Abstract
Dental implants are an established therapy for oral rehabilitation. High success rates are achieved in healthy bone, however, these rates decrease in compromised host bone. Coating of dental implants with components of the extracellular matrix is a promising approach to enhance osseointegration in compromised peri-implant bone. Dental titanium implants were coated with an artificial extracellular matrix (aECM) consisting of collagen type I and either one of two regioselectively low sulfated hyaluronan (sHA) derivatives (coll/sHA1Δ6s and coll/sHA1) and compared to commercial pure titanium implants (control). After extraction of the premolar teeth, 36 implants were inserted into the maxilla of 6 miniature pigs (6 implants per maxilla). The healing periods were 4 and 8 weeks, respectively. After animal sacrifice, the samples were evaluated histomorphologically and histomorphometrically. All surface states led to a sufficient implant osseointegration after 4 and 8 weeks. Inflammatory or foreign body reactions could not be observed. After 4 weeks of healing, implants coated with coll/sHA1Δ6s showed the highest bone implant contact (BIC; coll/sHA1Δ6s: 45.4 %; coll/sHA1: 42.2 %; control: 42.3 %). After 8 weeks, a decrease of BIC could be observed for coll/sHA1Δ6s and controls (coll/sHA1Δ6s: 37.3 %; control: 31.7 %). For implants coated with coll/sHA1, the bone implant contact increased (coll/sHA1: 50.8 %). Statistically significant differences could not be observed. Within the limits of the current study, aECM coatings containing low sHA increase peri-implant bone formation around dental implants in maxillary bone compared to controls in the early healing period.
Similar content being viewed by others
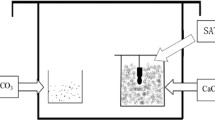
Explore related subjects
Discover the latest articles, news and stories from top researchers in related subjects.Avoid common mistakes on your manuscript.
1 Introduction
Oral rehabilitation with dental implants has become an established therapy in the last decades. Success rates of 97 % over 10 years are being achieved [1]. However, these success rates decrease in compromised host bone depending on the implant position e.g. in reduced bone quality [2]. Due to the demographic changes in industrial countries, an increasing number of patients will be suffering from diseases influencing bone healing. Thus, perspectively a growing number of implants will be inserted in sites of poor bone quality. According to Brånemark [3], the crucial pre-condition for sufficient implant healing is osseointegration. Enhancing osseointegration will be beneficial for the success rates in compromised bone. In recent years, different approaches to positively influence the healing of dental implants have been developed. Variations in implant macro design, micro surface roughness and the surface coating with e.g. nanocrystalline hydroxyapatite are possible means. Another promising approach is the coating of titanium implants with organic components of the extracellular matrix (ECM). The main organic component of bone ECM is collagen type I. Additionally, the ECM in bone contains glycosaminoglycans (GAG) like chondroitin sulfate (CS), dermatan sulfate (DS) and hyaluronan (HA) [4]. Among GAGs, HA is unique considering its linear structure and high molecular mass, the absence of sulfate groups and the fact that it is not bound to a protein [5]. Due to its specific structure and poly-anionic character, HA is capable of binding large amounts of water and ions. Thus, it has hydration and buffering functions [6]. Furthermore, HA influences inflammation and wound healing. In an animal study, the application of HA led to a decreased expression of matrix metalloproteinase 3 (MMP3) and interleukin-1beta (IL-1β) in mild forms of osteoarthritis in rabbits [7]. In addition, HA is known to interact with various proteins. Several HA binding proteins have been described, e.g. proteoglycans (aggrecan, versican) and the cell surface HA receptor CD44 [8].
HA had an enhancing effect on new bone formation in rat femurs [9]. Osseous defects augmented with high molecular weight HA showed a fostered osteogenesis when compared to controls. It was assumed, that growth factors such as TGF-β are retained in the defects by HA, thereby inducing new bone formation [9].
To modulate the properties of HA, sulfate groups can be introduced. Nagahata et al. [10] found an increased activity of alkaline phosphatase (AP) in rat calvarial osteoblasts when culturing them in a medium containing a high concentration of sulfated hyaluronan (sHA). Specific interactions between sHA and recombinant human bone morphogenetic protein 4 (rhBMP-4) as well as transforming growth factor beta 1 (TGF-β1), depending on the number and the sterical position of the sulfate groups have been described [11, 12].
Recent in vitro studies demonstrated an enhancing effect of sHA on the osteogenic differentiation of human mesenchymal stroma cells in two and three dimensional setups [13–16]. Furthermore, an inhibitory effect on osteoclastogenesis was observed by Salbach et al. [17] on murine and primary human osteoclasts. In another in vitro study, an immune-modulating effect on human polarized pro-inflammatory M1 macrophages was demonstrated for sHA. Macrophages grown on collagen I and high sulfated HA showed a reduced inflammatory activity compared to controls [18]. This reduced inflammatory response might have a positive effect on the osseointegration of implants.
The aim of the present study was to evaluate in vivo the influence of artificial extracellular matrix (aECM) coatings containing two different sHA modifications on osseointegration compared to uncoated titanium. We hypothesized that the coating of dental titanium implants with collagen and two different sHA derivatives enhances osseointegration in the early healing phase. Therefore, coated and uncoated dental implants were inserted in the maxilla of six minipigs and examined by histomorphological and histomorphometrical means after 4 and 8 weeks.
