Abstract
Ferroelectric–ferrite composites of BaTiO3–CoFe2O4 (BT–CFO) is synthesized via solid state reaction method. Powder XRD confirms the phase purity as well as composite formation with tetragonal phase of the BaTiO3. The FTIR and SEM–EDS studies also confirm the formation of BT–CFO composites. The P–E loop measurement confirmed the ferroelectric nature of the sample. The maximum energy storage density and efficiency achieved for BT–5CFO (5% CoFe2O4) composite was 8.33 mJ/cm3 and an efficiency of 59.7% respectively. The coupling between the ferroelectric and ferromagnetic phases was observed in the variation of P–E loop with magnetic field. A decrease in the maximum polarization was found with increase magnetic field. The P versus M analysis confirmed the highest magneto-electric (ME) coefficient of 13.33 mV/cm/Oe for BT–5CFO composite. These results are related to the volumetric and piezoelectric strain, suppression of ferroelectric ordering reveal the magnetoelectric nature in BaTiO3–CoFe2O4 composites.
Similar content being viewed by others
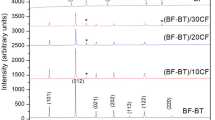
Explore related subjects
Discover the latest articles, news and stories from top researchers in related subjects.Avoid common mistakes on your manuscript.
1 Introduction
An enormous effort has been focused on the study of multiferroic materials with large magnetoelectric (ME) effect in the field of physics and material science for building new types of multistate memory devices [1]. The weak coupling in single phase multiferroics owing due to atomic diffusion and undesirable chemical reactions [2] and their scarcity has motivated the research of composite materials [3]. ME composites with large magnitudes of the ME voltage coefficient have turned out to be better alternatives. These composites exploit the product property of piezomagnetic and piezoelectric phases or individual magnetostrictive and piezoelectric phases of the materials.
Usually a magnetoelectric composite consists of ferroelectric and ferromagnet for piezoelectricity and magnetostriction to exhibit multiferroism. The coupling in these magnetoelectrics arises due to the indirect mechanical strain transfer at the interface of two phases. The composites of BaTiO3–CoFe2O4 has exciting magnetoelectric properties due to the contribution of their individual phases. Perovskite BaTiO3 (BT) is a good functional ceramic with interesting ferroelectric and optical properties has applications as multilayer ceramic capacitors (MLCC), transducers, actuators, energy storage ferroelectric random access memories [4]. CoFe2O4 (CFO), a spinel ferrite, is well known for its remarkable electrical, optical, and magnetic properties, especially in nanometer scale [5]. The earlier work on BT–CFO composites have contributed on the magnetoelectric properties to a certain extent [6, 7]. Though the magnetoelectric coupling observed in these materials was very low due to the undesirable phases of CFO and microstructural distortion in the composites. However, the studies of addition of CFO in low concentration to BT is not completely explored to observe the origin of coupling in BT–CFO composites. The effect of smaller concentration of CFO in BT on the structural, ferroelectric P–E and magneto-electric properties has been studied both qualitatively and quantitatively.
2 Experimental procedures
The solid state reaction method was employed to prepare BaTiO3 and CoFe2O4 bulk ceramics by calcining them at 1000 °C and 900 °C respectively. The powders of BaTiO3 (99% and 95%) of different stoichiometry were mixed with CoFe2O4 (1% and 5%) powders respectively. The samples have been named in composite as BT (pure BaTiO3), BT–1CFO (1% CoFe2O4), and BT–5CFO (5% CoFe2O4). All the mixed composites were then pelletized and sintered at 750 °C for 4 h and BT was sintered at 1100 °C for 4 h. The synthesis conditions have been tabulated in Table 1. Powder X-ray diffraction (XRD) patterns were recorded using an Rikagu X-ray Diffractometer employing Cu Kα radiations. The morphological studies have been done using Scanning electron microscopy (SEM) with EDS analysis. Polarization versus electric field (PE-loop) measurements were done with the help of ‘Marine India’ instrument at different frequency and magnetic field upto electric field of 25 kV/cm. The FTIR studies were made using Bruker FTIR setup.
