Abstract
In the present investigation, we are reporting the successive ferroelectric relaxor behavior of (Ba,Sr)TiO3 borosilicate glass ceramics. The ferroelectric relaxor nature of the present glass ceramics is attributed to structural phase transition from the rhombohedral to orthorhombic to tetragonal to cubic. A very high value of dielectric constant was found to be 89,684 at 100 Hz in glass ceramic sample 64[(Ba0.7Sr0.3)TiO3]–30[2SiO2–B2O3]–5[K2O]–1[La2O3], BST5K1L0.3S831 and such large value may be due to space charge polarization in glassy matrix. The various contribution in polarization processes are confirmed by impedance spectroscopic analysis.
Similar content being viewed by others
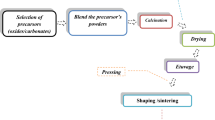
Avoid common mistakes on your manuscript.
1 Introduction
Many efforts have been made from few decades for the development of high dielectric constant ferroelectric glass ceramics. Generally, ferroelectric glass ceramics are used in high energy storage devices such as barrier layer capacitors. Barium titanate is the first ferroelectric glass ceramics, whose crystal structure varies with temperature from rhombohedral to orthorhombic to tetragonal to cubic to hexagonal [1–3]. The replacement of Ba2+ on A site by Sr2+ enhances the ferroelectric properties of solid solution BaTiO3. The addition of SrO in BaTiO3 decreases the Curie temperature of BaxSr1−xTiO3 (BST) ceramic. This property enables the ferroelectric–paraelectric transition temperature to be tailored by varying the Sr content for specific applications such as tunable phase shifters, resonators, filters, wave guides [4]. The SrO in place of BaO in such glass–ceramics increases the its conductivity and lead to the increase in the ionic conductivity with increasing frequency. Due to conductivity difference in various phases of glass ceramics increases the dielectric constant [5]. The glass former B2O3–SiO2 in BST promote the sintering at low temperature by the formation of liquid phase with a low melting point and dielectric constant is increased largely [6]. Some additives like La2O3 have also improves such properties as small amount of La3+ ions, which exclusively replace Ba sites in the perovskite structure of BaTiO3, leads to the formation of n-type BaTiO3 semiconductor [7].
The relaxor ferroelectrics are classified into classical relaxors in which only short range ordering is present and semi-classical relaxor ferroelectrics in which both short and long range orderings are present. The relaxor properties in semi-classical relaxor ferroelectrics can be arises due to compositional inhomogeneities, artificially induced strain, growth conditions [8, 9]. The glass ceramics consist of grains, grain boundaries, major phases, secondary phases, pores and electrode-ceramics surfaces. The conductivity of glass ceramics above constituent in glassy matrix is different and due to this conductivity difference, a space charge polarization take place. Hence, the value of high dielectric constant is attributed in the glass ceramics. These contributions of polarization in dielectrics were analyzed by impedance spectroscopy [10].
2 Materials and methods
(Ba,Sr)TiO3 borosilicate glasses were prepared by conventional melt quench method by high purity ingredients BaCO3, SrCO3, TiO2, SiO2, H3BO3, K2CO3 and La2O3 in glass system [(Ba1−xSrx)TiO3]–[2SiO2–B2O3]–[K2O]–[La2O3] and they are converted into glass ceramics by regulated heat treatment process [11–13]. Investigated glass ceramics are characterized for X-ray powder diffraction using Rigaku powder diffractometer along with 18 kW rotating anode, Cu-target, operating in the Bragg–Brentano geometry and fitted with a low temperature attachment and a curved crystal graphite monochromator in the diffracted beam. The dielectric/impedance spectroscopic measurements were carried out by Wayne Kerr 6500 P (high frequency LCR Meter, Frequency: 20 Hz–5 MHz) in temperature range of −80 to 150 °C of both side electrode (Ba,Sr)TiO3 glass ceramics in frequency range of 100 Hz–100 kHz.