2 Materials and methods
2.1 Materials
HA (from Streptococcus, MW = 1.1 × 106 g mol−1) was purchased from Aqua Biochem (Dessau, Germany). Sulfur trioxide/dimethylformamide complex (SO3-DMF, purum, ≥97 %, active SO3 ≥48 %) and sulfur trioxide/pyridine complex (SO3-pyridine, pract.; ≥45 % SO3) were purchased from Fluka Chemie (Buchs, Switzerland). Collagen type I from rat tail tendon was obtained from BD Bioscience (Heidelberg, Germany). The implants being used in this study were provided by Thommen Medical AG (Waldenburg, Switzerland). The screw type titanium implants (commercial pure titanium grade 4) with a gritblasted and thermally acid-etched microrough surface had a length of 15 mm and a diameter of 5 mm.
2.2 Preparation of HA derivatives
The low-sulfated HA (sHA1) was synthesized and characterized as described earlier [11, 19]. In brief, the tetrabutylammonium salt of HA (TeBA-HA) was used as starting material for the sulfation and suspended under argon at room temperature in DMF. SO3-pyridine complex dissolved in DMF was added to a suspension of TeBA-HA in DMF (molar polymer/SO3 ratio: 1:7). The sulfated products were precipitated into acetone and neutralized using ethanolic NaOH solution. The formed sodium salt of the sulfated GAG was washed several times with acetone and purified by dialysis against distilled water followed by lyophilization of the aqueous solutions and drying of the resulting polymers under vacuum. The preparation of the sulfated HA derivative sHA1Δ6s was previously reported by Becher et al. [20]. In a first step the primary hydroxyl group at C6 was protected by benzoylation of TeBA-HA in a mixture of DMF and pyridine with benzoyl chloride. The product was precipitated in water, washed with water and methylene chloride and dried in vacuum. The C6-benzoylated HA was sulfated in dry DMF with SO3-DMF (molar polymer/SO3 ratio 1:15). The reaction mixture was poured into acetone adjusted to pH 10 by addition of ethanolic NaOH solution and the product was isolated after neutralization of the mixture with HCl. After washing with acetone, the sulfated product was dissolved in aqueous K2CO3 solution for deprotection and stirred over night. Purification was performed by dialysis against distilled water, followed by lyophilisation of the solution and drying of the resulting polymer under vacuum.
2.3 Polymer characterization
The degree of sulfation (D.S.) giving the average number of sulfate groups per disaccharide repeating unit of HA was determined by estimation of the sulfur content using an automatic elemental analyzer (CHNS-932, Leco, Mönchengladbach, Germany). NMR spectra were recorded in D2O (99.9 %, Sigma-Aldrich, Schnelldorf, Germany) with a Bruker Advance 400 MHz spectrometer at a temperature of 373 K. D2O at 4.75 ppm was used as a reference line. Molecular weight determination was performed by gel permeation chromatography (GPC) with a double detection system consisting of a Postnova Analytics PN 3000 (15°) laser light scattering (LLS) detector, a Jasco RID-1531 refraction (RI) detector, and Suprema-Gel 10 μm–100 Å,10 μm–1,000 Å and 20 μm–30,000 Å columns. Concerning refraction index detection, the system was calibrated with standard pullulan (PSS, Mainz, Germany). The eluent was phosphate buffered saline (PBS; P3813, Sigma-Aldrich) and the flow rate was 0.5 and 0.8 ml/min, respectively. Absolute values of number-average (Mn) and weight-average (Mw) molecular weights were determined using the laser light scattering (LLS) detection system. Calculation of polydispersity (PD = Mw/Mn) was performed on the basis of Mn and Mw values obtained from RI detection. Analytical data of the used GAG derivatives are summarized in Table 1.
2.4 Coating of titanium implants with aECM
Before coating the implants were cleaned consecutively with Triton x 100, aceton, ethanol and deionised, sterile water three times and over 10 min each in an ultrasound bath. Collagen type I was diluted to 1 mg/ml in sterile, ice cold 10 mM acetic acid and mixed on ice with 0.139–0.151 mg/ml or rather 250 μmol/l disaccharide units of sHA1 or sHA1ΔC6 in sterile, ice cold fibrillogenesis buffer (50 mM sodium dihydrogen phosphate and 11 mM potassium dihydrogen phosphate, pH 7.4). The concentration of GAG was related to the respective molecular weight of the disaccharide units to account for the same amount of possible binding sites. Collagen was allowed to form fibrils at 37 °C for 16–18 h. Afterwards, the fibrillar suspensions were centrifuged at 5,000g for 15 min. The pellets were re-suspended to 2 mg/ml collagen in sterile phosphate buffered saline (PBS) and briefly homogenized with an ultrasound horn. Implants were dip-coated in 96 well plates filled with 250 μl collagen suspension for 5 min of gentle shaking. Next, they were dried for 30 min on air. Dip-coating and drying was repeated two more times. The aECM coating was washed by dipping the implants for 10 s in 250 μl deionised, sterile water followed by 15 min drying on air. Washing was repeated one more time and coatings were allowed to dry until the next day. Coated implants were then subjected to gamma sterilization at ≥25 kGy.
The collagen content of aECM coatings on titanium implants was quantified by Sirius Red staining as described by Bierbaum et al. [21–23]. In brief, the coatings were stained with 1 ml 0.1 % Direct Red (Sigma-Aldrich, Schnelldorf, Germany) in saturated picric acid for 1 h. Afterwards implants were washed three times with 0.01 M hydrochloric acid until the solution was free of residual dye. The staining was solubilized with 1 ml 0.1 M sodium hydroxide and quantified at 540 nm. Calibration curves in 24 well plates containing coatings with the same collagen/GAG ratios as for implants were used for comparison.