3 Result and discussion
The phase purity and content for different compositions are shown in Fig. 1. The diffraction patterns were matched and indexed JCPDS file (5-0626) with for the BT [6]. The tetragonality of BT is confirmed from the splitting of diffraction peaks at 2θ diffraction angles: 31°, 45°, 56°, 66° and 75° with miller indices (101), (002), (112), (202) and (103) for all the composites. The intensity of the BT peaks didn’t decrease significantly on increasing the concentration of cobalt ferrite. However small peaks due to cubic spinel cobalt ferrite matched with JCPDS file (22-1086) are observed in BT–5CFO composite (denoted by #). This confirms the co-existence of ferroelectric and ferromagnetic phases.
The presence of CFO and formation of composite of CFO–BT has been verified by the scanning electron microscopy (SEM) and energy-dispersive X-ray spectroscopy (EDX) and have been applied on pure BT and BT–5CFO. Figure 2a show the SEM images of BT and BT–5CFO respectively. The two different colors viz. black corresponds to CFO and white corresponds to BT of SEM images confirms the presence of two different domains of BT and CFO respectively in the BT–5CFO composite. The EDX analysis confirms the presence of CFO in to BT–5CFO sample due clear percentage amount of CFO seen in Fig. 2b and the strength of the peaks enhanced after the CFO addition in to BT. High strength of the peaks of BT–5CFO comparative to pure BT is due to the magnetic nature of the CFO and also further confirms the composite formation [8,9,10,11].
The FT-IR spectrum of the BT and BT–CFO composites is presented in Fig. 3. The characteristic absorption band at 3486 cm−1 is assigned to the O–H stretching vibration in water and confirms that the existence of the water in the BT and BT–CFO composites. The presence of the band located at 542 cm−1 is specific to the vibration mode of the Ti–O bond in the TiO6 octahedra [12]. In cobalt ferrite ceramic, the FTIR spectrum has peaks near 600 and 450 cm−1 represent the characteristic peaks of Co2O3 [13]. The bands at these wavenumbers represent tetrahedral and octahedral clusters and also confirm the presence of Fe–O stretching vibration in cobalt ferrite. The shifting of peak at 690 cm−1 and sharpening of peak at 458 cm−1 in Fig. 3 is observed. The anomaly at bands near 600 and 400 cm−1 confirm the formation of BT–CFO composite.
Polarization versus electric field (P–E) hysteresis loops measured at fixed frequency of 50 Hz for BT, BT–1CFO and BT–5CFO composites are plotted in Fig. 4. These samples show extensive ferroelectric nature at an applied field of 25 kV/cm. There is an observed decrease in maximum polarization of the samples with increase in Cobalt ferrite content indicating the suppression of ferroelectric ordering [14]. The P–E loops did not display any saturation which could possibly be due to low value of electric field.
The P–E loops have been exploited for estimation of energy storage density and discharge efficiency of BT–5CFO sample (Fig. 5). The energy storage density (WU) and efficiency (η) of a ferroelectric material are calculated as [15, 16]
where E is applied electric field, P is polarization respectively, WU is useful stored energy density and WL is energy loss density. The blue region of Fig. 5 represents the loss and the yellow region represents the recoverable useful energy.
The unloaded sample of BT–5CFO displayed the energy density of 8.33 mJ/cm3 and an efficiency of 59.7%. The efficiency can be increased by studying the sample under mechanical stress and which is currently under investigation. The variation in P–E loop due to the field-induced structural transformation is an indicative of volumetric and piezoelectric strains in the BaTiO3 phase.
In order to establish the magnetoelectric effect in composites at room temperature, proving the ferroelectricity of the piezoelectric phase of barium titanate is essential. The hysteresis P–E loop of BT–5CFO composite exhibits a substantial variation with external magnetic field upto 0.4 T as shown in Fig. 6. The remnant polarization is found to decrease and the coercive field do not change with increase in magnetic field. This display the tilting of P–E loops confirming the evidence of magneto-electricity due to increase in the content of CoFe2O4 in BaTiO3 [17, 18]. There was no variation in the P–E loop with magnetic field for BT–1CFO. This could be possibly due to less ferrite grains concentration in the composite which are incapable of generating magnetostriction in BaTiO3.
The magneto-electric coupling quantified by ME coefficient is characterized by the appearance of an electric polarization on applying magnetic field (α = δE/δH). Figure 7 shows the ME induced polarization as a function of the applied external magnetic field (dc). The slope of the curves is used for estimation of the direct magnetoelectric coefficient. Considering only the linear effects, the polarization can be expressed as
where Ps denotes the spontaneous polarization at constant field.
