Abstract
Lead-free (1-x) [0.934BNT-0.07KNN]-x SrTiO3/BNT-KNN-ST ceramics with x = 0, 0.04, 0.08, 0.12 and 0.16 were synthesized in single perovskite phase by conventional solid state reaction route. Effect of SrTiO3 modification on phase, microstructure, dielectric, electric field induced polarization, electric field induced strain and energy-storage properties were investigated and discussed in detail. Dielectric study confirmed relaxor nature with a drastic decrease of T c with the increase of SrTiO3 content in BNT-KNN-ST system. Saturation polarization, remnant polarization, coercive field (E c ) and maximum induced strain decreased with the increase of SrTiO3 content in BNT-KNN-ST system. High recoverable energy storage density of ~0.59 J/cm 3 with energy storage efficiency of ~64% were obtained in x = 0.16 ceramic samples, which suggested its usefulness for energy-storage capacitor applications.
Similar content being viewed by others
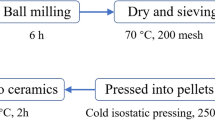
Avoid common mistakes on your manuscript.
1 Introduction
Recently, due to environmental concerns, green energy storage materials and devices are attracting the attention of research community. In comparison to Lithium based batteries, high dielectric constant (ε r ) and comparatively fast discharging speed of ferroelectric (FE) materials make them suitable for high energy storage density applications [1]. Energy storage density (W 1 ) of a FE material can be calculated from its polarization vs. electric field (P-E) hysteresis loop by using the following relation:
where, E is the applied electric field and P is the polarization. Energy storage efficiency (η) of a FE material is calculated by using the following relation:
where, W 1 is the stored energy density; W 2 is the energy loss density [2, 3]. Equations 1 & 2 suggest that FE materials with slim P-E loops, high saturated polarizations (P s ) and low remnant polarizations (P r ) would exhibit high energy storage density. There are two ways to achieve slim P-E loop, high P s and low P r in a FE material. These two methods are: (i) to modify FE material, having large energy storage density, with a suitable antiferroelectric (AFE) material [4] and (ii) to search for an effective relaxor FE material [5]. Liu et al. [6, 7] studied the temperature dependence of dielectric and energy storage properties of lead based antiferroelectric (AFE) ceramics. Due to environmental concerns of lead based materials, there is a need to develop lead free ceramics for energy storage applications. Among the potential lead free FE materials for high energy storage density applications, Bi0.5Na0.5TiO3 (BNT)-based complex FE materials are the promising materials. BNT has a rhombohedral perovskite structure with R3C symmetry. In the BNT-based system, A-site in the perovskite unit cell is shared by mixed-valency ions like: Bi3+, Na+, K+, Sr2+ etc. Local interactions among A-site ions in BNT based systems can give rise to multiple phase transitions [8]. Bi0.5Na0.5TiO3 – K0.5Na0.5NbO3 (BNT-KNN) is one of the BNT-based system having mixed-valency ions at A-site. It is a known fact that a FE system exhibit optimum performance near its morphotropic phase boundary (MPB) compositions. Kounga et al. [9] and Chandrasekhar et al. [10] reported the existence of a MPB with rhombohedral and tetragonal phases in the 0.93BNT-0.07KNN composition. This MPB composition exhibits good FE property and large electric field induced strain. Among the RT non-polar materials, SrTiO3 (ST) is an important perovskite cubic system. Good breakdown strength with linear dielectricity of ST system accounts for its low energy dissipation and suitability for energy storage applications [11].
Therefore, in the present work, for obtaining slim P-E loops, high P s and low P r , 0.93BNT-0.07KNN composition is modified with ST system. Phase formation, dielectric, FE and energy-storage properties of the ST modified BNT-KNN ceramics were studied and discussed in detail.
2 Experimental details
Conventional solid-state reaction route was followed to synthesize (1-x) [0.93BNT-0.07KNN]-x SrTiO3 (BNT-KNN-ST) ceramic samples with x = 0, 0.04, 0.08, 0.12 and 0.16. Stoichiometric weights of Bi2O3, Na2CO3, TiO2, SrCO3, Nb2O5 and K2CO3 (all are 99.9% purity) precursors were ball milled for 20 h (h) by using zirconia balls with acetone as grinding media followed by calcination at 850 °C for 4 h. Single perovskite phase formation in calcined powders was confirmed by X-ray diffraction (XRD) (Rigaku Ultima IV X-ray diffractometer, Tokyo, Japan) technique. Green pellets of ~10 mm in diameter and ~1.5 mm in thickness were compacted using a uniaxial hydraulic press with 6 tons/cm 2 pressure for 3 min. These green pellets were sintered in air atmosphere at 1100 °C for 4 h. Retention of single perovskite phase in sintered ceramic samples was confirmed by XRD study. Surface microstructures of the pellets (as sintered) were observed using Field emission scanning electron microscope (NOVA nano SEM). Polished sintered pellets were coated with silver paste and fired at 400 °C for 30 min for good adhesion. Dielectric constant (ε r ) and dielectric loss (tanδ) were measured as a function of temperature using a computer interfaced HIOKI 3532–50 LCR-HITESTER. Polarizations vs. electric field (P-E) hysteresis loops at room temperature were measured using Radiant precision premier II. Strain vs. electric field (S-E) hysteresis loops were measured using MTI-2100 Fotonic sensor, attached with Radiant precision premier II unit.