3 Results and discussion
Figure 1a–c depicts the XRD patterns of glass ceramic BST5K1L0.3S831 (BST denotes 64[(Ba0.7Sr0.3)TiO3]–30[2SiO2–B2O3], 5K refers the 5 % of K2O, 1L means 1 mol% of La2O3 and numeric digit, 0.3 is fractional content of Sr whereas S indicates crystallization time of 6 h and 850 means the crystallization temperature) at temperatures 0, 50 and 150 °C respectively. These patterns were indexed by comparing d-values with standard JCPDS cards Nos. 13-0522, 44-0093, 89-8211 and 84-0923. All XRD patterns show the secondary phase formation of Ba2TiSi2O8. BST borosilicate glasses were crystallized into major crystalline phase of Ba1.91Sr0.09TiO4 at 0 °C which exist in orthorhombic crystal structure and a larger amount of secondary phase was also observed in glassy matrix at this temperature. When XRD data was recorded at temperature 50 °C, then crystallographic structure changes from orthorhombic to tetragonal whereas further crystallographic change introduces in BST from tetragonal to cubic at 150 °C. The amount of secondary phase was continuously decreases with increase the temperature of the ceramics [14].
Rhombohedral (R) to orthorhombic (O), orthorhombic to tetragonal (T) and tetragonal to cubic (C) phase transitions were clearly observed in Fig. 2a. These phase transitions are diffuse in nature and described by plot ln(1/ε − 1/εm) versus ln(T − Tm) as shown in inset Fig. 2a, where, Tm is the temperature corresponding to highest value of dielectric constant. The values of diffuseness constant, γ at 100 Hz was found to be 1·63 which confirms diffuse phase transition and indicating high disorderness in the material [14, 15]. The substitution of Sr in BaTiO3 decreases the value of γ and showing to decrease of diffusivity of BST glass ceramics [16]. The plot of log(τ) versus 1,000/T is shown in inset Fig. 2b–d and these plots indicate the linear fit of Arrhenius type (τ = τ 0 exp (E a /k B T) behavior. Here, Ea represents the activation energy for thermally activated jumps of polar clusters, kB and τo are the Boltzmann constant and relaxation time respectively [17, 18]. The values of Ea and τo are 2.09 × 10−3 eV, 4.18 × 10−37 s 2.56 × 10−3 eV, 3.23 × 10−38 s and 5.03 × 10−3 eV, 1.56 × 10−65 s R–O, O–T and T–C phase transitions respectively [14]. The ferroelectric phase transitions were attributed in glassy matrix in three regions of temperature [19]. This may be due to distortion in axial ratio of crystal structure with rise in temperature. With increasing the frequency, temperature corresponding to peak value of dielectric constant in each ferroelectric region shifted to high temperature as shown in Fig. 2b–d. A high value of dielectric constant was attributed to the conductivity difference between various crystalline phases, grains and grain boundary [20].
Figure 3a–f show the complex impedance and complex modulus plots at temperatures 0, 100 and 150 °C of glass ceramics BST5K1L0.3S831. The glass ceramic typically consists of mainly three different electroactive regions as the glass matrix, crystalline phase and crystal–glass interface and these contributions are analyzed by complex impedance and complex modulus analysis. In low temperature regime about 0 °C, only single semicircular arc is seen in complex impedance plane which indicated parallel RC element with larger resistance while in complex modulus plane, there exists two semicircular arcs, indicating small parallel capacitance in RC network. The contributions in relaxation processes were also confirmed almost two semicircular arcs in complex modulus, M// versus M/, plots. These arcs are attributed to the contributions of crystal–glass interfaces and the BST crystalline phases [20] and represented by the series combination of two parallel RC networks as shown in inset Fig. 3a. The resistance of constant was zero because complex impedance plots starts from origin. The complex impedance plot shows two semicircular plots while complex modulus plot shows three semicircular plots at 100 °C. This confirms that the resistance due to glass contribution is very high and its capacitance contribution is low in RC network. All three contributions arises at temperature about 100 °C and the dielectric relaxation occurring at the crystal–glass interfaces is strongly changed, whereas the dielectric relaxation occurring in the crystalline phase is not as strongly influenced. With further rise in temperature up to 150 °C, contribution of crystalline phase as well as grain and grain boundary was enhanced as seen in Fig. 3e, f. The presence of ferroelectric domains and domain walls in glassy matrix BST borosilicate glass ceramics will require a complex equivalent circuit which is series combination of three parallel RC elements [21]. The sharply decrease in Z// with frequency in the low frequency region indicate large contribution of space charge polarization at highly resistive interface.
