Abstract
Vegetable oils with variable proportions of oleic, linoleic, and linolenic acids are more susceptible to oxidative processes. In this subject, this study evaluates the physical chemical properties and oxidative stability of non-conventional oils such as andiroba, babassu, sesame, oiticica, jatropha, and grape through accelerated oxidation techniques (pressurized differential scanning calorimetry, Rancimat and PetroOxy). It was verified that babassu and andiroba oil do not showed detectable induction period presenting high oxidative stability; moreover, it was observed that the enthalpic events occurred in 1.19, >10, 0.53, 0.49, 0.49, and 0.60 h for the andiroba oil, babassu oil, sesame seeds, jatropha, oiticica oils, and grapes, respectively, stimulating the conclusion of greater stability for the babassu oil.
Similar content being viewed by others
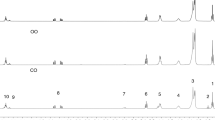
Explore related subjects
Discover the latest articles, news and stories from top researchers in related subjects.Avoid common mistakes on your manuscript.
Introduction
Among the sources of available biomass appropriate for consolidation of renewable energy programs, vegetable oils and animal fats appear as an excellent alternative for substitution of mineral diesel [1–3]. Oils and fats undergo a transesterification process which results in biodiesel production [4]. In Brazil, several oleaginous species are cultivated, such as the soybean, castor oil plant, sunflower, and corn, which have the potential for their usage as raw materials for biodiesel production [5].
The viability of each oilseed for biofuel production depends on its technical competitiveness, economic and socio-environmental [6]. In view of the great diversity of oil for biofuel production has stood on Glycine max (soybean), Helianthus annuus (sunflower), Ricinus communis (castor), Gossypium hirsutum (cotton). In addition, a series of oil seeds, such as Jatropha curcas, Licania rigid benth (oiticica), Sesamum indicum (sesame), Carapa guianensis (andiroba) Orbignya phalerata (babassu) Vitis sp (grape) and others, are being investigated in respect to physicochemical analysis showing preliminary the state of preservation, degree of unsaturation, and degree of oxidation of vegetable oils.
Oxidative degradation is directly related to the availability of air, temperature and the presence of unsaturated oils [7], such that the speed of the oxidation reactions for these molecules depend on the number and position of double bonds [8]. Bis-allylic positions in the fatty acid chains are effectively more susceptible to auto-oxidation reactions dropping the thermal stability of vegetable oils due to the decrease in temperature and the oxidation induction time—OIT, changing sensory factors, color and increasing viscosity [9].
This study evaluates the physicochemical properties and oxidative stability of non-conventional oil: andiroba, babassu, sesame, oiticica oils, jatropha, and grape seed through accelerated oxidation techniques, pressurized differential scanning calorimetry (PDSC) [10, 11], Rancimat and PetroOxy, with the aim of proposing alternative sources of oils suitable for biofuel production.
Materials and methods
Materials
Babassu, oiticica, jatropha, and sesame oils were obtained directly by cold pressing in a mechanical press adapted trademark Ribeiro, with maximum pressure of 30 t. After extraction, the oils were vacuum filtered and dried at 60 °C in an oven. Andiroba and grape seed oils were obtained commercially, trademark Vegetable Union.
Physicochemical characterization
The analyses of acid number, peroxide and iodine were performed according to [12, 13].
Chromatographic identification
The derivatization of the oils, the method described by [14], has become necessary to obtain the chromatographic data. The identification of the ester was obtained by gas chromatography coupled with mass spectrometry (GC–MS) equipped with split injector (1:50) from Shimadzu, model GCMS-QP 2010 with auto sampler and injection volume 1 μL. DB-23 capillary column (30 m × 0.25 mm × 0.25 mm) loaded with 50 % cyanopropyl-metilpolisiloxano as a stationary phase and helium as carrier gas at a flow rate of 96 mL min−1.
Viscosity assay
The kinematic viscosity was determined according to ABNT NBR 10441. We used a kinematic viscometer manual trademark Julabo, model ME 18 V, at 40 °C temperature.
Oxidative stability
Rancimat
The oxidative stability of oils was determined according to AOCS Cd 12b-92 and 14 112 EN in a Rancimat equipment trademark Metrohm model 743. 3 g of the sample was aged under constant air flow of 10 L h−1 at 110 °C temperature. The volatiles were drawn into the oxidation vessel containing deionized water where the conductivity is measured. The time elapsed until detection of a sudden increase in conductivity is called induction period—PI.
