Abstract
Removal of heavy metals from aqueous solutions has attracted much attention worldwide. Many processes and technologies have been developed to remove heavy metals ions. In our previous study, a silica-poly acrylic amphoteric hybrid hydrogel was successfully prepared with double-network (DN) structure using γ-aminoporpyltriethoxysilane as precursor through a two-step sequential network formation technique. In the present research, the absorption behavior of this hydrogel was investigated carefully using Cu2+ ions and Cr2O 2−7 ions as representatives of negative and positive ions respectively. Under different adsorption conditions, the adsorption behaviors of the hydrogel were studied in detail, including initial concentration of the adsorbed ions, adsorption time, pH and ionic strength. The results showed the absorption capacity to Cu2+ can reach 700 mg/g with an initial Cu2+ concentration of 1,200 mg/L within 2 h. The experimental results show that the Freundlich adsorption law is applicable to the adsorption of Cu2+ and Cr2O 2−7 on the hydrogel.
Similar content being viewed by others
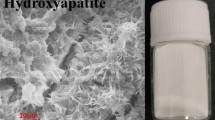
Explore related subjects
Discover the latest articles, news and stories from top researchers in related subjects.Avoid common mistakes on your manuscript.
Introduction
Removal of heavy metals from aqueous solutions has attracted scientists’ interest worldwide. Many processes and technologies have been developed and applied to remove heavy metal ions from water, such as precipitation or the use of an exchange resin, membrane filter or an absorbent. Among which, the use of absorbents is an effective method from both economic and technological viewpoints.
The industrial and biomedical applications of hydrogels made from either natural or synthetic sources are strongly limited by their poor mechanical properties [1]. Organic–inorganic hybrid hydrogels showed higher mechanical strength compared with traditional organic hydrogels [2–5]. Recently, a lot of work reported on preparation of hybrid hydrogel by introducing silica [6–8], however, in which the high mechanical strength is achieved by the sacrifice of swelling capacity [9]. To increase the mechanical strength of hydrogels, three kinds of hydrogels with unique structures and high mechanical strength have been developed. Slide-ring gels are topological (TP) networks [10, 11], Nanocomposite (NC) gels [12–15], and double-network (DN) materials generally consist of two independently cross-linked networks, these hydrogels apparently exhibit the best mechanical properties when the first network is highly cross-linked and the second only lightly cross-linked [10].
One of most important function with amphoteric hybrid hydrogel is to separate and recover both cation and anion from contaminated water via the electrostatic effect. Because it not only combines the advantages of organic and inorganic materials but also exhibits some distinguished properties, such as structural flexibility and thermal and mechanical stability. In our previous study (not published data), a silica-poly(acrylic acid) (PAA) amphoteric hybrid hydrogel was successfully prepared with DN structure using γ-aminoporpyltriethoxysilane as precursor through a two-step sequential network formation technique. The prepared DN hydrogel showed significant high mechanical strength. In this paper, the adsorptive capacities of the Silica-PAA amphoteric hybrid hydrogel with DN structure to cations and anions (Cu2+ and Cr2O 2−7 were chose as model ions) were investigated in a range of systems. The effects of various parameters such as ionic density, pH, and ionic strength were investigated.
Experimental
Materials
Hydrochloric acid (HCl), ammonium persulfate (APS), N,N′-methylenebisacrylamide (BIS) ammonium persulfate (APS) and NaOH were purchased from Tianjin Chemistry Reagent Factory (Tianjin, China). Sodium hydroxide (NaOH), Cupric sulfate (CuSO4) and Potassium dichromate (K2Cr2O7) were purchased from Tianjin Yonghao Fine Chemicals Co (Tianjin, China). Ltd. Acrylic acid (AA) was purchased from Chengdu Kelong Chemistry Reagent Factory (Chengdu, China). γ-aminoporpyltriethoxysilane were purchased from Hubei Wuda silicone material company (Hubei China). Sodium chloride (NaCl) was purchased from Beijing Chemical Reagent Company (Beijing, China). Deionized water was used in all experiments, and all other chemicals and solvents were of analytical grade and used without any further purification.