The GAG content of aECM coatings was quantified by the dimethylmethylen blue (DMMB) assay as described in Hintze et al. [15, 24]. In brief, the coatings were incubated for 24 h at 60 °C in 0.4 ml 0.1 mg/ml papain (Sigma-Aldrich) solubilized in PBS. Afterwards 40 μl samples were mixed with 250 μl DMMB solution (21 mg/l DMMB (Sigma-Aldrich), 5 ml/l ethanol and 2 mg/l sodium formate, pH 1.5) and quantified at 590 nm. Calibration curves with defined GAG concentrations were used for comparison.
2.5 Stability of aECM coating
To test the stability of the coatings, five randomly selected implants of each state were inserted into artificial bone (Sawbones Europe AB, Malmö, Sweden). An implant cavity was prepared according to the manufacturer’s instructions. The implants were inserted with a torque calibrated device reaching 35 Ncm. Next, the implants were resected and the collagen content was quantified as described.
2.6 Animal model
The experimental protocol was approved by the Commission for Animal Studies of the District Government Dresden, Germany. For the current study, six adult female Berlin miniature pigs (breed: “Mini-Lewe”) with an average weight of 79.0 ± 7.5 kg and an age of 3 years and 9 months were used. The animals were randomly subdivided into healing groups of 4 and 8 weeks, respectively.
2.7 Surgical interventions
The animals were kept in appropriate stables and were fed a soft diet during the study period. Fresh water was available ad libidum. All surgical interventions were carried out in general anaesthesia under surveillance of a veterinarian. The induction of anaesthesia was performed by intramuscular injection of 1 mg/kg body weight Dormicum® (Ratiopharm GmbH Ulm, Germany) and 10 mg/kg body weight ketamine (Riemser Arzneimittel AG, Greifswald, Germany). To reduce salivation 0.05 mg/kg body weight atropine (Eifelfango Chem.-Pharm.-Werke, Bad Neuenahr-Ahrweiler, Germany) were administered. Maintenance of anaesthesia was performed via intravenous injection of the above mentioned mixture. For local anaesthesia Ultracain® D-S forte (Sanofi-aventis Deutschland GmbH, Frankfurt/Main, Germany) was applied. Prophylaxis of wound infection was performed by intramuscular injection of 15 mg/kg body weight Duphamox® LA (Fort Dodge Veterinär GmbH, Würselen, Germany). For postoperative analgesia 3 mg/kg body weight Rimadyl® (Sigma-Aldrich Chemie GmbH, Steinheim, Germany) were administered subcutaneously. First, the premolar teeth were carefully extracted without osteotomy using elevators to avoid fractures of bone walls. After a healing time of 8 weeks, each animal received six implants in the maxilla. In the mandible implants were inserted likewise, which is subject of another study. The implant position was randomized according to the surface state. After a crestal incision, a full mucoperiostal flap was elevated. Sharp edges of the alveolar ridge were flattened and smoothened using round burrs. Under permanent cooling with sterile saline solution the implant sites were prepared using burrs with increasing diameter. The implants were placed torque calibrated with 35 Ncm according to the manufacturer’s protocol. After reposition of the mucoperiostal flap, wound closure was performed using resorbable sutures (Vicryl 3 × 0, Ethicon, Norderstedt, Germany). Postoperatively, wound healing, food intake and animal behaviour were monitored daily.
2.8 Sample preparation
Animal sacrifice was performed in sedation after 4 and 8 weeks by an overdose of T61 (Veterinaria AG, Pfäffikon, Switzerland). After removal of the soft tissue, the maxillae were dissected. The specimens were fixed in 4 % formaldehyde for 48 h. Digital lateral radiographs of the maxillae were performed (Orthophos XG, Sirona Dental Systems GmbH, Bensheim, Germany) for the localization of the implants. Subsequently, the blocks containing the implant and the surrounding bone were dissected. After dehydration in a graded series of ethanol, the specimens were embedded in methylmethacrylate (Technovit 9100 NEU, Heraeus Kulzer, Wehrheim, Germany). Longitudinal sections of 100 μm thickness in oro-vestibular direction were produced using Donath’s sawing and grinding technique [25]. The three central sections of each specimen were chosen for evaluation. After grinding and polishing, the sections were stained with Masson-Goldner trichrome staining.
2.9 Histological evaluation
Subsequently, the specimens were imaged by light microscopy (Olympus BX 61, Olympus Deutschland GmbH, Hamburg, Germany). Using an automatic scanning table (Märzhäuser, Wetzlar, Germany), 15 images per implant were scanned with a 4 × 10 fold magnification and fused to one image. The evaluation of the sections was performed by one masked examiner.
Possible inflammatory reactions and the quality of bone formation were histomorphologically evaluated. The percentage of mineralized bone (bone implant contact, BIC) and non-mineralized osteoid (osteoid implant contact, OIC) along the entire implant surface and in spongious bone areas (BIC spong; OIC spong) was measured. Area fractions of mineralized (bone volume density, BVD) and non-mineralized bone substance (osteoid volume density, OVD) were measured in an area within two implant threads in the middle part of the implant. A tangent line connecting the crests of the mentioned threads was placed defining the area of interest (Fig. 1).
2.10 Statistics
Statistical analysis was performed using IBM SPSS 19.0.0.2 (IBM Corporation, Armonk, NY, USA). Mean values and standard deviations of the parameters were calculated. A linear mixed model was performed. Surface modification and healing time were considered as fixed factors, whereas the inter-animal variability and the implant positions were determined as random factors. The pairwise comparisons of the parameters were tested using the Kolmogorov-Smirnov test. The level of significance was set at α = 0.05.