The values obtained from straight line fitting of P–M graph are Ps = 0.78366 and slope dP/dH = − 0.11801 µC/cm2/Oe. It can be seen that the coefficient is negative and low. This decrease in induced polarization in biphasic composites could be explained by the effect of demagnetization arising from local strain. As the amount of magnetic phase is increased in BT, the polar domains of BT get surrounded by the magnetic CFO phase and hinder the domain wall rotation. On the application of magnetic field, a local magnetic field is generated around the BT domains resulting in an effective strain mediated magneto-electric coupling [19]. The M–E coupling coefficient obtained for BT–5CFO composite is found to be 13.33 mV/cm/Oe which is relatively higher than reported values of 10 mV/cm/Oe and 12 mV/cm/Oe for BaTiO3–20% CoFe2O4 and BaTiO3–30% CoFe2O4 respectively [7].
4 Conclusion
In conclusion, we have prepared pure phase BT–CFO composites with tetragonal phase via solid state reaction route and is confirmed from XRD, SEM–EDS and FTIR studies. P versus E measurement confirmed the ferroelectric nature, high energy storage density and efficiency for BT–5CFO (5% CoFe2O4). The P versus E in the presence magnetic field and P versus M measurements confirmed the magneto-electric coupling in the composite both qualitatively and quantitatively which is related to the volumetric and piezoelectric strain, suppression of ferroelectric ordering. These features have direct influence on the application of BT–CFO composites in energy storage and multistate memory devices.
References
X. Lu, Y. Kim, et al., Nano Lett. 11, 3202–3206 (2011)
C.W. Nan, M.I. Bichurin et al., J. Appl. Phys. 103, 031101 (2008)
N. Hur, S. Park et al., Nature 429, 392–395 (2005)
F. Li, M. Zeng et al., J. Mater. Sci. Mater. Electron. 27, 2836–2840 (2017)
S. Jabez, S. Mahalakshmi, S. Nithiyanantham, J. Mater. Sci. Mater. Electron. 28, 5504–5511 (2017)
L.V. Leonel, A. Righi et al., Ceram. Int. 37, 1259–1264 (2011)
C.A.F. Vaz, J. Hoffman et al., Adv. Mater. 22, 2900–2918 (2010)
M. Etier, V. V. Shvartsman et al., J. Am. Ceram. Soc. 99, 3623–3631 (2016)
M. Etier, C.S. Antoniak et al., Acta Mater. 90, 1–9 (2015)
N. Adhlakha, K. L. Yadav, R. Singh, Smart Mater. Struct. 23, 105024-1-16 (2014)
M. Naveed-Ul-Haq, V. V. Shvartsman et al., Sci. Rep. 6, 32164-1-10 (2016)
D. Sun, X. Jin et al., Ferroelectrics 355, 145–148 (2007)
G. Allaedini, S.M. Tasirin, P. Aminayi, Int. Nano Lett. 5, 183–186 (2015)
M. Chandrasekhar, P. Kumar, Physica B 497, 59–66 (2016)
M. Chandrasekhar, P. Kumar, Ceram. Int. 41, 5574–5580 (2015)
V.S. Puli, D.K. Pradhan et al., J. Alloys Compd. 584, 369–373 (2014)
M. Kumar, S. Shankar et al., J. Alloys Compd. 577, 222–227 (2013)
M. Kumar, S. Shankar et al., J. Mater. Sci. Mater. Electron. 26, 1427–1434 (2015)
D. Zhang, J. Cheng et al., J. Alloys Compd. 740, 1067–1076 (2018)
Acknowledgements
Authors are thankful to University of Delhi, New Delhi for funding the Star Innovation Project (ARSD-SIP-01). The authors would like to thank Centre for Nanoscience, Jamia Millia Islamia University, New Delhi, National Physical Laboratory (NPL) New Delhi, and NSIT, New Delhi India for their characterization facilities.
Author information
Authors and Affiliations
Corresponding author
Rights and permissions
About this article
Cite this article
Shankar, S., Kumar, M., Tuli, V. et al. Energy storage and magnetoelectric coupling in ferroelectric–ferrite composites. J Mater Sci: Mater Electron 29, 18352–18357 (2018). https://doi.org/10.1007/s10854-018-9949-4
Received:
Accepted:
Published:
Issue Date:
DOI: https://doi.org/10.1007/s10854-018-9949-4