3 Results and discussion
3.1 XRD and microstructure study
Figure 1 shows the XRD patterns of sintered BNT-KNN-ST ceramic samples. Single perovskite phase is observed in all the compositions. Fig. 1(b), (c) show the enlarged pictures of (111) and (200) XRD peaks. Splitting of (111) and (200) XRD peaks of x = 0 ceramic samples suggests its MPB nature. In x = 0 ceramic samples, rhombohedral (R) and tetragonal (T) phases with lattice parameters: (aR, αR) ~ (5.4974 Å, 90.08°) and (aT, cT) ~ (5.4998 Å, 13.7274 Å), respectively [9, 10] were obtained. In other than x = 0 ceramic samples, XRD peak splitting corresponding to (111) and (200) planes is not observed, which suggests cubic or pseudo cubic structures of these samples [12]. In x = 0.04, 0.08, 0.12 and 0.16 ceramic samples, cubic lattice parameters ~3.8608 Å, 3.8638 Å, 3.8641 Å and 3.8686 Å, respectively were obtained. With the increase of ST content, XRD peaks shift towards higher angle side, shown in Fig. 1(b). This suggests the decrease of lattice parameters with the increase of ST content [12].
Figure 2 shows the FESEM micrographs of sintered BNT-KNN-ST ceramic samples. Average grain size of BNT-KNN-ST ceramic samples, determined by using linear intercept method, are given in Table 1. With the increase of ST content from x = 0 to 0.08, average grain size increases and above x = 0.08, average grain size starts decreasing. It is observed from microstructure that tightly bounded sharp cornered cubic like grains are present in x = 0 ceramic samples and porosity increases with the increase of ST content in BNT-KNN-ST ceramic samples. Variation of grain size can be explained on the basis of complex defects theory. Complex defects as well as single-ion vacancies can be formed due to the presence of aliovalency at A-site ions and covalency of A-O bond in BNT-KNN-ST ceramic samples. During sintering process, mass transfer increases with the increase of diffusion of single-ion-defects, which can promote the grain growth. This suggests that upto x = 0.08, diffusion of single-ion defects process dominates and diffusion process of complex defects starts dominating in x = 0.12 and 0.16 ceramic samples [13].
3.2 Dielectric properties:
Fig. 3 shows temperature dependence of ε r and tanδ at different frequencies of sintered BNT-KNN-ST ceramic samples. Dielectric anomaly, observed near depolarization temperature (T d ), corresponds to FE to intermediate phase transition. FE-AFE phases coexist beyond T d . Whereas, dielectric anomaly, observed near maximum dielectric constant temperature (T m or T c ) corresponds to paraelectric phase transition [2, 14]. Up to T c /T m , value of ε r at different frequencies increases with the increase of temperature which can be related to the increase in polarization due to increased easy orientation of dipoles. Above T c /T m , ε r decreases due to increase of thermal oscillation of molecules and increase of degree of disorderness of dipoles. Dielectric parameters such as ε r , ε m, T d and T m are given in Table 1. With the increase of ST content, T m decreases and dielectric spectra broadens, which is attributed to the increased isovalency of A-site and increased covalency of A–O bond in the BNT-KNN-ST system. This increased isovalency can be accounted in terms of partial replacement of (Bi3+/Na+/K+) ions by Sr2+ ions at A-site. Increased covalency of A–O bonds can be accounted in terms of fractional replacement of Na–O or K–O bonds by Sr–O bonds, since they are ionic in nature, while Bi–O bonds are covalent in nature. Increased dielectric dispersion with the increase of ST content can be explained in terms of increased heterogeneity at A-site. This heterogeneity can perturb the Coulombic interaction at long range and can ultimately lead to the formation of polar nano-domains. Moreover, increased heterogeneity at A-site reduces the degree of coupling between permanent dipoles, which results in destruction of FE domains with short-range cation ordering and can lead to relaxor behaviour [15]. At high temperature, dielectric loss at lower frequencies increases drastically, which can be accounted by the increased electrical conductivity of space charges [16]. RT dielectric constant increases with the increase of ST content, which may be due to the increase of space charge effect as well as compositional inhomogeneity [17]. Decrement in T d with the increase of ST content is caused by increased AFE ordering in the BNT-KNN-ST system [18].