Preparation of DN hydrogels
The hydrogel was prepared by in-situ copolymerization of acrylic acid (AA) and tetramethoxysilane in solution. Facture as follow, γ-aminoporpyltriethoxysilane was dispersed in 0.01 wt.% acrylic acid (AA) solution under stirring for 40 min in an ice bath to make a uniform solution. The volume of tetramethoxysilane to 0.01 wt.% AA was kept at a ratio of 2:5 (v/v). And then designed γ-aminoporpyltriethoxysilane solution was added into 10 mL AA neutralized to a neutralization degree of 70 mol% with 30 wt.% NaOH solution in an ice bath before, and stirred for another 10 min to make solution even. Afterwards, 0.03 wt.% organic crosslinker N,N′-methylenebisacrylamide and 1 wt.% initiator ammonium persulfate (APS) both concentration relative to AA weight were added to the system, additional water was added to make all the volume equally, the solutions were stirred for another 5 min, the components of solutions was illustrated in Table 1. The solution was finally transferred into the sealed plastic tubes (interior size = 19 mm diameter × 150 mm length) and then was put in a constant temperature bath at 50 °C for 2 h. After polymerization, the resulting hydrogels were removed, lay aside for a night, then cut into small pieces, and soaked in a large amount of deionized water for at least 24 h in order to remove monomers and oligomers which were not actually incorporated into the hydrogels, and then hydrogels were soaked in ethanol to deprive the contained water. Finally, the prepared hydrogel was dried in an oven at 80 °C until a constant weight was obtained.
Adsorption behaviors under different conditions
The adsorption experiments of these hydrogel for heavy-metal ions were carried out in a series of beaker containing the 0.1 g prepared hydrogel and the 250 mL solution at the desired concentration of ions (Cu2+ and Cr2O 2−7 ), initial pH (adjusted by dilute hydrochloric acid and NaOH) and different ionic intensities in the atmosphere temperature. Over a short time scale, the hydrogels were removed and the concentrations of ions after the adsorption were analyzed by atomic adsorption devices (for Cu2+) or ultraviolet spectrophotometer (for Cr2O 2−7 ). The absorption capacity to Cu2+ and Cr2O 2−7 ions (mg/g) can be calculated using following equation:
Where V is the volume of solution, W is the weight of the Hydrogel(g), C1, C2 are the concentrations of ions before and after the adsorption, respectively (mg/L)[16].
The removal ratio of Cu2+ and Cr2O 2−7 (%) were determined by using the following equation:
Where W1 is the weight of ions before adsorption (g) and W2 is the weight of ions after adsorption (g)[17].
Results and discussion
The prepared hydrogels were characterized by Fourier transform infrared (FTIR) spectroscopy, transmission electron microscopy (TEM), and electronic universal material testing machine. The results showed two independently cross-linked networks were formatted, PAA and Silica. The inorganic networks play as the second network in DN structure preventing cracks from growing to the point of producing catastrophic failure of the material [10]. And more, the silica network also provided –NH2 groups which can increase the salt tolerance of hydrogels [18]. In this work, a series of hydrogels were prepared with different silica ratio, among which, product 3 can sustain highest compressive stress of 58 kPa with a water content of 99.00 wt.% (Table 1), and was chose to investigate its absorption behavior.
Adsorption kinetics
To systematically study the adsorption behavior of the hydrogel, at first 0.1 g Silica-PAA hydrogel was dispersing in 250 mL 3 mg/mL CuSO4 solution or 0.3 mg/mL K2Cr2O7 solution at room temperature. All solutions were used directly without adjusting pH value of solution. The amount of adsorption ions were monitored via time. Experimental results were illustrated in Fig. 1. It was observed that the adsorption amount increased via time gone, the adsorption equilibrium was gotten around 60 min to Cu2+ ion, and 120 min to Cr2O 2−7 ion. In this work, the amphoteric hydrogel was used, in which total amount of carboxylate group (provided by the first network, PAA) is much larger than that of amino group (provided by the second network, silica), so the absorption capacities of Cu2+ ion was much bigger than that of Cr2O 2−7 ion.
