Abstract
In this paper, we report a facile hydrothermal route to prepare different morphologies of zinc sulfide nanostructures, using a new inorganic precursor, zinc phthalate [Zn(pht)(H2O)]n, and different sulfur sources. This study focuses on the effect of different sulfur sources on the crystal structure and morphology of the products. The structural and morphological studies of the products were performed by using X-ray diffraction, scanning electron microscopy, transmission electron microscopy and selected area electron diffraction. The high temperature (over 1020 °C) hexagonal ZnS nanostructured spheres self-assembled from ZnS nanocrystals have been synthesized at a low temperature of 160 °C by using thioglycolic acid as sulfur source.
Similar content being viewed by others
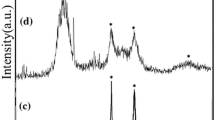
Explore related subjects
Discover the latest articles, news and stories from top researchers in related subjects.Avoid common mistakes on your manuscript.
1 Introduction
In the past few decades, semiconductors as a class of materials defined primarily by their electronic properties, have been of immense interest due to their novel properties such as large surface to volume ratio and three dimensional confinement of electrons [1, 2]. Among II–VI compound semiconductors, zinc sulfide (ZnS) with a direct band gap of 3.66 eV for cubic zinc blende phase and 3.74–3.88 eV for hexagonal wurtzite phase [3], is considered as a versatile semiconductor material with potential applications in photocatalysis [4], photoluminescence and electroluminescence [5–7], infrared optical windows [8], flat panel displays [9], ultraviolet light emitting diodes [10] and so on.
In the past few decades different synthesis routes such as hydrothermal method [11, 12], chemical vapor deposition [13], γ irradiation route [14], sonochemical method [15], microwave irradiation [16], sol–gel technique [17], microemulsion method [18], template method [14], thermal decomposition [19, 20], solid-state reaction [21, 22] were used to synthesize ZnS nanostructures. Among various methods, the hydrothermal route is preferred due to its simplicity, high efficiency, cost effectiveness, attainment of controlled morphology and potential for large scale production [23, 24].
The natural crystal structure of substances is important in physical and chemical properties. ZnS has two phases including thermodynamically metastable hexagonal (wurtzite) phase and more stable phase of cubic (zinc blende) under ambient condition, but above 1020 °C, hexagonal ZnS is a more stable phase [25]. It was reported that nanoscale size [26] or using surface modifying reagent adsorbed on the surface of ZnS nanocrystals [27] may efficiently decrease the phase transformation temperature from cubic to hexagonal.
The shape controlled ZnS nanostructures is another important factor for as-synthesized product to tune their physical properties, since the properties of the nanocrystals are highly dependent on their morphology, size and size distribution. To date, different shape of ZnS nanostructures such as nanoparticles [28], nanowires [29], nanotubes [30], nanosheets [31], nanobelts [32], nanoballs [33], nanotwin belts [34] and well aligned tetrapods [35] have been synthesized. In comparison with mono-morphological structures, the complex 3D structures constructed from nanoscale building blocks show novel properties because of collective features of nanoscale building blocks [36]. For instance, assembly of nanoparticles to monodispersed spheres is crucial for the applications in 2D and 3D arrayed photonic crystals in micro and nanoelectronic devices, optics, electronics and so on [37–49].
There is an immense challenge to develop an environmentally safe and moderate method to produce ZnS nanostructures from a simple precursor. Use of the novel compound such as organometallic and inorganic compound can be useful for preparing nanomaterial to tune shape, size and size distribution. In this paper, a facile hydrothermal approach to fabricate ZnS nanoclusters with different crystal structures and morphologies has been reported. The synthesis features application of [Zn(pht)(H2O)]n and different sulfur sources as novel precursors. The morphology and crystal structure of the products were also investigated by X-ray diffraction (XRD), scanning electron microscopy (SEM), transmission electron microscopy (TEM) and selected area electron diffraction (SAED).