Curie–Weiss temperature (T CW ) of BNT-KNN-ST ceramic samples is calculated from linearly fitted graph between 1/ε r vs. T for T > T m . Burn’s temperature (above T c ) T B , below which 1/ε r is a non-linear function of T is determined from 1/ε r vs. T graph, shown in Fig. 4. T B values ~302, 295, 294, 267 and 260 °C were obtained for x = 0, 0.04, 0.08, 0.12 and 0.16 ceramic samples, respectively. T B represents cross-line between soft mode and order–disorder dynamics. Therefore, when T < T B , polar nano-regions appear, and the displacive-type soft mode is over damped [19] and Curie–Weiss law becomes invalid within the T m –T B temperature range. ΔT m = T m -T B , estimates the deviation from classic Curie–Weiss behaviour of a system. Non-zero values of ΔT m suggest the relaxation near phase transition and broadening in the ε r vs. T plots [19, 20].
Diffuseness of BNT-KNN-ST ceramic samples is studied by plotting dielectric vs. temperature behaviour near T c of the modified Curie–Weiss law [1, 21], given below:
where γ and C are constants. Normally, γ value is between 1 and 2 and gives information about the nature of phase transition. γ values are 1 and 2 for normal FE and ideal relaxor FE materials, respectively [22]. Figure 5 shows the log (1/ε r – 1/ ε m ) vs. log (T − T m ) plots of the BNT-KNN-ST ceramic samples. Slopes of the linearly fitted lines in log (1/ε r – 1/ε m ) vs. log (T − T m ) plots gives γ factor. γ values ~1.94, 1.78, 1.74, 1.89 and 1.97 are observed for x = 0, 0.04, 0.08, 0.12 and 0.16 ceramic samples, respectively. γ values of BNT-KNN-ST ceramic samples are ~2, which confirm their diffusive phase transition nature.
3.3 P-E hysteresis loop and energy storage density study
Figure 6 shows the P-E hysteresis loops, measured at 10 Hz frequency, of BNT-KNN-ST ceramic samples. A well saturated typical P-E hysteresis loop of x = 0 ceramic sample confirms its FE nature. Whereas, with the increase of ST content, a drastic reduction in E c and P r values with relatively less reduction in P s values is observed, which suggests the suppressing of FE order and increase of non-polar order [23].
Variation of W 1 and η% with the increase of ST content in BNT-KNN-ST ceramic samples is shown in Fig. 7. All the measurements for W 1 and η% are done under same external applied electric field (~70 kV/cm). With the increase of ST content, W 1 and η% increases, which can be related to increase of non-polar state and decrease of FE state in BNT-KNN-ST system [9, 24]. Enhancement of W 1 values with the increase of ST content can also be further related to reduction in remnant polarization [25], confirmed by P-E hysteresis loop study. Highest W 1 value of ~0.59 J/cm 3 as well as highest η% value of ~64 was obtained in x = 0.16 ceramic samples, which suggest its usefulness for energy storage applications.
3.4 S-E hysteresis loop study
Figure 8 shows the RT bipolar S-E hysteresis loops, measured at 10 Hz, of BNT-KNN-ST ceramic samples. Butterfly shaped S-E loop of x = 0 ceramic samples suggest its piezoelectric and FE nature. Whereas, decrease of butterfly nature of S-E loops with the increase of ST content suggest the decrease of piezoelectric nature and increase of AFE nature [2, 26]. Asymmetry in butterfly S-E hysteresis loops of x = 0 and 0.04 ceramic samples suggest the presence of internal bias, resulting from volatilization of Bi and Na cations during sintering at high temperature [27–30]. Maximum induced strain % values of ~0.62, 0.18, 0.15, 0.11and 0.09 were obtained in x = 0, 0.04, 0.08, 0.12 and 0.16 ceramic samples, respectively. Drastic decrease of induced strain% with the increase of ST content in BNT-KNN-ST system can be related to replacement of FE ordering with AFE ordering [31].
4 Conclusions
XRD study confirmed rhombohedral-tetragonal MPB nature of x = 0 ceramic samples and pseudo cubic structure nature of other ceramic samples. Variation of average grain size with ST modification in BNT-KNN-ST system was explained on the basis of complex defect theory. Dielectric study and modified Curie–Weiss law revealed relaxor behaviour of ST modified BNT-KNN-ST ceramic samples. Drastic decrease of P r , E c and S% with the increase of ST content suggested the increased AFE nature of BNT-KNN-ST ceramic samples. Highest values of W 1 ~ 0.59 J/cm 3 and η% ~64 of x = 0.16 ceramic samples suggested their usefulness for energy storage applications.