Effect of pH on the adsorption of hydrogel to Cu2+ and Cr2O 2−7 ions
At room temperature, 0.1 g hydrogel was dispersing in 100 mL of 3 mg/mL CuSO4 solution with a pH range of 2–4 or 0.3 mg/mL K2Cr2O7 solution with a pH range of 3–12. As obtained results (Fig. 2), to Cu2+ ion, the adsorption capacity of the hydrogel increased along with pH. At pH 2, adsorption for Cu2+ ion was 548 mg/mL, and when the pH 3.5, the max adsorption capacity of 690 mg/mL attained. It is due to that the higher the pH is, the more quantity of –COO− the solution have and the more Cu2+ will be adsorbed by the hydrogel through static attraction between –COO− and Cu2+. This result seems a little different from that using negatively charged hydrogel, in which the adsorption mechanism was only based on electrostatic interaction between negatively charged group and Cu2+ ion [19]. In which, at pH 2, adsorption for Cu2+ ion was negligible. In this study, the amino group was introduced into hydrogel, and partly contributed to the absorption capacity of hydrogel through coordination bond.
To Cr2O 2−7 ion, at pH 2, the highest amount of adsorption was gotten, reached at 19.1 mg/mL, and adsorption capacity decreased with pH value of solution increasing. At pH 7, the total amount of Cr2O 2−7 ion was only 2.2 mg/mL. There are two reasons: the first, when pH is low, the degree of dissociation of –COOH is little and there has a few of –COO− exists in the network of the hydrogel, so the electrostatic repulsive force which Cr2O 2−7 has from –COO− is small, so more Cr2O 2−7 can enter into the network of the hydrogel. On the contrary, when pH is high, there will be more –COO− dissociated from –COOH, the huge electrostatic repulsive force between –COO− and Cr2O 2−7 , and lowers the adsorption capacity of Cr2O 2−7 . The second, with the pH increasing, the number of –NH3+ group would decrease, the electrostatic interaction between –NH +3 group and Cr2O 2−7 ion turned weak.
According to above the experimental result, the following experiments for adsorption of Cu2+ and Cr2O 2−7 ions were carried out at pH 3.5.
Effect of ionic strength
To investigate the effect of ionic strength on the adsorption capacity of hydrogel, at pH 3, 0.1 g hydrogels was added into 100 ml of 3 mg/ml CuSO4 solutions or K2Cr2O7 with a NaCl concentration of 7, 9, 12, 15, 30 wt.%, respectively, and stood 24 h. The ion adsorptive capacity of hydrogels was determined. Results were illustrated in Fig. 3.
To both of Cu2+ and Cr2O 2−7 ions, along with ionic strength increasing, the adsorption capacity of hydrogel decreased. Adsorption capacity of hydrogel to cation and anion strongly rely on the ionic strength. One possible explanation for this phenomenon is an electric-shielding effect. Salt additives would screen the electrostatic interactions of the carboxylic groups of poly(acrylic acid) and make the polymer chains cohere and shrink [18, 20].
Effect of initial ion concentration
In theory, the adsorption capacities of adsorbents for metal ions should increase with the increasing of initial ion concentration, and when the initial ion concentration increased to a certain range, the adsorption capacities would reached maximum, and not change or just vary very few. But our experimental results were contradicted with this presume. In this study, at pH 3, 0.1 g hydrogels was added into 100 ml of CuSO4 solutions, the concentration of Cu2+ ion ranged from 0.4 mg/mL to 12 mg/mL or K2Cr2O7 solutions, the concentration of Cr2O 2−7 ion ranged from 0.2 mg/mL to 0.6 mg/mL with a NaCl concentration of 7 wt.%, respectively.