2 Results and Discussion
The XRD patterns and crystallite size of the as-synthesized products obtained from the reaction between Zn[(pht)(H2O)]n complex and different sulfur sources are shown in Fig. 1 and Table 1 respectively. As it is known, in the bulk form and under ambient conditions, the cubic phase of ZnS is more stable than the hexagonal phase, but at temperatures above 1020 °C, the hexagonal phase is more stable [25]. The change in the surface energy of the ZnS nanostructures can decrease the phase transformation temperatures [39]. Also, the surface-modifying reagents influences on the surface energy of prepared ZnS nanocrystals and can efficiently decrease the phase transformation temperature from cubic to hexagonal phase. For example, the hexagonal phase of ZnS nanocrystals was prepared by hydrothermal at 200 °C in the presence H2O/ethanolamine [40]. As shown in Fig. 1a, by using Na2S2O3 as sulfur source, cubic phase (JCPDS = 77-2100) was obtained along with Na2S impurity (JCPDS = 01-1085), while pure cubic ZnS products (JCPDS = 01-0792) were synthesized using thioacetamide as a sulfur source (Fig. 1b). Figure 1c shows the XRD pattern of the as-synthesized sample using thiourea as sulfur source. It clearly shows that two phases of cubic and hexagonal, coexist in this sample (JCPDS = 80-0007 and 80-0020). By using thioglycolic acid as sulfur source, the pure phase of nanostructured hexagonal ZnS was obtained. In the current synthesis system, thioacetamide, thiourea and thioglycolic acid have different effects on the crystal structure of ZnS nanocrystals. Taking into account the chemical structure of these three sulfur sources, one can see that thioacetamide has two donor groups of CH3 and NH2 conjugated to C=S fragment, thiourea has two donor groups of NH2 conjugated to C=S, while thioglycolic acid has a functional group of –SH. The –SH group is more hydrophilic than –CSNH2 and –CSCH3 and can easily bond to zinc ions [40]. So, the reaction of thioglycolic acid with Zn ions is more efficient that may lead to a change of surface energy and formation of the hexagonal wurtzite phase at low temperatures. Comparing thioacetamide and thiourea, one can conclude that thiourea connect to Zn ions easier than thioacetamide. So, in thiourea case, changing the surface energy is mediocre and a mixture of cubic and hexagonal phase of ZnS nanocrystals coexist in the sample, but about thioacetamide sulfur source, only cubic phase of the product was prepared.
Figure 2a shows the SEM image of ZnS microspheres prepared by using Na2S2O3 as sulfur source. The as-prepared microspheres are composed of nanoparticles, so they display rough surface. When thioacetamide or ammonium sulfide were used as sulfur source, due to the high S2− ion release rate, tiny nanoparticles were produced (Fig. 2b, c). In the product prepared from the aqueous solution contained thiourea as sulfur source, elongated ZnS crystals are present along with nanoparticles (Fig. 2d). As shown in the XRD pattern, the sharper (002) diffraction peak in Fig. 1c suggests the preferred growth along the [001] direction, i.e. c-axis, but it is surely required to optimize reaction conditions to control the growth of wurtzite ZnS nanorods. SEM image of the product that was synthesized from the reaction between [Zn(pht)(H2O)]n complex and thioglycolic acid as a sulfur source in hydrothermal medium is shown in Fig. 3a. Before the hydrothermal process, the composite structure of ZnSHCH2COOH is produced. Because of steric hindrance of the precursor and difficulty of thioglycolic acid to link with Zn2+, each completed ZnS cluster can grow, leading to the formation of the complexed ZnS particle. At the same time, some of the organic ligands of SCH2COOH− broke away from the complexed ZnS particles. The formation of the spherical ZnS based nanoparticles is due to the formation of complexed ZnS particles and dissociation of SCH2COOH− proceeded concurrently during the hydrothermal process [41]. SEM image of the products that are synthesized with Zn complex and thiosemicarbazide, cysteine and carbon disulfide are shown in Fig. 3c, d respectively. Use of these sulfur sources led to the formation of nanosized microspherical aggregates with some nanoparticles.
Figure 4a–c shows TEM image of the products prepared from thioglycolic acid sulfur source. It can be seen that the sample is composed of nanoparticles. Selected area electron diffraction of this product shows the hexagonal structure of the products (Fig. 4d). It can be seen that due to the encapsulation with the organic compound of thioglycolic acid, the electron diffraction rings were relatively obscure.
3 Conclusion
In this study, zinc blende and wurtzite ZnS nanocrystals have been successfully synthesized by hydrothermal method via a facile solution phase, in the presence of [Zn(pht)(H2O)]n complex precursor and different sulfur sources without using templates and surfactants. Indeed, it is deduced that steric hindrance of phthalate precursors acts like a protecting agent and there is no need to use capping agents. Using only this novel precursor compound without any surfactants can be useful because it opens a new way to control size and morphology of the products. Also, the sulfur source can affect on crystal structure and shape of the ZnS nanocrystals. ZnS nanostructures with controlled synthesis procedure have a high potential for providing low cost and large scale functional nanomaterials with new properties and applications.