PetroOxy
The oxidative stability was determined by using the apparatus PetroOxy Petrotest. 5 mL of the sample was analyzed, under initial pressure of pure oxygen to 700 kPa at room temperature. As stabilized pressure, the temperature was raised to 110 °C. The induction period is given as the time required for the sample absorbs 10 % of available oxygen pressure for the test.
Pressurized differential scanning calorimetry (PDSC)
PDSC curves were obtained using a differential calorimeter exploratory apparatus, coupled to a pressure cell, model DSC 2920 from TA Instruments, under dynamic and isothermal analyses conditions. Analyses were processed in dynamic mode using a platinum crucible. 5 mg of the sample was used under pressure of 1400 kPa of oxygen atmosphere and with a heating rate of 10 °C min−1, and 25–600 °C temperature range.
Results and discussion
Physicochemical characterization
Table 1 shows the characterization of the oils. It can be seen that for the acidity index, the jatropha and grape seed oils do not need a process of neutralization prior transesterification, since they showed values of 0.98 and 0.64 mg KOH g−1, respectively. The prerequisite to biofuel production is an acidity index equal to or less than 1.0 mg KOH g−1, to prevent saponification reaction [15].
The peroxide index, which estimates the degree of degradation of the raw material, reveals that the selected samples exhibits light peroxides index which refers to suitable oils for either purposes food or synthesis of biodiesel. Exceptions are the andiroba and oiticica oils.
The iodine index of the oils is related to the level of unsaturation of the chains, so it appears that the sesame oil, oiticica, jatropha, and grape seed exhibit elevated levels of unsaturation when compared with babassu and andiroba oils [16].
Table 2 shows the different fatty acid compositions of the studied oils. It is stressed the predominance of unsaturated acids in andiroba, sesame, oiticica, jatropha and grape seed oils, particularly the linoleic and linolenic acids. Babassu oil is composed mainly of saturated fatty acids, with the predominance of lauric acid. The chemical composition of the oils directly from corroborates the obtained iodine index.
It is well known that the chain length, degree of unsaturation and molecular interactions can affect the viscosity. Unsaturated fatty acids in cis configuration prevent the alignment of these molecules, resulting in weaker intermolecular interactions and hence lower viscosity [17].Consequently, grape seed oil with high percentage of linoleic acid has lower viscosity than andiroba and oiticica oils and babassu oil shows low viscosity because the composition of saturated fatty oils and shorter length of the chains.
According to Rancimat results it is noted the high oxidative stability of babassu and andiroba oils with no detectable PI, Table 3. Ever since even after the end of 30 h, no sudden variation in conductivity of the water, representative of the presence of volatile organic compounds due to oxidation. Jatropha oil also showed high oxidative stability of 12.36 h, probably because the presence of 44 % of monounsaturated fatty acids and 18 % of saturated fatty acids. In contrast, sesame, grape seed and oiticica oils show low oxidative stability, with the induction period of only 2.01, 0.06, and 1.11 h, respectively, which can be attributed to higher percentages of polyunsaturated fatty acids in composition. Thus, the high oxidative stability of babassu oil correlates with high content of saturated fatty acids present.
Results from Rancimat and PetroOxy PI tests showed consistency. It is noteworthy that the oxidative degradation of oils in PetroOxy test show lower PI than those obtained by the Rancimat. This fact can be attributed to the use of O2 pressure on the PetroOxy, as additional component, to accelerate the oxidation and reduce the time of analysis.
The PDSC curves, Fig. 1a,b, were obtained in dynamic mode, in which 110 °C temperature was provided to carry out the analysis of the samples in an isothermal mode. Results of oxidation induction of the oils showed the initial stages of oxidation from enthalpic events arising from the abstraction of hydrogens of the unsaturated chain of the fatty acid.
According to this analysis there was the first event of enthalpy to andiroba, babassu, sesame, jatropha, oiticica, and grape seed oils, respectively, for 1.19, >10, 0.53, 0.49, 0.49, and 0.60 h. This explains the increased stability for babassu oil, in accordance with its composition.
Conclusions
Vegetable oils from jatropha and grape seeds showed values of acidity index lower than 1.0 mg KOH g−1 oil. Other oils such as andiroba, babassu, sesame, and oiticica showed acidity index values higher than that, emphasizing the need to prior treatment to fix this parameter in order to be used as a matrix in the production of biodiesel.