From Fig. 4, it can be seen that while the initial ion concentration increased, both to Cu2+ and Cr2O 2−7 ions, the adsorption capacity of the hydrogel continuously increased. To cationic Cu2+ ion, the adsorption amount reached 7,000 mg/g in 12 mg/mL Cu2+ solution; however it seems that this point is not the ending. Limited by the solubility of CuSO4, no further adsorption experiment was done. So does to anionic Cr2O 2−7 ion, in which the adsorption amount is 18 mg/g in 0.6 mg/mL. This results may be caused by the structure of hydrogel, which is appropriate to be linked by the three-dimensional network structure and can significantly absorb a large amount of water or ion solution.
Adsorption isotherms
The Freundlich isotherm expression is empirical equation based on adsorption on a heterogeneous surface, describes reversible adsorption, is not restricted to the formation of monolayer, and predicts that the initial ion concentrations on adsorbents would increase so long as there is an increase in the initial ion concentration in solution [5]. The equilibrium isotherm data were characterized by Freundlich equation. The Freundlich equation can be expressed as a simple model as follow:
Where Qe (mg/g) is the equilibrium value for removal of adsorbate per unit weight of adsorbent, Ce (mg/L) is the equilibrium concentration of metal ion in solution, and Kf and n are Freundlich isotherm constants which are related to the adsorption capacity (or the bonding energy) and intensity of the sorbent, respectively [21, 22]. Equilibrium data obtained were fitted to Freundlich isotherms, linear plots of lnQe versus lnCe for the different initial ion concentrations was presented in Fig. 5. The co-efficient of co-relation (R 2) was found to be as high as 0.9863 and 0.9223 for adsorption of Cu2+ and Cr2O 2−7 ions indicating good compliance with Freundlich model. Freundlich equation value of Kf is 145.67 and 8.42 mg/g, the value of n is 0.66 and 0.94 to Cu2+ and Cr2O 2−7 ions respectively. According to Freundlich adsorption theory, the n values between 0.1 and 1 represented favorable adsorption conditions [23]. These results showed that the equilibrium adsorption data of Cu2+ and Cr2O 2−7 ions conformed well to the Freundlich isotherms.
Conclusion
The adsorption properties of silica-PAA amphoteric hybrid hydrogel with DN structure for cation and anion (Cu2+ and Cr2O 27 ) from water solution were investigated. It was found that not only ion density but also external stimuli such as the pH, and ionic strength of solution, play an important role in the adsorption behavior of the hydrogel. Compare with previous report, after introduced silica network, the silica-PAA amphoteric hybrid hydrogel with DN structure can keep high mechanic strength, and also showed high adsorption capacity to cation and anion. The adsorption of the hydrogel followed Freundlich isotherm.
References
Tang L, Liu W, Liu G (2010) High-strength hydrogels with integrated functions of H-bonding and thermoresponsive surface-mediated reverse transfection and cell detachment. Adv Mater 22:2652–2656
Wu J, Ge Q, Mather PT (2010) PEG-POSS multiblock polyurethanes: synthesis, characterization, and hydrogel formation. Macromolecules 43:7637–7649
Shi J, Liu L, Liu X, Sun X, Cao S (2008) Inorganic-organic hybrid alginate beads with LCST near human body temperature for sustained dual–sensitive drug delivery. Polym Adv Technol 19:1467–1473
Sahiner N (2007) Colloidal nanocomposite hydrogel particles. Colloid Polym Sci 285:413–421
Zhu X, Jiang X, Cheng S, Wang K, Mao S, Fan L-J (2010) Preparation of high strength ultrafine polyvinyl chloride fibrous membrane and its adsorption of cationic dye. J Polym Res 17:769–777