3 Results
3.1 Structural characteristics of used HA sulfates
In this study regioselectively sulfated HA derivatives have been provided with comparable, relatively low D.S. values of D.S. = 1.0 (sHA1) and D.S. = 1.4 (HA1Δ6s). Despite the comparable D.S values, the two HA sulfates completely differ in their distribution of sulfate groups within the HA disaccharide repeating unit (Fig. 2). Whereas sHA1 is exclusively sulfated at the C6 position of the N-acetyl-D-glucosamine unit, sHA1Δ6s is only sulfated at the C2, and C3 position of the D-glucuronic acid unit but not at the C6 position of the N-acetyl-D-glucosamine sugar. The C4 position of the N-acetyl-d-glycosamine sugar in sHA1Δ6s is also non-sulfated or sulfated to a very low extent. Both HA sulfates are of low molecular weight compared to unmodified HA and polydispersities are in a similar range (Table 1).
3.2 Collagen and GAG quantification of aECM coatings
The collagen content for coll/sHA1 coatings was 81.87 ± 34.99 μg and for coll/sHA1Δ6s 59.66 ± 26.36 μg. The values are the mean value of three triplicates from three independent experiments (n = 9).
The GAG content for coll/sHA1 coatings was 13.44 ± 1.11 μg and for coll/sHA1Δ6s 11.90 ± 0.50 μg. The values are the mean value of triplicates from one experiment (n = 3). In terms of total mass the composition of the aECM coatings was 86 % collagen to 14 % sHA1 versus 83 % collagen to 17 % sHA1Δ6s and therefore quite comparable. The amount of disaccharide units per mg collagen was largely similar as well for both aECM coatings with 294 nmol/mg for coll/sHA1 and 330 nmol/mg for coll/sHA1Δ6s. Furthermore, the collagen content was comparable before and after insertion in the test block revealing that the coating remained stably attached to the implant even during and after implant insertion (Fig. 3).
3.3 Clinical results
All animals showed good general health and completed the study. Two implants (1 × titanium, 1 × sHA1) were lost during the 8 week healing period. All remaining implants showed uneventful healing by the time of euthanasia. No clinical signs of inflammation or foreign body reaction could be observed.
3.4 Histomorphology
After 4 weeks of healing, all surface modifications showed a sufficient degree of osseointegration. No signs of inflammatory reactions could be observed. However, differences between the different surface modifications were distinctive. Implants with titanium surfaces were embedded in woven bone with a pronounced layer of osteoid. Bone formation was initiated in the thread areas. In the implant neck area, crestal bone resorption on the vestibular side was observed. For all uncoated titanium implants, bone formation was predominant in the crestal implant half whereas areas with connective tissue were located in the apical parts (Fig. 4a). Implant surfaces modified with sHA1Δ6s containing aECM were mainly surrounded by a fine meshwork of lamellar bone with less osteoid. The bone was more mature when compared to titanium implants. Bone formation around sHA1Δ6s coated implants was predominantly in the thread areas and was spread along the entire implant length. Crestal bone resorption on the vestibular side could be observed likewise (Fig. 4b). Around implants modified with sHA1 woven bone with a pronounced osteoid layer was obvious. Bone quality was less mature when compared to the other two surfaces. Bone formation was observed in the threads and around the tips of the threads along the entire implant length. Compared to titanium and implants coated with aECM containing sHA1Δ6s the crestal resorption around implants modified with aECM containing sHA1 was more pronounced (Fig. 4c).
After 8 weeks of healing, titanium implants were embedded in a meshwork of lamellar bone which was of similar structure compared to the host bone. The trabecular meshwork was looser when compared to the 4 weeks healing period. Bone formation occurred mainly in the thread areas along the entire implant length. The crestal resorptions were not as pronounced as after 4 weeks (Fig. 4d). The bone around implants covered with sHA1Δ6s containing aECM showed a mature trabecular meshwork with secondary osteons and resorption lacunae indicating remodelling. Osteoid was rarely detectable. No differentiation between newly formed bone and host bone was possible. Compared to the other two surface modifications, more vestibular and bilateral resorption in the crestal area could be observed compared to the other surface modification. Hence, more connective tissue in the crestal area was found (Fig. 4e). Implant surfaces modified with aECM having sHA1 as GAG component were embedded in mature lamellar bone comparable to the host bone. Signs of remodelling were present but less distinctive than at sHA1Δ6s containing surfaces. Bone formation started at the tips of threads, partly extending into the threads. Only few vestibular crestal resorptions were obvious (Fig. 4f).
3.5 Histomorphometry
The means and standard deviations of the BIC, the OIC along the entire implant surface, in spongious bone (BIC spong; OIC spong) and the area fractions of bone and osteoid within the threads (BVD; OVD) are presented in Table 2. The boxplots of the examined parameters are shown in Fig. 5. Over time, an increase of the BIC along the entire implant and in the spongious bone could only be observed for implants coated with aECM containing sHA1. However, the increase was not statistically significant. The decrease of OIC along the implant (Ti: P < 0.05; sHA1Δ6s: P = 0.47; sHA1: P = 0.45) and in the spongious area (Ti: P = 0.003; sHA1Δ6s: P = 0.004; sHA1: P = 0.027) from 4 to 8 weeks was statistically significant for all surface modifications. BVD and OVD within the threads decreased, likewise. After 8 weeks, all examined histomorphometric parameters were higher on coated implants compared to the reference. No statistically significant differences for BIC, BIC spong and BVD could be observed over time or between the surface modifications.
Boxplots presenting data of bone implant contact (BIC) and osteoid implant contact (OIC) along the entire implant in (a/b), in the spongious area (BIC spong; OIC spong) in (c/d) and the area fractions of bone (BVD) and osteoid (OVD) in the thread areas of the implant in (e/f). All data is presented in percent
4 Discussion
In the present study, it was hypothesized that the coating of dental implants with an aECM consisting of collagen type I and either one of two regioselectively different modifications of low sHA derivatives (coll/sHA1∆6s and coll/sHA1) promotes peri-implant bone formation in the early healing phase.