4 Experimental
4.1 Materials and Physical Measurements
All the chemicals in our experiments were of analytical grade and used as-received without further purification. The precursor complexes zinc phthalate, [Zn(pht)(H2O)]n were prepared according to the procedure described previously [38]. A Teflon-lined stainless steel cylindrical closed chamber with 150 ml capacity was used for the synthesis. The water used in this work was Millipore (18.2 MΩ) deionized water. XRD patterns were recorded by a Rigaku D-max C III, filtered Cu Kα radiation (λ = 1.5406 Å). GC-2550TG (Teif Gostar Faraz Company, Iran) were used for all chemical analyses. SEM images were obtained on Philips XL-30 ESEM equipped with an energy dispersive X-ray spectroscopy. TEM images and SAED pattern were obtained on a Philips EM208 transmission electron microscope with an accelerating voltage of 200 kV.
4.2 Synthesis of ZnS Nanostructures
In a typical synthesis procedure [Zn(pht)(H2O)]n and different sulfur sources in a molar ratio of 1:3 were added to 100 ml distilled water. After 40 min stirring, the solution was transferred to the Teflon-lined container and was placed inside a box furnace at a chosen temperature of 160 °C for 12 h and then gradually cooled down to room temperature. The resulting white precipitate was centrifuged and washed with ethanol and distilled water several times for removing unreacted chemicals and dried at 60 °C for 8 h.
References
J. Rita, S. Sasi Florence, Chalcogenide Lett. 7, 269 (2010)
M. Salavati-Niasari, E. Esmaeili, M. Sabet, J. Clust. Sci. 24, 799 (2013)
S.H. Yu, M. Yoshimura, Adv. Mater. 14, 296 (2002)
D. Xiang, Y. Zhu, Z. He, Z. Liu, J. Luo, Mater. Res. Bull. 48, 188 (2013)
P.L. Burn, A.B. Holmes, A. Kraft, D.D.C. Bradley, A.R. Brown, R.H. Friend, R.W. Gymer, Nature 356, 47 (1992)
Z. He, Y. Su, Y. Chen, D. Cai, J. Jiang, L. Chen, Mater. Res. Bull. 40, 1308 (2005)
S.V. Svechnikov, L.V. Zav’yalova, N.N. Roshchina, V.E. Rodionov, V.S. Khomchenko, L.I. Berezhinskii, I.V. Prokopenko, P.M. Litvin, O.S. Litvin, Y.V. Kolomzarov, Y.A. Tsyrkunov, Semiconductors 34, 1128 (2000)
P. Calandra, M. Goffredi, V.T. Liveri, Colloids Surf. A 160, 9 (1999)
M. Bredol, J. Merikhi, J. Mater. Sci. 33, 471 (1998)
D.W. Wang, S.L. Zhao, Z. Xu, C. Kong, W. Gong, Org. Electron. 12, 92 (2011)
M. Salavati-Niasari, M.R. Loghman-Estarki, F. Davar, J. Alloy. Compd. 475, 782 (2009)
M. Salavati-Niasari, F. Davar, M. Mazaheri, J. Alloy. Compd. 470, 502 (2009)
J.Z. Liu, P.X. Yan, G.H. Yue, L.B. Kong, R.F. Zhuo, D.M. Qu, Mater. Lett. 60, 3471 (2006)
D. Zhang, L. Qi, H. Cheng, J. Ma, J. Colloid Interface Sci. 246, 413 (2002)
X.H. Liao, J.J. Zhu, H.Y. Chen, Mater. Sci. Eng. B 85, 85 (2001)
J.J. Zhu, M. Zhu, J. Xu, X. Liao, Mater. Lett. 47, 25 (2001)
N.I. Kovtyukhova, E.V. Buzaneva, C.C. Waraksa, T.E. Mallouk, Mater. Sci. Eng. B 69/70, 411 (2000)
J. Xu, Y. Li, J. Colloid Interface Sci. 262, 275 (2003)
A. Sobhani, M. Salavati-Niasari, M. Sobhani, Mater. Sci. Semicond. Process. 16, 410 (2013)