The analysis of thermal and oxidative stability shows andiroba, babassu, and jatropha oils more resistant to oxidation, with higher values of OIT (PDSC) and PI (RANCIMAT) over six hours after all. Data related from PetroOxy also shown to be consistent, showing higher PI values for these oils. Thermal and oxidative stability shows that andiroba, babassu, and jatropha oils presented better potential for biodiesel production.
References
Silva MCD, Silva LM, Santos NA, Conceicão MM, Souza AG, Santos IMG. Study of ethylic Babassu biodiesel properties at low temperatures. J Therm Anal Calorim. 2011. doi:10.1007/s1097301114740.
Knothe G, Krahl J, Van Gerpen J. The biodiesel handbook. 1st ed. Champaign: AOCS Press; 2005.
Rodrigues FMG, Souza AG, Santos IMG, Bicudo TC, Silva MCD, Sinfrônio FSM, Vasconselos AFF. Antioxidative properties of hydrogenated cardanol for cotton biodiesel by PDSC and UV/Vis. J Therm Anal Calorim. 2009;97:605.
Ferrari RA, Oliveira VS, Scabio A. Biodiesel de soja—taxa de conversão em ésteres etílicos, caracterização físicoquímica e consumo em gerador de energia. Quim Nova. 2005;28:19–23.
Dantas MB, Almeida AAF, Conceição MM, Fernades VJ Jr, Santos IMG, Silva FC, Soledade LEB, Souza AG. Characterization and kinectic compensation effect of corn biodiesel. J Therm Anal Calorim. 2007;87:847–51.
Ferrari RA, Oliveira VS, Scabio A. Oxidative stability of biodiesel from soybean oil ethyl esters. Sci Agric. 2005;62:291–5.
Bailey AE. Industrial oil and fat products. 5th ed. New York: Wiley; 1996.
Freedman BE, Bagby MO. Heat of combustion of fatty esters and triglycerides. J Therm Anal Calorim. 1989;66:1601–5.
Lôbo IP, Ferreira SLC, Cruz RS. Biodiesel: parâmetros de qualidade e métodos analíticos. Quím Nova. 2009;32:1604.
Melo MLS, Santos NA, Rosenhaim R, Souza AG, Athayde Filho PF. Use of thermal analysis techniques for evaluation of the stability and chemical properties of papaya biodiesel (Carica Papaya L.) at Low Temperatures. J Therm Anal Calorim. 2011. doi:10.1007/s1097301118616.
Conceicão MM, Dantas MB, Rosenhaim R, Fernandes VJJR, Santos IMG, Souza AG. Evaluation of the oxidative induction time of the ethilic castor biodiesel. J Therm Anal Calorim. 2000;97:643–6.
AOCS—American Oil Chemists Society. Official methods and recommended practices of the AOCS. 5th ed. Champaign: AOCS; 1999.
Zenebon O, Pascuet NS, Tiglea P, Normas Analíticas do Instituto Adolfo Lutz. Métodos físico-químicos para análise de alimentos. 4th ed. São Paulo: Instituto Adolfo Lutz; 2008.
Nascimento RJS, Couri S, Antoniassi R, Freitas SP. Composição em ácidos graxos do óleo da polpa de açaí extraído com enzimas e com hexano. Rev Bras Frutic. 2008. doi:10.1590/s0100-29452008000200040.
Knothe G. Some aspects of biodiesel oxidative stability. Fuel Process Technol. 2007;88:669–77.
Ramalho EFSM, Albuquerque AR, Souza AL, Barro AK, Maia AS, Santos IMG, Souza AG. Use of different techniques in the evaluation of the oxidative stability of poultry fat biodiesel. J Therm Anal Calorim. 2011;106:787–91.
Hui YH. Handbook of food science technology, and engineering, vol. 1. Boca Raton: CRC Press; 2006. p. 1000.
Author information
Authors and Affiliations
Corresponding author
Rights and permissions
About this article
Cite this article
Melo, M.A.M.F., de Melo, M.A.R., Pontes, A.S.G.C. et al. Non-conventional oils for biodiesel production: a study of thermal and oxidative stability. J Therm Anal Calorim 117, 845–849 (2014). https://doi.org/10.1007/s10973-014-3825-0
Received:
Accepted:
Published:
Issue Date:
DOI: https://doi.org/10.1007/s10973-014-3825-0