References
S.A. Sherrill, P. Banerjee, G.W. Rubloff, S.B. Lee, Phys. Chem. Chem. Phys. 13, 20714 (2011)
M. Chandrasekhar, P. Kumar, Ceram. Int. 41, 5574 (2015)
V.S. Puli, D.K. Pradhan, B.C. Riggs, D.B. Chrisey, R.S. Katiyar, J Alloys Comp 584, 369 (2014)
X. Hao, J Adv Dielectr 3(1), 1330001 (2013)
B. Hitesh, V.N. Singh, B.P. Singh, M. Tomar, V. Gupta, A. Kumar, RSC Adv. 4, 22840 (2014)
Z. Liu, X. Chen, W. Peng, C. Xu, X. Dong, F. Cao, G. Wang, Appl. Phys. Lett. 106, 262901 (2015)
Z. Liu, B. Yang, X. Chen, X. Dong, H. Nie, F. Cao, G. Wang, J Alloys Comp. 691, 721 (2017)
D. Xiao, D. Lin, J. Zhu, P. Yu, J. Electroceram. 16, 271 (2006)
A.B. Kounga, S.-T. Zhang, W. Jo, T. Granzow, J. Rödel, Appl. Phys. Lett 92, 222902 (2008)
M. Chandrasekhar, P. Kumar, Process Appl Ceram 10(2), 73 (2016)
G. Zhao, Y. Li, H. Liu, J. Xu, H. Hao, M. Cao, Z. Yu, J Ceram Process Res 13(3), 310 (2012)
F. Wang, C. Jin, Q. Yao, W. Shi, J. Appl. Phys. 114, 027004 (2013)
L. Zhang, S. Jiang, Y. Zeng, F. Ming, K. Han, Q. Li, Q. Wang, G. Zhang, Ceram. Int. 40, 5455 (2014)
W. Jo, S. Schaab, E. Sapper, L.A. Schmitt, H.J. Kleebe, A.J. Bell, et al., J. Appl. Phys. 110, 074106 (2011)
S.A. Shahin Sayyed, P.K. Acharya, V. Sa, RSC Adv. 5, 50644 (2015)
C. Ma, X. Tan, E. Dul’kin, M. Roth, J. Appl. Phys. 108, 104105 (2010)
V. Pal, R.K. Dwivedi, O.P. Thakur, Mater. Res. Bull 51, 189 (2014)
R. Zuo, C. Ye, X. Fang, J. Li, J. Eur. Ceram. Soc. 28, 871 (2008)
K. Chu, Y. Shi, K. Li, B. Fang, J. Ding, J. Phys. Chem. Solids 81, 10 (2015)
K. Datta, P. Thomas, K. Roleder, Phys. Rev. B: Condens. Matter Mater. Phys 82, 224105 (2010)
R.A. Mondal, B.S. Murty, V.R.K. Murthy, Physica B 461, 10 (2015)
C.-C. Wu, C.-F. Yang, Cryst. Eng. Comm. 15, 9097 (2013)
M. Chandrasekhar, Sonia, P. Kumar, Physica B 497(59) (2016)
M. Chandrasekhar, P. Kumar, Phase Transitions 89(9), 944 (2016)
K. Yao et al., IEEE Trans. Ultrason., Ferroelect., Freq. Control 58(9), 1968 (2011)
T.Y. Ansell, D.P. Cann, Mater. Lett 80, 87 (2012)
X. Liu, X. Tan, Adv. Mater. 28, 574 (2016)
L. Jin, F. Li, S. Zhang, J. Am. Ceram. Soc. 97, 1 (2014)
Q. Zhang, X. Zhao, R. Sun, H. Luo, Phys. Status Solidi A 208, 1012 (2011)
L. Liu, D. Shi, M. Knapp, H. Ehrenberg, L. Fang, J. Chen, J. App. Phys. 116, 184104 (2014)
Y. Li, W. Cao, Q. Li, Q. Yan, J. Gao, F. Zhuo, X. Xi, Y. Zhang, X. Chu, Appl. Phys. Lett. 104, 052912 (2014)
Author information
Authors and Affiliations
Corresponding author
Rights and permissions
About this article
Cite this article
Chandrasekhar, M., Kumar, P. Synthesis and characterizations of SrTiO3 modified BNT-KNN ceramics for energy storage applications. J Electroceram 38, 111–118 (2017). https://doi.org/10.1007/s10832-017-0074-1
Received:
Accepted:
Published:
Issue Date:
DOI: https://doi.org/10.1007/s10832-017-0074-1