M. Salavati-Niasari, F. Davar, M.R. Loghman-Estarki, J. Alloy. Compd. 494, 199 (2010)
T.Y. Zhou, X.Q. Xin, Nanotechnology 15, 534 (2004)
L.P. Wang, G.Y. Hong, Mater. Res. Bull. 35, 695 (2000)
Z.H. Ibupoto, K. Khun, X. Liu, M. Willander, Nanomaterials 3, 564 (2013)
M. Salavati-Niasari, F. Davar, H. Seyghalkar, E. Esmaeili, N. Mir, CrystEngComm 13, 2948 (2011)
I. Barin, O. Knacke, O. Kubaschewski, Thermochemical Properties of Inorganic Substances: Supplement (Springer, Berlin, 1997), p. 827
F. Huang, J.F. Banfield, J. Am. Chem. Soc. 127, 4523 (2005)
X.J. Chen, H.F. Xu, N.S. Xu, F.H. Zhao, W.J. Lin, G. Lin, Y.L. Fu, Z. Huang, H.Z. Wang, M.M. Wu, Inorg. Chem. 42, 3100 (2003)
W.S. Chae, J.H. Yoon, H. Yu, D.J. Jang, Y.R. Kim, J. Phys. Chem. B 108, 11509 (2004)
H. Moon, C. Nam, C. Kim, B. Kim, Mater. Res. Bull. 41, 2013 (2006)
Y. Li, X. Li, C. Yang, Y. Li, J. Phys. Chem. B 108, 16002 (2004)
L.W. Yin, Y. Bando, J.H. Zhan, M.S. Li, D. Golberg, Adv. Mater. 17, 1972 (2005)
J. Li, Y. Xu, D. Wu, Y. Sun, Solid State Commun. 130, 619 (2004)
Y. Zhao, J.M. Hong, J.J. Zhu, J. Cryst. Growth 270, 438 (2004)
Y. Li, L. You, R. Duan, P. Shi, G. Qin, Solid State Commun. 129, 233 (2004)
Y.C. Zhu, Y. Bando, D.F. Xue, D. Golberg, J. Am. Chem. Soc. 125, 16196 (2003)
X. Fang, T. Zhai, U.K. Gautam, L. Li, L. Wu, Y. Bando, D. Golberg, Prog. Mater. Sci. 56, 175 (2011)
X. Wu, K. Li, H. Wang, J. Alloy. Compd. 487, 537 (2009)
M. Salavati-Niasari, E. Esmaeili, F. Davar, Comb. Chem. High Throughput Screen. 16, 47 (2013)
S.B. Qadri, E.F. Skelton, D. Hsu, A.D. Dinsmore, J. Yang, H.F. Gray, B.R. Ratna, Phys. Rev. B 60, 9191 (1999)
H. Tong, Y.J. Zhu, L.X. Yang, L. Li, L. Zhang, J. Chang, L.Q. An, S.W. Wang, J. Phys. Chem. C 111, 3893 (2007)
F. Davar, M. Mohammadikish, M. Loghman-Estarki, Z. Hamidi, CrystEngComm 14, 7338 (2012)
M. Salavati-Niasari, D. Ghanbari, M.R. Loghman-Estarki, Polyhedron 35, 149 (2012)
M. Salavati-Niasari, Inorg. Chem. Commun. 7, 963 (2004)
M. Esmaeili-Zare, M. Salavati-Niasari, A. Sobhani, Ultrason. Sonochem. 19, 1079 (2012)
M. Salavati-Niasari, N. Mir, F. Davar, Polyhedron 28, 1111 (2009)
M. Salavati-Niasari, F. Davar, Z. Fereshteh, Chem. Eng. J. 146, 498 (2009)
M. Salavati-Niasari, Chem. Lett. 34, 1444 (2005)
M. Salavati-Niasari, Inorg. Chem. Commun. 8, 174 (2005)
M. Salavati-Niasari, F. Davar, A. Khansari, J. Alloy. Compd. 509, 61 (2001)
Acknowledgments
Authors are grateful to the council of Iran National Science Foundation (91053846) and University of Kashan for supporting this work by Grant No. 159271/678.
Author information
Authors and Affiliations
Corresponding author
Rights and permissions
About this article
Cite this article
Sabet, M., Salavati-Niasari, M. & Esmaeili, E. Synthesis of Zinc Sulfide Nanostructures with Different Sulfur Sources via Mild Hydrothermal Route: Investigation of Crystal Phase and Morphology. J Inorg Organomet Polym 26, 738–743 (2016). https://doi.org/10.1007/s10904-016-0374-y
Received:
Accepted:
Published:
Issue Date:
DOI: https://doi.org/10.1007/s10904-016-0374-y