Currently, dental implants are an established treatment option for the rehabilitation of partially and fully edentulous jaws. However, success rates are influenced by the implant position in the jaw. In the posterior maxilla, characterized by a thin cortical layer and large marrow spaces, the risk of implant failure is considerably increased [2, 26, 27]. Enhancing peri-implant bone formation and therefore the osseointegration of dental implants would be desirable to increase success rates in areas of poor bone quality. One approach to enhance osseointegration of dental implants is the coating with organic components of the ECM. Recent animal studies have shown promising results using different organic surface modifications on dental implants [28–30].
In the present study, adult minipigs were chosen as experimental animals due to their similarities to humans regarding development and anatomy of the oro-facial region [31]. Additionally, the bone metabolism is comparable to humans [32]. Therefore, the minipig seems to be a suitable experimental animal for implant research. In order to select a reduced bone quality animal model, the implants were inserted into the maxilla which is characterized by the predomination of spongious bone areas of bone qualities D3 and D4 according to Misch [33]. Primary implant stability and the bone implant contact in these areas are considerably lower compared to the mandible, where bone qualities of D1 and D2 are predominant [33]. A sufficient primary stability implying the absence of micromotion is a crucial pre-condition in the early phase of bone healing to allow osseointegration [3]. In the present study, the primary stability was achieved by using a torque calibrated insertion device reaching an insertion torque of 35 Ncm which is generally seen as sufficient. Two implants were lost during the 8 weeks healing period. This failure rate is comparable to a previous study in the maxilla [30]. Reasons for early implant failure in minipigs can be wound infection caused by poor oral hygiene or masticatory stress. Perioperative infection control was performed by administration of antibiotics. Masticatory stress in animals cannot be prevented and possibly leads to micromotion of the implant which can cause implant failure.
The evaluation of the measurement parameters was performed histomorphologically and histomorphometrically using three undecalcified microsections per implant. Histological means are considered as “gold standard” to evaluate peri-implant bone formation and used in many studies [28, 34, 35]. Histomorphometry allows an exact measurement of BIC and BVD. This is supported by Bernhardt et al. [36] who showed in an experimental study, that the examination of three to four histological sections allows a sufficient description and measurement of the peri-implant bone formation.
The coating of the implants was performed using an established protocol [21]. A crucial issue is the fact that the coating might be partially sheared off during implant insertion. In the experimental setup of this study could be demonstrated that almost no reduction of the coating mass occurred during the insertion procedure, being shown by experiments with artificial bone, which were performed using the same torque. Thus, it can be supposed that the surface coating mainly remains on the implant surface during insertion and will be able to actively interact with the surrounding biological system.
Pure collagen I was used as an implant coating with inconsistent results [28, 30, 37]. Rammelt et al. [37] found an increased BIC around medullary titanium nails coated with collagen I compared to uncoated controls in rat femurs. Schliephake et al. [28] could not support this finding when comparing to acid-etched titanium implants. In another study performed in the maxillary bone of minipigs, no enhancing effect of a coating containing pure collagen I on peri-implant bone formation could be found after 4 weeks compared to uncoated acid-etched titanium implants [30]. One reason might be that acid-etched implant surfaces itself enhance bone implant contact due to their surface’s morphological features [28].
In the present study, the coating of the implants consisted of collagen I and two different regioselectively sulfated derivatives of HA. Its fibrillary structure enables collagen to associate the GAG molecules. Thus, collagen I might act as a carrier for the sulfated HA derivatives. Pure collagen I coating was not included in the study as a control which can be considered as a drawback of the set up. However, in the maxilla of the minipig pure collagen I did not show an enhancing effect regarding bone formation after 4 and 8 weeks [30]. Thus, it was reasonable not to include it in the present study.
The histological evaluation showed no inflammatory reactions for both sHA modifications. No signs of resorption or fibrous integration could be observed. This is in accordance to an in vitro study where an inhibition of the differentiation of monocytes into macrophages in the presence of a collagen/sHA matrix was observed [38]. Additionally, pro-inflammatory macrophages grown on collagen I/sHA showed a reduced inflammatory activity [18]. This decreased inflammation might positively influence the healing of dental implants. These findings were made in vitro and under sterile conditions which are not feasible in animal experiments. But nevertheless, these in vitro data suggest that collagen/sHA matrices are suitable surface modifications.
