatal deficit. The heterozygoticity of the fetus could also be evidenced.
In conclusion, this study reports reliable reference values for a relevant number of compounds of various chemical classes. Although limited to fetuses suffering from the Downs’ syndrome, our results strongly corroborate the correlation between anomalies in the metabolic profile of AF and the fetal development [11, 12, 26]. Furthermore, our data suggest that the metabolic profile of AF and the approach adopted in the metabolic neonatal screenings (broad spectrum of metabolites analyzed, large size of samples screened in a sample population with unknown risk for IEM) may be adopted as additional biochemical test in amniocenteses, useful to highlight anomalies potentially related to IEM. To this purpose, our physiologic reference values obtained in AF from actually normal pregnancies appear to be valuable to evidence anomalous AF biochemical profiles potentially caused by an IEM. The occasional case report here described would seem to indicate that the analysis of the metabolic profile of AF may provide additional information otherwise not detectable with different laboratory and clinical tests. It should also be recalled that the HPLC methods and the sample preparation adopted can successfully be applied to large-scale screenings, since they are characterized by relative analytical rapidity, ease of sample preparation and methods of analyses and, additionally, by low analytical costs, thereby presenting unquestionable advantages with respect to other analytical approaches [11, 12, 26].
Limitations of the study
It is worth underlining that the case report here illustrated was not a case of fetal IEM. In addition, data presented does not support indications on the diagnostic performance of the prenatal screening (percent of false positives and false negatives). Even though a much larger sample size is required before these information are available, we would like to remind that to date neither false positive nor false negative were recorded in these 1,681 AF samples screened. It should also be recalled that other classes of compounds, such as organic acids and acyl-carnitines, might be assayed in AF to have a broader spectrum of IEM potentially diagnosable. To fulfill these shortages, the prenatal screening through the metabolic analysis of AF is still in progress.
Abbreviations
- Ala:
-
Alanine
- AAAA:
-
α-aminoadipic acid
- AF:
-
Amniotic fluid
- Arg:
-
Arginine
- Arg-succ:
-
Argininosuccinate
- Asn:
-
Asparagine
- Asp:
-
Aspartate
- Carn:
-
Carnosine
- CI:
-
Confidence interval
- Cit:
-
Citrulline
- CV:
-
Coefficient of variation
- Cystat:
-
l-Cystathionine
- GABA:
-
γ-Aminobutyrate
- Gln:
-
Glutamine
- Glu:
-
Glutamate
- Gly:
-
Glycine
- HPLC:
-
High-performance liquid chromatography
- His:
-
Histidine
- IEM:
-
Inborn errors of metabolism
- Ile:
-
Isoleucine
- ISTD:
-
Internal standard
- Leu:
-
Leucine
- LOD:
-
Lowest limit of detection
- LOQ:
-
Lowest limit of quantification
- Lys:
-
Lysine
- MA:
-
Malonic acid
- Met:
-
Methionine
- MHPA:
-
Mild hyperphenylalaninemia
- MMA:
-
Methylmalonic acid
- MPA:
-
3-Mercaptopropionic acid
- NAA:
-
N-acetylaspartate
- Norval:
-
Norvaline
- OPA:
-
Ortophtalaldehyde
- Orn:
-
Ornithine
- PAH:
-
Phenylalanine hydroxylase
- Phe:
-
Phenylalanine
- PKU:
-
Phenylketonuria
- SAH:
-
S-adenosylhomocysteine
- SAM:
-
S-adenosylmethionine
- Ser:
-
Serine
- Tau:
-
Taurine
- Thr:
-
Threonine
- Trp:
-
Tryptophane
- Tyr:
-
Tyrosine
- Val:
-
Valine
References
Jones PM, Bennett MJ (2010) Urine organic acid analysis for inherited metabolic disease using gas chromatography-mass spectrometry. Methods Mol Biol 603:423–431
Pitt JJ, Eggington M, Kahler SG (2002) Comprehensive screening of urine samples for inborn errors of metabolism by electrospray tandem mass spectrometry. Clin Chem 48:1970–1980
Simoni RE, Gomes LN, Scalco FB, Oliveira CP, Aquino Neto FR, de Oliveira M (2007) Uric acid changes in urine and plasma: an effective tool in screening for purine inborn errors of metabolism and other pathological conditions. J Inherit Metab Dis 3:295–309
Geelhoed EA, Lewis B, Hounsome D, O’leary P (2005) Economic evaluation of neonatal screening for phenylketonuria and congenital hypothyroidism. J Paediatr Child Health 41:575–579
Gao T, Zablith NR, Burns DH, Skinner CD, Koski KG (2008) Second trimester amniotic fluid transferrin and uric acid predict infant birth outcomes. Prenat Diagn 28:810–814
Gao T, Zablith N, Burns DH, Koski KG, Skinner CD (2009) Identification and quantitation of human amniotic fluid components using capillary zone electrophoresis. Anal Biochem 388:155–157
Inoue Y, Ohse M, Shinka T, Kuhara T (2008) Prenatal diagnosis of propionic acidemia by measuring methylcitric acid in dried amniotic fluid on filter paper using GC/MS. J Chromatogr B 870:160–163