Zou H, Wu S, Shen J (2008) Polymer/silica nanocomposites: preparation, characterization, properties, and applications. Chem Rev 108:3893–3957
Yang J, Wang X, Xie X (2012) In situ synthesis of poly(acrylic acid) physical hydrogels from silica nanoparticles. Soft Matter 8:1058–1063
Fei X, Xu S, Feng S, Lin J, Lin J, Shi X, Wang J (2011) Mechanically strengthened double network composite hydrogels with high water content: a preliminary study. J Polym Res 18:1131–1136
Shi X, Xu S, Lin J, Feng S, Wang J (2009) Synthesis of SiO(2)-polyacrylic acid hybrid hydrogel with high mechanical properties and salt tolerance using sodium silicate precursor through sol-gel process. Mater Lett 63:527–529
Johnson JA, Turro NJ, Koberstein JT, Mark JE (2010) Some hydrogels having novel molecular structures. Prog Polym Sci 35:332–337
Karino T, Okumura Y, Zhao CM, Kataoka T, Ito K, Shibayama M (2005) SANS studies on deformation mechanism of slide-ring gel. Macromolecules 38:6161–6167
Seiffert S, Sprakel J (2012) Physical chemistry of supramolecular polymer networks. Chem Soc Rev 41:909–930
Tai Y, Watanabe M, Kaneko K, Tanemura S, Miki T, Murakami J, Tajiri K (2001) Preparation of gold cluster/silica nanocomposite aerogel via spontaneous wet-gel formation. Adv Mater 13:1611–1614
Kawaguchi M, Fukushima T, Hayakawa T, Nakashima N, Inoue Y, Takeda S, Okamura K, Taniguchi K (2006) Preparation of carbon nanotube-alginate nanocomposite gel for tissue engineering. Dent Mater J 25:719–725
Huang T, Xu H, Jiao K, Zhu L, Brown HR, Wang H (2007) A novel hydrogel with high mechanical strength: a macromolecular microsphere composite hydrogel. Adv Mater 19:1622–1626
El-Hag Ali A, Shawky HA, Abd El Rehim HA, Hegazy EA (2003) Synthesis and characterization of PVP/AAc copolymer hydrogel and its applications in the removal of heavy metals from aqueous solution. Eur Polym J 39:2337–2344
Bajpai SK, Johnson S (2005) Superabsorbent hydrogels for removal of divalent toxic ions. Part I: synthesis and swelling characterization. React Funct Polym 62:271–283
Takahashi A, Nagasawa M (1964) Excluded volume of polyelectrolyte in salt solutions. J Am Chem Soc 86:5543–5548
Ören S, Çaykara T, Kantoglu Ö, Güven O (2000) Effect of pH, ionic strength, and temperature on uranyl ion adsorption by poly(N-vinyl 2-pyrrolidone-g-tartaric acid) hydrogels. J Appl Polym Sci 78:2219–2226
Ceylan D, Okay O (2007) Macroporous polyisobutylene gels: a novel tough organogel with superfast responsivity. Macromolecules 40:8742–8749
Dubey A, Shiwani S (2012) Adsorption of lead using a new green material obtained from Portulaca plant. Int J Environ Sci Technol 9:15–20
Bayramoglu G, Arica MY (2011) Preparation of a composite biosorbent using scenedesmus quadricauda biomass and alginate/polyvinyl alcohol for removal of Cu(II) and Cd(II) ions: isotherms, kinetics, and thermodynamic studies. Water Air Soil Pollut 221:391–403
Baskaralingam P, Pulikesi M, Elango D, Ramamurthi V, Sivanesan S (2006) Adsorption of acid dye onto organobentonite. J Hazard Mater 128:138–144
Acknowledgement
This work was supported by the National Natural Science Foundation of China (50763005), the Startup Foundation for Doctors of Xinjiang University, China (BS090119), and National University Student Innovation Program of China (101075505).
Author information
Authors and Affiliations
Corresponding author
Rights and permissions
About this article
Cite this article
Fei, C., Huang, D. & Feng, S. Adsorption behavior of amphoteric double-network hydrogel based on poly(acrylic acid) and silica gel. J Polym Res 19, 9929 (2012). https://doi.org/10.1007/s10965-012-9929-y
Received:
Accepted:
Published:
DOI: https://doi.org/10.1007/s10965-012-9929-y