After 4 weeks of healing, the BIC of coll/sHA1 was almost identical to the titanium reference. Stadlinger et al. observed a BIC for sHA containing aECM even lower than for the titanium Ref. [30]. In the latter study, two different degrees of sulfation (high, D.S. 2.9 and very low, D.S. 0.3) were used. It was concluded, that a very low sulfation degree might result in too weak binding of bone stimulating factors. In the current study, therefore HA sulfation degrees (D.S. 1.0 and 1.4) closer to that of CS (D.S. 0.8–0.9) were chosen, since coll/CS coatings have proofed in several studies to show a positive influence on early bone formation in vivo. There seems to be an effect of the degree of sulfation, although the differences reached no statistical significance. After a healing period of 8 weeks, both modifications showed higher, however not significant, BIC and BVD than the acid-etched titanium reference. These finding are in line with the previous study of Stadlinger et al. [30]. The positive influence of coll/sHA coatings could be explained by the reduced inflammatory response, as mentioned before and further direct effects on cells relevant to healing. In vitro, a stimulating effect of collagen/sHA matrices on the osteogenic differentiation of human mesenchymal stromal cells (hMSC) was observed in two- and three-dimensional setups [13–15]. As far as hMSC are found in the bone marrow niche, the presence of sHA might positively influence their differentiation into osteoblasts. By generating an attractive environment for these cells, adhesion of osteogenic cells on the implant surface and the induction of bone formation could be possible. Another reason might be an inhibitory effect of sHA on osteoclasts. In vitro it was reported that sHA decreased the resorptive activity of osteoclasts [17]. A decreased degradation of bone substance by osteoclasts might lead to increased amounts of bone compared to the reference. Furthermore, unmodified HA itself seems to have a stimulating effect on the osteogenic differentiation of stem cells [39, 40]. In animal studies, unsulfated HA enhanced bone formation in femur and calvarial defects of rats [9, 41]. In a rabbit model, Morra et al. [42] showed a positive influence of a HA coating on bone maturation. It was supposed, that HA stimulates bone formation by accumulating growth factors like TGF-β [9]. One general finding of the present study were the lower values obtained for bone implant contact along the implant and in the spongious bone area compared to Stadlinger et al. [30]. An unexpected finding was that the BIC, except for sHA1, decreased during the study period or persisted on the base level. Previous animal studies have shown an increase of the BIC over time [28, 43]. The BVD in the threads decreased over time, likewise. This decrease was less pronounced for implants coated with coll/sHA1∆6s and coll/sHA1 compared to the reference. This may be due to the absence of implant loading, being a triggering factor on remodelling. Also the animal age should be considered, seeing that most studies analyse osseointegration in adolescent animals with possible higher bone turnover. In the current study, adult minipigs of an age of 3 years and 9 months were used. This might lead to decreased bone turnover and thus to decreased values for the histomorphometrical parameters.
Furthermore, around implants coated with sHA1, the percentage of osteoid was found higher compared to the other surfaces. The deposition of osteoid can be seen as histological sign of osteoblast activity [44]. Thus, sHA1 seems to enhance osteoblast activity in vivo. These findings are in contrast to results of an in vitro study where a decreased activity of rat calvarial osteoblasts in the presence of high-sulfated HA was observed [19]. The degree of sulfation might influence peri-implant bone formation as high-sulfated HA earlier did not show an enhancing effect [30]. An increased degree of sulfation might cause a stronger binding to certain mediators such as BMP-4 or TGF-β1 [11, 12]. It seems possible that the release of these growth stimulating factors is hampered and therefore, the mediator can not address the target cell. Another possible reason might be a hindered interaction with the receptor molecules due to the higher electric charge of the molecule. A lower degree of sulfation might effect an accumulation of stimulating factors with the possibility of release. Thus, osteoblasts and their precursors can be attracted and bone formation can be induced. Therefore, the sHA applied in the present study, were low-sulfated HA derivatives. As anticipated, the percentage of osteoid was lower after the 8 weeks healing period, compared to 4 weeks. This is in line with the observed advanced degree of bone maturation. During the process of bone maturation, osteoid is mineralized resulting in woven bone, which is consecutively being replaced by lamellar bone [45].
In summary, the results found in the current study support findings of in vitro studies regarding the effects of sHA. Except BIC and BIC spong for sHA1 after the 4 weeks healing period, BIC and BVD of the coated implants are increased when compared to the uncoated reference, even though not statistically significant. However, compared with in vivo studies, the results may be somewhat inconclusive. Reasons for this might be the higher complexity of interactions of GAGs and mediators as well as the application in biological systems.
In conclusion, in the present study it could be shown, that two regioselectively different sulfated surface modifications of HA enhance peri-implant bone formation compared to titanium implants after 4 and 8 weeks in the maxilla of minipigs. However, the influence of the degree of sulfation and the position of the sulfated groups is still not clear and has to be elucidated in further studies.
References
Buser D, Janner SFM, Wittneben J, Bragger U, Ramseier CA, Salvi GE. 10-year survival and success rates of 511 titanium implants with a sandblasted and acid-etched surface: a retrospective study in 303 partially edentulous patients. Clin Implant Dent Relat Res. 2012. doi:10.1111/j.1708-8208.2012.00456.x.
Alsaadi G, Quirynen M, Komarek A, van Steenberghe D. Impact of local and systemic factors on the incidence of oral implant failures, up to abutment connection. J Clin Periodontol. 2007. doi:10.1111/j.1600-051X.2007.01077.x.
Branemark PI, Hansson BO, Adell R, Breine U, Lindstrom J, Hallen O, Ohman A. Osseointegrated implants in the treatment of the edentulous jaw. Experience from a 10-year period. Scand J Plast Reconstr Surg Suppl. 1977;16:1–132.
Prince CW, Navia JM. Glycosaminoglycan alterations in rat bone due to growth and fluorosis. J Nutr. 1983;113(8):1576–82.
Taylor KR, Gallo RL. Glycosaminoglycans and their proteoglycans: host-associated molecular patterns for initiation and modulation of inflammation. FASEB J. 2006. doi:10.1096/fj.05-4682rev.
Almond A. Hyaluronan. Cell Mol Life Sci. 2007. doi:10.1007/s00018-007-7032-z.
Takahashi K, Goomer RS, Harwood F, Kubo T, Hirasawa Y, Amiel D. The effects of hyaluronan on matrix metalloproteinase-3 (MMP-3), interleukin-1β(IL-1β), and tissue inhibitor of metalloproteinase-1 (TIMP-1) gene expression during the development of osteoarthritis. Osteoarthr Cartil. 1999. doi:10.1053/joca.1998.0207.