López Ramón Y, Cajal C, Ocampo Martínez R, Couceiro Naveira E, Martínez M (2007) Amino acids in amniotic fluid in the 15th–16th weeks of gestation and preterm labor. J Matern Fetal Neonatal Med 20:225–231
Oliveira FR, Barros EG, Magalhães JA (2002) Biochemical profile of amniotic fluid for the assessment of fetal and renal development. Braz J Med Biol Res 35:215–222
Cohn BR, Joe BN, Zhao S, Kornak J, Zhang VY, Iman R, Kurhanewicz J, Vahidi K, Yu J, Caughey AB, Swanson MG (2009) Quantitative metabolic profiles of 2nd and 3rd trimester human amniotic fluid using (1)H HR-MAS spectroscopy. MAGMA 22:343–352
Graça G, Duarte IF, Goodfellow BJ, Carreira IM, Couceiro AB, Domingues Mdo R, Spraul M, Tseng LH, Gil AM (2008) Metabolite profiling of human amniotic fluid by hyphenated nuclear magnetic resonance spectroscopy. Anal Chem 80:6085–6092
Graça G, Duarte IF, Barros AS, Goodfellow BJ, Diaz S, Carreira IM, Couceiro AB, Galhano E, Gil AM (2009) (1)H NMR based metabonomics of human amniotic fluid for the metabolic characterization of fetus malformations. J Proteome Res 8:4144–4150
Ottolenghi C, Abermil N, Lescoat A, Aupetit J, Beaugendre O, Morichon-Delvallez N, Ricquier D, Chadefaux-Vekemans B, Rabier D (2010) Gestational age-related reference values for amniotic fluid organic acids. Prenat Diagn 30:43–48
Tavazzi B, Lazzarino G, Leone P, Amorini AM, Bellia F, Janson CG, Di Pietro V, Ceccarelli L, Donzelli S, Francis JS, Giardina B (2005) Simultaneous high performance liquid chromatographic separation of purines, pyrimidines, N-acetylated amino acids, and dicarboxylic acids for the chemical diagnosis of inborn errors of metabolism. Clin Biochem 38:997–1008
Friedecky D, Adam T, Bartak P (2002) Capillary electrophoresis for detection of inherited disorders of purine and pyrimidine metabolism: a selective approach. Electrophoresis 23:565–571
Vidotto C, Fousert D, Akkermann M, Griesmacher A, Müller MM (2003) Purine and pyrimidine metabolites in children’s urine. Clin Chim Acta 335:27–32
Waterval WA, Scheijen JL, Ortmans-Ploemen MM, Habets-van der Poel CD, Bierau J (2009) Quantitative UPLC-MS/MS analysis of underivatised amino acids in body fluids is a reliable tool for the diagnosis and follow-up of patients with inborn errors of metabolism. Clin Chim Acta 407:35–42
Monsen AL, Schneede J, Ueland PM (2006) Mid-trimester amniotic fluid methionine concentrations: a predictor of birth weight and length. Metabolism 55:1186–1191
Amaral C, Gallardo E, Rodrigues R, Pinto Leite R, Quelhas D, Tomaz C, Cardoso ML (2010) Quantitative analysis of five sterols in amniotic fluid by GC-MS: application to the diagnosis of cholesterol biosynthesis defects. J Chromatogr B 878:2130–2136
Bouchlariotou S, Tsikouras P, Maroulis G (2009) Undiagnosed maternal phenylketonuria: own clinical experience and literature review. J Matern Fetal Neonatal Med 22:943–948
Brass CA, Isaacs CE, McChesney R, Greengard O (1982) The effects of hyperphenylalaninemia on fetal development: a new animal model of maternal phenylketonuria. Pediatr Res 16:388–394
Rouse B, Azen C (2004) Effect of high maternal blood phenylalanine on offspring congenital anomalies and developmental outcome at ages 4 and 6 years: the importance of strict dietary control preconception and throughout pregnancy. J Pediatr 144:235–239
Fekkes D, van Dalen A, Edelman M, Voskuilen A (1995) Validation of the determination of amino acids in plasma by high-performance liquid chromatography using automated pre-column derivatization with o-phthaldialdehyde. J Chromatogr B 669:177–186
Hilton MA (1982) Liquid-chromatographic direct determination of phenylalanine and tyrosine in serum or plasma, with application to patients with phenylketonuria. Clin Chem 28:1215–1218
Zhang LF, Yu YL, Yang RY (1983) Direct determination of phenylalanine in serum extracts of phenylketonuria patients by reversed-phase high-performance liquid chromatography. J Chromatogr 282:333–339
Graca G, Duarte IF, Barros AS, Goodfellow BJ, Diaz SO, Pinto J, Carreira IM, Galhano E, Pita C, Gil AM (2010) The impact of prenatal disorders on the metabolic profile of 2nd trimester amniotic fluid: a nuclear magnetic resonance (NMR) metabonomic study. J Proteome Res 9:6016–6024
Acknowledgments
This study was supported in part by research funds of the University of Catania. A special thank to Dr. Roberto Tozzi for his significant contribution in the statistical analysis of data.
Author information
Authors and Affiliations
Corresponding author
Rights and permissions
About this article
Cite this article
Amorini, A.M., Giorlandino, C., Longo, S. et al. Metabolic profile of amniotic fluid as a biochemical tool to screen for inborn errors of metabolism and fetal anomalies. Mol Cell Biochem 359, 205–216 (2012). https://doi.org/10.1007/s11010-011-1015-y
Received:
Accepted:
Published:
Issue Date:
DOI: https://doi.org/10.1007/s11010-011-1015-y