These glass ceramic samples show the ferroelectric behavior due to addition of La2O3. The solid solution crystallites of BT/BST become semiconducting due to diffusion of La ions in the solution. Strontium titanate leads to the formation of electronic defects/vacancies of titanium cation with addition of La2O3 and which depends on the doping level and processing condition as per the following equations, where all symbols are in Kröger–Vink notations [22].
The doping of La2O3 in small concentration in perovskite ceramics induces n-type semiconductivity by electronic compensation and given by Eq. (3) but higher concentration of La3+ creates titanium vacancies given by Eq. (4).
4 Conclusions
The crystal structure of (Ba,Sr)TiO3 borosilicate glass ceramics depends upon temperature and it associates the ferroelectric relaxor nature. The existence of successive relaxor ferroelectrics was confirmed by dielectric analysis in various regions of temperatures. The contribution of glass, crystalline phases, grains and grain boundary was verified by three semicircular arcs in complex impedance and complex modulus plots. A very high value of dielectric constant for glass ceramic BST5K1L0.3S831 was attributed to conductivity difference between insulating glass, semiconducting crystalline phases, conducting grains and insulating grain boundary.
References
F. Wan, J.G. Han, Z.Y. Zhu, Phys. Lett. A 372, 2137 (2008)
Z. Kutnjak, J. Petzelt, R. Blinc, Nature (London) 441, 956 (2006)
R.E. Cohen, Nature (London) 441, 941 (2006)
D. Damjanovic, Rep. Prog. Phys. 61, 1267 (1998)
M.M. Gomaa, H.A. Abo-Mosallam, H. Darwish, J. Mater. Sci. Mater. Electron. 20, 507 (2009)
Z. Jiwei, Y. Xi, C. Xiaogang, Z. Liangying, H. Chen, J. Mater. Sci. 37, 3739 (2002)
H.S. Maiti, R.N. Basu, Mater. Res. Bull. 21, 1107 (1986)
S.H. Yoon, C.A. Randall, K.H. Hur, J. Appl. Phys. 107, 103721 (2010)
H. Beltran, E. Cordoncillo, P. Escribano, D.C. Sinclair, A.R. West, J. Appl. Phys. 98, 094102 (2005)
D.C. Sinclair, A.R. West, J. Appl. Phys. 66, 3850 (1989)
C.R. Gautam, A.K. Yadav, P. Singh, Mater. Res. Innov. 17, 148 (2013)
A.K. Yadav, C.R. Gautam, A. Gautam, V.K. Mishra, Phase Transit. 86, 1000 (2013)
A.K. Yadav, C.R. Gautam, Adv. Appl. Ceram. 113, 193 (2014)
S. Singh, S.P. Singh, D. Pandey, J. Appl. Phys. 103, 016107 (2008)
C. Menoret, J.M. Kiat, B. Dkhil, M. Dunlop, H. Dammak, O. Hernandez, Phy. Rev. B 65, 224104 (2002)
T. Badapanda, S.K. Rout, S. Panigrahi, T.P. Sinha, Bull. Mater. Sci. 31, 897 (2008)
Y. Guo, K. Kakimoto, H. Ohsato, Solid State Commun. 129, 274 (2004)
E. Courtens, Phys. Rev. Lett. 52, 69 (1984)
Y. Zhang, T. Ma, X. Wang, Z. Yuan, Q. Zhang, J. Appl. Phys. 109, 084115 (2011)
D. Kumar, C.R. Gautam, O. Parkash, Appl. Phys. Lett. 89, 112908 (2006)
F.D. Morrison, D.C. Sinclair, A.R. West, J. Appl. Phys. 86, 6355 (1999)
C.R. Gautam, P. Singh, O.P. Thakur, D. Kumar, O. Parkash, J. Mater. Sci. 47, 6652 (2012)
Acknowledgments
The authors are gratefully acknowledged to the University Grant Commission (UGC), New Delhi, (India) for financial support under Major Research Project F. No. 37-439/2009 (SR).
Author information
Authors and Affiliations
Corresponding author
Rights and permissions
About this article
Cite this article
Yadav, A.K., Gautam, C.R. Successive relaxor ferroelectric behavior in La modified (Ba,Sr)TiO3 borosilicate glass ceramics. J Mater Sci: Mater Electron 25, 3532–3536 (2014). https://doi.org/10.1007/s10854-014-2051-7
Received:
Accepted:
Published:
Issue Date:
DOI: https://doi.org/10.1007/s10854-014-2051-7