Toole BP. Hyaluronan in morphogenesis. Semin Cell Dev Biol. 2001. doi:10.1006/scdb.2000.0244.
Sasaki T, Watanabe C. Stimulation of osteoinduction in bone wound healing by high-molecular hyaluronic acid. Bone. 1995;16(1):9–15.
Nagahata M, Tsuchiya T, Ishiguro T, Matsuda N, Nakatsuchi Y, Teramoto A, Hachimori A, Abe K. A novel function of N-cadherin and Connexin43: marked enhancement of alkaline phosphatase activity in rat calvarial osteoblast exposed to sulfated hyaluronan. Biochem Biophys Res Commun. 2004. doi:10.1016/j.bbrc.2004.01.098.
Hintze V, Moeller S, Schnabelrauch M, Bierbaum S, Viola M, Worch H, Scharnweber D. Modifications of hyaluronan influence the interaction with human bone morphogenetic protein-4 (hBMP-4). Biomacromolecules. 2009. doi:10.1021/bm9008827.
Hintze V, Miron A, Moeller S, Schnabelrauch M, Wiesmann H, Worch H, Scharnweber D. Sulfated hyaluronan and chondroitin sulfate derivatives interact differently with human transforming growth factor-β1 (TGF-β1). Acta Biomater. 2012. doi:10.1016/j.actbio.2012.03.021.
Hempel U, Moller S, Noack C, Hintze V, Scharnweber D, Schnabelrauch M, Dieter P. Sulfated hyaluronan/collagen I matrices enhance the osteogenic differentiation of human mesenchymal stromal cells in vitro even in the absence of dexamethasone. Acta Biomater. 2012. doi:10.1016/j.actbio.2012.06.039.
Hess R, Jaeschke A, Neubert H, Hintze V, Moeller S, Schnabelrauch M, Wiesmann H, Hart DA, Scharnweber D. Synergistic effect of defined artificial extracellular matrices and pulsed electric fields on osteogenic differentiation of human MSCs. Biomaterials. 2012. doi:10.1016/j.biomaterials.2012.08.056.
Hintze V, Miron A, Moller S, Schnabelrauch M, Heinemann S, Worch H, Scharnweber D. Artificial extracellular matrices of collagen and sulphated hyaluronan enhance the differentiation of human mesenchymal stem cells in the presence of dexamethasone. J Tissue Eng Regen Med. 2012. doi:10.1002/term.1528.
Kliemt S, Lange C, Otto W, Hintze V, Moller S, von Bergen M, Hempel U, Kalkhof S. Sulfated hyaluronan containing collagen matrices enhance cell-matrix-interaction, endocytosis, and osteogenic differentiation of human mesenchymal stromal cells. J Proteome Res. 2013. doi:10.1021/pr300640h.
Salbach J, Kliemt S, Rauner M, Rachner TD, Goettsch C, Kalkhof S, von Bergen M, Moller S, Schnabelrauch M, Hintze V, Scharnweber D, Hofbauer LC. The effect of the degree of sulfation of glycosaminoglycans on osteoclast function and signaling pathways. Biomaterials. 2012. doi:10.1016/j.biomaterials.2012.08.028.
Franz S, Allenstein F, Kajahn J, Forstreuter I, Hintze V, Moller S, Simon JC. Artificial extracellular matrices composed of collagen I and high-sulfated hyaluronan promote phenotypic and functional modulation of human pro-inflammatory M1 macrophages. Acta Biomater. 2013. doi:10.1016/j.actbio.2012.11.016.
Kunze R, Rosler M, Moller S, Schnabelrauch M, Riemer T, Hempel U, Dieter P. Sulfated hyaluronan derivatives reduce the proliferation rate of primary rat calvarial osteoblasts. Glycoconj J. 2010. doi:10.1007/s10719-009-9270-9.
Becher J, Möller S, Weiss D, Schiller J, Schnabelrauch M. Synthesis of new regioselectively sulfated hyaluronans for biomedical application. Macromol Symp. 2010;269:446–52.
Bierbaum S, Douglas T, Hanke T, Scharnweber D, Tippelt S, Monsees TK, Funk RHW, Worch H. Collageneous matrix coatings on titanium implants modified with decorin and chondroitin sulfate: characterization and influence on osteoblastic cells. J Biomed Mater Res A. 2006. doi:10.1002/jbm.a.30572.
Junqueira LC, Bignolas G, Mourao PA, Bonetti SS. Quantitation of collagen-proteoglycan interaction in tissue sections. Connect Tissue Res. 1980;7(2):91–6.
Walsh BJ, Thornton SC, Penny R, Breit SN. Microplate reader-based quantitation of collagens. Anal Biochem. 1992;203(2):187–90.
Farndale RW, Buttle DJ, Barrett AJ. Improved quantitation and discrimination of sulphated glycosaminoglycans by use of dimethylmethylene blue. Biochim Biophys Acta. 1986;883(2):173–7.
Donath K, Breuner G. A method for the study of undecalcified bones and teeth with attached soft tissues. The Sage-Schliff (sawing and grinding) technique. J Oral Pathol. 1982;11(4):318–26.
Friberg B, Jemt T, Lekholm U. Early failures in 4,641 consecutively placed Brånemark dental implants: a study from stage 1 surgery to the connection of completed prostheses. Int J Oral Maxillofac Implants. 1991;6(2):142–6.
Jaffin RA, Berman CL. The excessive loss of Branemark fixtures in type IV bone: a 5-year analysis. J Periodontol. 1991. doi:10.1902/jop.1991.62.1.2.
Schliephake H, Aref A, Scharnweber D, Bierbaum S, Sewing A. Effect of modifications of dual acid-etched implant surfaces on peri-implant bone formation. Part I: organic coatings. Clin Oral Implants Res. 2009. doi:10.1111/j.1600-0501.2008.01603.x.
Stadlinger B, Pilling E, Huhle M, Mai R, Bierbaum S, Bernhardt R, Scharnweber D, Kuhlisch E, Hempel U, Eckelt U. Influence of extracellular matrix coatings on implant stability and osseointegration: an animal study. J Biomed Mater Res Part B Appl Biomater. 2007. doi:10.1002/jbm.b.30787.
Stadlinger B, Hintze V, Bierbaum S, Moller S, Schulz MC, Mai R, Kuhlisch E, Heinemann S, Scharnweber D, Schnabelrauch M, Eckelt U. Biological functionalization of dental implants with collagen and glycosaminoglycans-a comparative study. J Biomed Mater Res A. 2011. doi:10.1002/jbm.b.31953.
Wang S, Liu Y, Fang D, Shi S. The miniature pig: a useful large animal model for dental and orofacial research. Oral Dis. 2007. doi:10.1111/j.1601-0825.2006.01337.x.
Weaver ME, Sorenson FM, Jump EB. The miniature pig as an experimental animal in dental research. Arch Oral Biol. 1962;7:17–23.
Misch CE. Density of bone: effect on treatment plans, surgical approach, healing, and progressive boen loading. Int J Oral Implantol. 1990;6(2):23–31.
Germanier Y, Tosatti S, Broggini N, Textor M, Buser D. Enhanced bone apposition around biofunctionalized sandblasted and acid-etched titanium implant surfaces. A histomorphometric study in miniature pigs. Clin Oral Implants Res. 2006. doi:10.1111/j.1600-0501.2005.01222.x.
Hermann JS, Jones AA, Bakaeen LG, Buser D, Schoolfield JD, Cochran DL. Influence of a machined collar on crestal bone changes around titanium implants: a histometric study in the canine mandible. J Periodontol. 2011. doi:10.1902/jop.2011.090728.
Bernhardt R, Kuhlisch E, Schulz MC, Eckelt U, Stadlinger B. Comparison of bone-implant contact and bone-implant volume between 2D-histological sections and 3D-SRmicroCT slices. Eur Cell Mater. 2012;23:237–47 discussion 247–8.
Rammelt S, Illert T, Bierbaum S, Scharnweber D, Zwipp H, Schneiders W. Coating of titanium implants with collagen. RGD peptide and chondroitin sulfate. Biomaterials. 2006. doi:10.1016/j.biomaterials.2006.06.034.
Kajahn J, Franz S, Rueckert E, Forstreuter I, Hintze V, Moeller S, Simon JC. Artificial extracellular matrices composed of collagen I and high sulfated hyaluronan modulate monocyte to macrophage differentiation under conditions of sterile inflammation. Biomatter. 2012. doi:10.4161/biom.22855.
Astachov L, Vago R, Aviv M, Nevo Z. Hyaluronan and mesenchymal stem cells: from germ layer to cartilage and bone. Front Biosci. 2011;16:261–76.
Bastow ER, Byers S, Golub SB, Clarkin CE, Pitsillides AA, Fosang AJ. Hyaluronan synthesis and degradation in cartilage and bone. Cell Mol Life Sci. 2008. doi:10.1007/s00018-007-7360-z.
Itoh S, Matubara M, Kawauchi T, Nakamura H, Yukitake S, Ichinose S, Shinomiya K. Enhancement of bone ingrowth in a titanium fiber mesh implant by rhBMP-2 and hyaluronic acid. J Mater Sci Mater Med. 2001;12(7):575–81.
Morra M, Cassinelli C, Cascardo G, Fini M, Giavaresi G, Giardino R. Covalently-linked hyaluronan promotes bone formation around Ti implants in a rabbit model. J Orthop Res. 2009. doi:10.1002/jor.20797.
Stadlinger B, Bierbaum S, Grimmer S, Schulz MC, Kuhlisch E, Scharnweber D, Eckelt U, Mai R. Increased bone formation around coated implants. J Clin Periodontol. 2009. doi:10.1111/j.1600-051X.2009.01435.x.
Rutten S, Nolte PA, Korstjens CM, van Duin MA, Klein-Nulend J. Low-intensity pulsed ultrasound increases bone volume, osteoid thickness and mineral apposition rate in the area of fracture healing in patients with a delayed union of the osteotomized fibula. Bone. 2008. doi:10.1016/j.bone.2008.04.010.
Terheyden H, Lang NP, Bierbaum S, Stadlinger B. Osseointegration–communication of cells. Clin Oral Implants Res. 2012. doi:10.1111/j.1600-0501.2011.02327.x.
Acknowledgments
The study was supported by the Transregio 67 grant of the German Research Foundation DFG (A2, A3, B6). The implants were donated by Thommen Medical AG, Waldenburg, Switzerland. The authors are grateful to Dr. Roland Jung and Dr. Kathrin Spekl for their support during the animal experiments. Furthermore, the authors wish to thank Diana Jünger, Heike Zimmermann and Sarah Beckmann for their excellent technical assistance.
Author information
Authors and Affiliations
Corresponding author
Rights and permissions
About this article
Cite this article
Schulz, M.C., Korn, P., Stadlinger, B. et al. Coating with artificial matrices from collagen and sulfated hyaluronan influences the osseointegration of dental implants. J Mater Sci: Mater Med 25, 247–258 (2014). https://doi.org/10.1007/s10856-013-5066-3
Received:
Accepted:
Published:
Issue Date:
DOI: https://doi.org/10.1007/s10856-013-5066-3